Chapter 3 - Radiation Protection PDF
Document Details
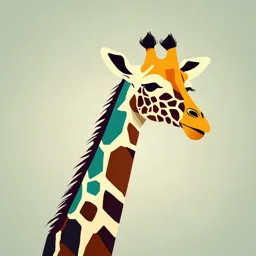
Uploaded by IdyllicUranus1947
Washington Adventist University
Tags
Summary
This document discusses interaction of X-radiation with matter. It details fundamental physics concepts, processes of interaction between radiation and matter, and technique factors like peak kilovoltage (kVp) and milliampere-seconds (mAs). It also explains the significance of x-ray absorption in biological tissue.
Full Transcript
"1 CHAPTER 3 Interaction of X-Radiation With Matter 35 photoelectric absorption primary radiation radiographic fog photoelectron radiographic contrast...
"1 CHAPTER 3 Interaction of X-Radiation With Matter 35 photoelectric absorption primary radiation radiographic fog photoelectron radiographic contrast small-angle scatter Fundamental physics concepts that relate to radiation If an interaction occurs, electromagnetic energy is absorption and scatter are reviewed in this chapter. The transferred from the x-rays to the atoms of the patient's processes of interaction betwe~n radiation a~d matter biologic tissue. This process is called absorption are emphasized because a basic understandmg of the (Fig. 3.1 ), and the amount of energy absorbed per unit subject is necessary for radiographers to optimally select mass is referred to as the absorbed dose (D). The more the following technical exposure factors: electromagnetic energy that is received by the atoms. peak k.ilovoltage (kVp), the highest energy level of the patient's body, the greater is the possibility of of photons in the x-ray beam, equal to the highest biologic damage in the patient. Therefore, the amount voltage established across the x-ray tube of electromagnetic energy transferred should be kept milliampere-seconds (mAs), the product of electron tube current and the amount of time in seconds that the x-ray tube is activated Peak kilovoltage controls the quality, or penetrat- ing power, of the photons in the x-ray beam and to some degree also affects the quantity, or number of photons, in the beam. The product of milliamperes (mA), which is the x-ray tube current, and time (sec- X-ray photons onds [s] during which the x-ray tube is activated) is the main determinant of how much radiation is directed toward a patient during a selected x-ray exposure. Because the level of energy (beam quality) and the number of x-ray photons are controlled by technique factors selected by the radiographer, the radiographer is responsible for the radiation dose the patient receives during an imaging procedure. With a suitable understanding of these factors, radiographers will be able to select appropriate techniques that can minimize that dose to the patient while producing optimal-quality images. SIGNIFICANCE OF X-RAY ABSORPTION IN BIOLOGIC TISSUE X-rays are carriers of human-made electromagnetic energy. If x-rays enter a material such as biologic tissue, they may: 1. Interact with the atoms of the biologic material in " - Image receptor the patient and be absorbed Fig. 3.1 X-ray photons can interact w ith atoms of the patient's 2. Interact with the atoms in the biologic material and body and transfer energy to the tissue. This transference of · be scattered, causing some indirect transmission electromagnetic energy to the atoms of the material is called 3. Pass through without interaction absorption. CHAPTER 3 Interaction of X-Radiation With Matte r as small as possible. However, without absorption and Target the differences in the absorption properties of various (anode +) body structures, it would not be possible to produce diagnostically useful images, that is, images in which different anatomic structures could be perceived and distinguished. Thus, some small amount of radiation dose is necessary. The radiographer also benefits when the patient's dose is minimal because less radiation is scattered from the patient. Filament X-RAY BEAM PRODUCTION AND ENERGY window (cathode-) Production of Primary Radiation A diagnostic x-ray beam is produced when a stream of very energetic electrons bombards a positively charged target in a highly evacuated glass tube. In general radi- ography, this target, also known as the anode, is usually made of tungsten or an alloy of tungsten and rhenium. ~ Primary radiation These materials have: Fig. 3.2 Primary radiation emerg es from the x-ray tube target High melting points and consists of x-ray photons of various energies. It is pro- High atomic numbers (tungsten and rhenium ) duced when the positively charged target is bombarded with a As the electrons interact with the atoms of the tube stream of high-speed electro ns and these electrons interact target, x-ray photons are produced. Photons are parti- w ith the atoms of the target. cles associated with electromagnetic radiation that have neither mass nor electric charge and travel at the speed bombard the target. The energy of the electrons inside of light. X-ray photons exit from the tube target with the x-ray tube is generally specified in terms of the a broad range, or spectrum, of energies and leave the electrical voltage applied across the tube. In diagnostic x-ray tube through a glass window. The glass window radiology, this voltage is expressed in thousands of volts, permits passage of all but the lowest-energy compo- or kilovolts (kV). Because the voltage across the tube nents of the x-ray spectrum. It therefore acts as a filter is not fully constant but fluctuates a bit, it is usually by removing diagnostically useless, very-low-energy characterized by the kilovolt peak value (kVp). x-rays. In addition to this, a certain thickness of added If an electron is drawn across an electrical potential aluminum is placed within the collimator assembly difference of 1 volt, it has acquired an energy of 1 elec- to intercept the emerging x-rays before they reach tron volt (eV). Therefore a technique factor setting of the patient. This aluminum "hardens" the x-ray beam 100 kVp (i.e., establishing a peak potential difference of (i.e., raises its effective energy) by removing low-energy 100,000 volts between cathode and anode of the x-ray components that would serve only to increase patient tube) means that the electrons bombarding the tube dose. The combination of the x-ray tube glass wall and target have acquired a maximum energy of 100,000 eV, the added aluminum placed within the collimator is or 100 keV. X-rays of various energies, comprising a called the permanent inherent filtration of the x-ray unit. spectrum, are produced, but the most energetic x-raY This filtered x-ray photon beam is coUectively referred photon can have no more energy than 100 keV. For a to as primary radiation (Fig. 3.2). typical diagnostic x-ray unit, the mean photon energy Energy of Photons in a Diagnostic X-Ray in the x-ray beam is about one-third the energy of the most energetic photon. Therefore a 100-kVp beam con· Beam tains photons having energies of 100 keVor less, with an Although all photons in a diagnostic x-ray beam do not average or effective energy of approximately 33 keV. In have the same energy, the most energetic photons in the summary, the units kVp or kV refer to the voltage on the beam can have no more energy than the electrons that x-ray tube, and keV refers to the energy of specific x-ra)'S C CHAPTER 3 Interaction of X-Radiation With Matter ATTENUATION always the result of scatter. However, all scatter does not result in indirect transmission. The goal of radio- Direct and Indirect Transmission X-Ray graphic imaging is to use the directly transmitted Photons photons to construct the final image and to eliminate In radiography, x-rays travel from an x-ray tube to an as much indirect transmission as possible. The latter image receptor, a type of digital device that can convert detracts from the sharpness or quality of the image. the spatial pattern of the photons that emerge from the Unfortunately, indirect transmission is always pres- patient (the patient's x-ray "shadow") into electrical ent. A number of techniques, however, have been signal values that can be stored in a computer and dis- devised to substantially reduce the influence of indi- played as an image. A number of terms are used to refer rect transmission or scattered photons upon the final to the behavior of x-ray photons as they interact or else image simply pass through the patient. The atomic processes that produce the behaviors of the x-ray photons will be Primary, Exit, and Attenuated Photons discussed later in this chapter. Fig. 3.3 illustrates the passage of four x-ray photons through a patient. Before the four photons produced Absorption vs. Scatter. X-rays sometimes interact by the x-ray source enter human tissue, they are re- with atoms of a patient such that they give up all of their ferred to as primary photons. Only two photons emerge energy and cease to exist. These photons are said to from the tissue and strike the x-ray detector below it. be absorbed. Other photons interact with atoms of the They are referred to as exit, or image-formation, patient, but only surrender part of their energy. They photons. The two that miss the detector are classified will continue to exist but will emerge from the interac- as attenuated. The term attenuation is rather broad. tion at a different angle (somewhat like a billiard ball With respect to x-rays, attenuation may be used to colliding with another billiard ball). These photons are refer to any process decreasing the intensity of the said to be scattered. primary photon beam (i.e., the number of photons crossing unit area per second) directed toward a par- Attenuation vs. Transmission. Photons that strike the ticular destination. In Fig. 3.3 that end point is the image receptor are called transmitted photons. These are image receptor. Therefore, photon 3, which has devi- photons that either did not interact with the atoms of ated from its path (i.e., it has been "scattered") to the the patient or else interacted through scatter but still extent that it will not strike the detector is said to have struck the receptor, although at a different spot than been attenuated. Photon 4 seems to disappear. It has they would have struck the image receptor if they had transferred all its energy to the atoms of the patient not interacted. Attenuated photons are the photons and has therefore been eliminated. This occurs because that have undergone either absorption or scatter and a photon has no mass, only energy, which implies that do not strike the image receptor. This terminology can it ceases to exist when it gives up its energy. Attenua- be connected to absorption and scatter as follows: If a tion, then, refers to both absorption and scatter pro- person were counting photons that were emitted from cesses that prevent photons from reaching a predefined the x-ray tube, the total number of photons attenuated location. Fig. 3.3 shows that the path of photon 2 was would equal the total number of photons absorbed plus bent, but not so much that the photon missed jts tar- the total number that scattered away from the image get. Since photon 2 reaches the image receptor (IR), it receptor. is part of the exit, or image-formation, radiation, but the bending of its path represents what is called small- Direct Transmission vs. Indirect Transmission. If angle scatter. Scattered photons in this category have photons pass through the patient without interacting essentially the same energy as the incoming, or "inci- with the atoms of the patient, they are referred to as dent," photons. As mentioned previously, sn1all-angle direct transmission photons. If they interacted but still scatter degrades the appearance of a completed radio- happened to strike the image receptor, they are graphic image by blurring the sharp outlines of dense termed indirect transmission photons. To connect to structures. Because many billions of such scatter events the previous terminology, indirect transmission is happen, a greater overall exposure of the IR occurs CHAPTER 3 Interaction of X-Radiation With Matter / PROBABILITY OF PHOTON INTERACTION WITH MATTER l3ecause the interaction of photons with biologic tnatt is random, it is impossible to predict with certain:r what will happen to any single photon when it ente y human tissue. When dli ea ng wit' h a very large nurnbers of photons, h owever, it is possible to predict what wi~ happen on the average, and this is more than adequat to determine the characteristics of the image that result: from these numerous interactions. Table 3.1 provide.s a short description of the characteristics of each of the various types of radiation interactions with tissue. Table 3.2 shows the factors that influence the proba- Small-angle bility of interactions in m atter. In the remainder of scatter ~ this chapter, the different interactions of photons with No h Scattered individual atoms and the effect of a particular type photon of interaction on the radiographic image are examined. PROCESSES OF INTERACTION Five types of interactions between x-radiation and matter are possible: \ Image receptor Exit, or image- l. Coherent scattering formation, 2. Photoelectric ab sorption radiation 3. Compton scattering Primary - Exit = Attenuation 4. Pair production 5. Photodisintegration Fig. 3.3 Primary, exit, and attenuated photons. Primary pho- tons (photons 1, 2, 3, and 4) are photons that emerge from the Of these, only two are important in diagnostic radiology: x-ray source. Exit, or image-formation, photons (photons 1 and l. Compton scattering 2) are photons that pass through the patient being radio- 2. Photoelectric absorption graphed and reach the radiographic image receptor. Attenuated Box 3.l presents an overview of the various interac- photons (photons 3 and 4) are photons that have interacted w ith atoms of the patient's biologic tissue and been scattered tions between x-radiation with matter and where they or absorbed such that they do not reach the radiographic image are important. receptor. Coherent Scattering Coherent scattering is sometimes also called by the following names: than is needed. This additional, undesirable exposure Classical scattering is commonly called tadiographic fog. It interferes Elastic scattering with the radiologist's ability to distinguish different Unmodified scattering structures in the image. Reducing the amount of tissue It is a simple process that results in no loss of energy irradiated decreases the amount of fog produced and as x-rays scatter. is therefore another method of limiting the number of indirectly transmitted photons. The radiographer can Process of Coherent Scattering. When a low-energy achieve this by confining, or collimating, the x-ray (typically less than 10 keV) photon interacts with an beam as much as possible to include only the region of atom, it may transfer its energy by causing some or all interest (Fig. 3.4). of the electrons of the atom to momentarily -vibrate. CHAPTER 3 Interaction of X-Radiation With Matter Left clavicle :r-- --=-~1111111 Jugular notch L ,---,..~- Sternoclavicular""f_ _-=':;.,...,. joint [ --- 1st Rib Lr--,-~-.-=, -:-:::=-:;:::;-::;-~ Manub,;um !' Sternal angle =[-= =----,----~ Body r Xiphold process j ~...... ---~~-............. -'---~ Fig. 3.4 (A) Lateral image of the lumbar vertebrae showing improper collimation, which results in the production of -~ radiographic fog and a consequent lack of radiographic clarity. (B) Lateral image of the lumbar vertebrae showing adequate collimation, which eliminates radiographic fog and consequently increases radiographic clarity. (C) PA oblique projection (right anterior oblique (RAO! position) of the sternum, demonstrating poor collimation. (D) PA oblique projection (RAO position) of the sternum, demonstrating good collimation. (D, From Long BW, Rollins JH, Smith BS: Merrill's Atlas of radiographic positioning and procedures, ed 13, St. Louis, 2016, Elsevier.) CHAPTER 3 Interacti on of X-Radiat ion With Matter Site Typical By-Products X -Ray Photon n X -Ray Photon Interactio n of Interaction Energy Range !::!!.::!.lil.. of Interactio.!!!:!~!:_- ~~~~~!!...._..!~:: :L.;..:.:: :..:.=.:..: -------..;. ;.;_~~;;. ;;_;-----. ;__;..;..;.;;.;;.:,.: ;~:::!!.-- --- An atom Energy: unchanged; direction Coherent scattering * None 1-50 kVp after interaction : slight change (< 20 degrees) Energy: absorbed ; direction Photoelec tric Photoelectron 1-50 kVp Inner-shell after interaction : not absorption t (character istic electron (usually K or applicable photon) L shell) Outer-shell Energy: reduced; direction Compton scattering * Compton scattered 60-90 kVp electron after interaction : changed Photoelec tric electron (x-ray photon energy partially absorptio nt Compton scattered absorbed) photon Outer-shell Energy: reduced after interac- Compton * Compton scattered 90-120 kVp t ion: changed (x-ray photon scattering electron electron energy partially absorbed) Photoelectric Compton scattered absorption t photon Energy: reduced; direction Compton scattering §ll Compton scattered 200 kVp-2 MeV Outer-shell after interaction : changed electron elect ron Compton scattered photon Energy: disappears after Pair product ion Positive electron Begins at about Nucleus of interact ion w ith nucleus; (positron); ordinary 1.022 MeV; atom transform ed into two new electron (negatron); becomes particles that annihilate each two 0.511-MeV important at other; at photon energy photons a 1.022 10 MeV; 1.022 MeV. after interaction : MeV initial energy becomes predomin ant energy reappea rs in the form at 50 MeV of two 0.511-MeV photons and greater moving in opposite directions Greater than Nucleus of Energy: absorbed by nucleus Photodisi ntegration Neutron; other types 10 MeV atom after collision with high- of emissions possi- energy photon; excess ble if sufficient en- energy in nucleus creates ergy is absorbed by instability that is usually the nucleus: proton alleviated by emission of a or proton-neu tron neutron; other emissions combination (deu- possible teron) or even an alpha particle tric absorption. *This scattering occurs mostly in this energy range, but it is still much less probable than photoelec tThe interaction most responsible for radiation dose in this energy range. *Both Compton and photoelectric interactions occur in this energy range. §Compton interaction is predominantly responsible for radiation dose in this energy range. tric interactions on their a-Nfl. llln this energy range, the scattered particles go on to produce many more Compton and photoelec This is analogous to the behavior of electrons in the one another, as multiple water waves would similarlJ antenna of a receiver intercepting a radio signal. merge, to form a resultant scattered wave, or photo Because they are charged particles, each of the atom's Because the wavelengths of both incident and sea now vibrating electrons radiates energy in the form waves are the same, no net energy has been absor of electromagnetic waves. These waves combine with by the atom (see Appendix E). However, a small ch CHAPTER 3 Interaction of X-Radiation With Matter TABLE 3.2 Factors That Influence the Probability of Interaction of Photons With Energy E in Materials With Density p Photon Energy Atomic Number Electron Density Physical Density Interaction Deeendence Dependence ~ (e/9) e. (~/cm3 ) Photoelectric 1/E3 Z3 Independent p Compton 1/E Independent Pe p Pair production E z Independent p B_- l!f". In,._ _ lnter0ction m:portance of Various ,:~f 'X-Radiation With Matter Where Important Coherent scattering Not significant in any energy range Scattered photon Photoelectric absorption Diagnostic radiology possessing the Compton scattering same wavelength Diagnostic radiology and and energy, or therapeutic radiology penetrating power, Pair production Therapeutic radiology as that of the Photodisintegration Therapeutic radiology incident x-ray photon traveling in a small angle of scatter in direction for the emitted photon is very likely. In One wavelength (distance between general, this change in direction is less than 20 degrees two crests) with respect to the initial direction of the original Fig. 3.5 Coherent scattering. The incoming low-energy x-ray photon. This is the net effect of coherent, or unmodi- photon interact s with an atom and transfers its energy by fied, scattering. Although coherent scattering is most causing some or all of the electrons of the atom to momen- like]y to occur at less than 10 keV (energies that will be tarily vibrate. The electrons then radiate energy in the form of eliminated by the inherent filtration), some of this electromagnetic waves.These waves nondestructively combine with one another to form a scattered wave, which represents unmodified scattering occurs throughout the diagnos- the scattered photon. Its wavelength and energy, or penetrating tic energy range and can result in small amounts of power, are the same as those of the incident photon. Generally, the radiographic fog (Fig. 3.5). This source of fog, however, emitted photon may change in direction less than 20 degrees is not significant in general diagnostic imaging. In w ith respect to the direction of the original photon. (Wavelength is the distance from one crest to the next.) breast imaging or mammography, which of necessity involves many low-energy photons and therefore uti- lizes much less inherent filtration, coherent scattering far more likely to be scattered and to scatter over a also does not contribute noticeably to radiographic greater ang1e. Therefore when people look up in the sky fog because during this imaging procedure, breast tissue in any direction, they tend to see the blue light scattered is gently but firmly compressed. As a result of this com- toward them. At sunset and at sunrise, as a person looks pression, the breast becomes relatively much thinner. toward the sun, the blue light is mainly scattered away This eliminates the production of a large amount of from them, so the sun appears to consist of mostly scatter radiation. longer wavelength visible light, that is, mostly red. Small angle coherent scattering does not have much A summary of facts about the process of coherent of an effect on radiographic imaging, but it does have a scattering is presented in Box 3.2 for quick reference. ' noticeable effect on visible light. This is why the sky is blue and sunsets are red. 1 Photons of much longer Photoelectric Absorption wavelengths than those of x-rays, especially "visible" Within the energy range of diagnostic radiology wavelengths such as those belonging to blue light, are (23 to 150 kVp), which also includes mammography, ◄ CHAPT ER 3 Interact ion of X-Radia tion With Matter The process of coheren t scattering with regard to patient absorbe d dose is of no importan ce in any energy range. When the low-energy x-ray photon inter- Inner-shell acts with an atom of human tissue, it does not lose Incoming - electron energy. The emitted photon merely changes direction x-ray phot by 20 degrees or less. No ionization of the biologic atom therefor e occurs. A Negatively charged photoelectron photoel ectric absorption is the m ost importa nt mode of interact ion between x-ray photo ns and the atoms " of the patient' s body for produci ng useful images. Process of Photoelectric Absorption. Photoel ectric absorption is an interaction between an x-ray photon and an inner-shell electron ( usually in the K or L shells B Ko Ato shells : :::::~_:-......... vacancy I 1 shell [Fig. 3.6, Table 3.3; and for a detailed explana tion see Append ix F] ). To dislodge an inner-sh ell electron from e - its atomic orbit, the incoming x-ray photon must be able to transfer a quantity of energy as large as or larger than the amount of energy that holds the electron in e yo er- its orbit. On , interact ing with an inner-shell electron, shell electrons- the x-ray photon surrend ers all its energy to the orbital Atomic M K causing radiation electron and ceases to exist. The electron escapes shells (the emission from its inner shell, thus creating a vacancy. The now of characteristic photons) or an unboun d orbital electron, called a photoel ectron, ejected electron possesses energy equal to the energy of the incident C (alternative effect) photon minus the binding energy of its electron shell. This photoelectron may interact with other atoms in Fig. 3.6 Photoelectric absorption. (A) On encountering."" the vicinity, thereby causing excitation (promo tion of inner-shell electron, usually in the K or L shell, the · electrons from lower energy shells to higher energy x-ray photon surrenders all its energy to the electro_n, ~ shells) or ionizati on (comple te ejection of the electron photon ceases to exist. (B) The atom responds by from an atom), until all of its energy has been spent. electron, called a photoelectron, from its inner shell, th~ The photoelectron is usually absorbe d within a few ing a vacancy in that shell. (Cl To fill the opening, an from an outer shell drops down to the vacated in micrometers of the medium through which it travels. releasing energy in the form of a characteristic phOt~· In the human body, this energy transfer results in fill the new vacancy in the outer shell, another e increased patient absorbed dose and does not contrib - the shell next farthest out drops down and anothe~ ute to the radiographic image. tic photon is emitted and so on until the atom regains As a result of the photoelectric interaction, a vacancy equilibrium. There is also some probability that_ Inst is created in an inner shell of the target atom. For the characteristic photon, an Auger electron will ~e eJ ionized atom, this represents an unstable energy situa- tion. The instability is alleviated by filling the vacancy (farther from the nucleus ) to a more tightlyhJ in the inner shell with an electron from an outer shell, (closer to the nucleus). The amount of en which spontaneously "falls down" into this opening. involved is simply equal to the difference in d).ei To do this, the descending electron must lose energy, or "holding," energies associated with each that is, must pass from a less tightly bound atomic state shell. For a large atom such as an atom of th CHAPTER 3 Interaction of X-Radiation With Matter TABLE 3.3 Electron Shell Occupancies for Some Common Atoms* SHELL N 0 p Atom Symbol Atomic Number K L M Hydrogen H 1 1 Helium He 2 2 Lithium Li 3 2 Carbon C 6 2 4 Oxvgen 0 8 2 6 Sodium Na 11 2 8 1 Aluminum Al 13 2 8 3 Calcium Ca 20 2 8 8 2 Copper Cu 29 2 8 18 1 Molybdenum Mo 42 2 8 18 13 Tungsten w 74 2 8 18 32 12 2 Lead Pe 82 2 8 18 32 18 4 Radon Rn 86 2 8 18 32 18 8 "for a detafled discussion of electron shell structure. please see Appendix F. lead, this energy can be ih the kiloelectron volt range, a radiationless effect. It reduces the total amount of whereas for the small or low atomic number atoms that characteristic radiation produced by photoelectric make up most of the human body, the energy is on interactions. The term fluorescent yield refers to the the order of 10 eV. The "released" energy is carried off number of x-rays emitted per inner-shell vacancy. in the form of a photon that is called a characteristic Because the Auger effect is more prevalent in materials photon, or characteristic x-ray, because its energy is with higher atomic number atoms, the fluorescent yield directly related to the shell structure of the atom from per photoelectron is generally lower in such materials which it was emitted. Those photons generated from than for substances with low atomic numbers (see photoelectric interactions within human tissue are low Fig. 3.6C). enough in energy that they are predominantly absorbed In summary, the by-products of photoelectric withfa the body. In general, ensuing vacancies in other absorption include the following: electron shells are successively filled, and associated l. Photoelectrons (those induced by interaction with characteristic photons are emitted until the atom external radiation and the internally generated Auger achieves an electronic equilibrium. electrons) One additional process can occur as a result of 2. Characteristic x-ray photons (fluorescent radiation) photoelectric interactions. It is called the Auger effect When the energy of these by-products is locally ab- (pronounced "awzhay"), named after the French scien- sorbed in human tissue, both the dose to the patient and tist, Pierre Vjctor Auger, who discovered it in 1925. the potential for biologic damage increase. When an inner electron is removed from an atom in a A summary of facts about the process of photoelectric photoelectric interaction, thus causing an inner-shell absorption is presented in Box 3.3 for qu·ick reference. vacancy, the energy liberated when this vacancy is filled can be transferred to another electron of the atom, Probability of Occurrence of Photoelectric Absorption. thereby ejecting that electron, instead of emerg.i ng from The probability of occurrence of photoelectric absorp- the atom as characteristic radiation. Such an emitted tion per atom within a particular material depends on electron is called an Auger electron. Its energy is equal to the energy (E) of the incident x-ray photons and the the difference between that released by an outer electron atomic number (Z) of the atoms comprising the irradi- in fillfag the initial created vacancy and the binding ated object; it increases markedly as the energy of the energy of the emitted or Auger electron. Because this incident photon decreases and the atomic number of process does not include any x-ray emission, it is called the irradiated atoms increases. Experimentally, the ~ A..._ _ _ ___.--1114~~:.i__C_H "'--=-- _ A_P...:T_:E:_R____:_ 3_ 1 ~n:...:..:: teracti on o f X- R:,:a:_:d:,.::ia : :t:.:..io :.n ~ W ~ it~h_M _ a_tt_e_r _ _ _ __ __ _ _ _ _ __ __ the reby permitting m ore radiation to reach the im age receptor. This result~ in a g:eater exposure to the phos. phor plate or the digital radiography receptor than fro Photoelectric absorption is the most important mode of the denser expanses of t issue.. The outcome is mo: interaction between x-radiat1on and the atoms of the adequate image contrast. As discussed with "air;' dis- patient's body in the energy range used in diagnostic sim ilar densities (mas~ density measured in grams per radiology because this interaction 1s responsible for cubic centim eter) of different body structures influence both t he patient's dose and contrast in t he image. Dur- attenuation. A d en sity increase le~ds t~ a corresponding ing the process of photoelectric absorption , the total increase in the number of atoms ma given volume with energy of the incident photon is completely absorbed as it interacts with and ejects an inner-shell electron of which x-ray photons can interact and therefore to an an atom within human tissue or bone from its orbit. The increased probability of photon absorption. Therefore newly ejected photoelectron has appreciable energy in any given sample of biologic material, both density and thus can subsequent ly ionize other atoms it en- and atomic number are important in determining counters until its energy is sufficiently depleted. After attenuation and ultimately imaging contrast. As an losing an electron, the original ionized atom is unstable example, if radiography is performed on an equal and attempts to re-stabilize. This occurs as an electron thickness of bone and soft tissue, the bone, which is from a higher shell drops down and fills the vacancy in approximately twice as dense as soft tissue, would the inner shell by relea sing energy as a characteristic absorb twice as much radiation due to the density photon. This cascading effect of electrons dropping difference alone. Furthermore, bone would absorb down to f ill existing shell vacancies continues until the original atom regains its stability. 6.5 times as much radiation because of the difference in effective atomic numbers since (13.8) 3 / (7.4)3 = 6.5. So, for equal thicknesses of bone and soft tissue, bone will absorb 13 times as many x- ray photons as soft tissue probability is observed to vary approximately as Z 3 /E 3 in the diagnostic energy range (Fig. 3.7A). Thus, in the radiographic kilovoltage range, compact bone with an effective atomic number* of 13.8 under- Body Part Thickness and Density Differences. Thick- goes much\ more photoelectric absorption than an ness of body parts also plays a role in absorption. The equal mass of soft tissue (Zerr approximately= 7.4) and thickness factor is approximately linear. If two struc- air (Zerr = 7.6). Consequently, because of the greater tures have the same density and atomic number but "x-ray shadow" that it casts, bone can be exceptionally one is twice as thick as the other, the thicker structure well delineated from soft tissue and air in diagnostic will absorb twice as many photons. Consider now t~e imaging. situation when there are different thickness and density Since air has only a slightly higher effective atomic values for adjacent structures: if a 2-cm-thick ?one number than soft tissue, the photoelectric interaction sample is radiographed n ext to a 4-cm-thick ussue occurrence probability for either substance is virtually sample, because the bone is h alf as thick in this e~ampl: identical. Thus, this interaction alone, for equal amounts but is approximately twice as dense, the de~sity an or volume densities of each substance, will not yield an thickness factors will cancel each other out (Fig. 3.7B). · how· imaging difference between the two media. The effective atomic number difference remains, ever, so that there will be 6.5 times as many x-raY Mass Density and Effective Atomic Number of. photons absorbed m the bone, and 1t w1 5. ·11 till cast a Different Body Structures. Since the density of air is noticeable "shadow" or produce directly a darker gray approximately 1000 times smaller than that of soft tis- effect on a digital detector image. sue, a given volume of air will interact with far fewer x-ray photons than adjacent regions of soft tissue, Effects of Attenuation on Radiographic Images: less a given structure attenuates radiation, the bng e !!; Effective atomic number (Z.rtl is a composite Z value by its appearance will be on a raw digital detector i~~g ~. I rad1at1° weight for a material that is composed of multiple chemical an d t h e more 1t absorbs radiat ion, the ess ' the elements. will be received by the detector a nd consequently CHAPTER 3 Interaction of X-Radiation W ith Matter X-ray photons X-ray photons Soft tissue Booe 1 91cm 3 1> - p- 2 g/cm3 Ziu - 7 4 Z.,.-13,8 1, Soft tissue Bone p ,1 g/~m3 p-2 gtom:1 Zutt ~ 7 4 Z.11 - 13.8 A Fig. 3.7 (A) Equal thickness of.bone and soft tissue are shown here The bone absorbs 13 times as many photons as 1he soft tissue A factor o,f 2. is.the result of bone's being approximately twice as dense as soft tissua. A factor of 6.5 is, the result of the higher atomic number of bone compared with that of soft tissue. Both factors together result In 2 x 6,5 = 13 times more absorption in this sample of bone. (Bl In the example sliown here, the soft tissue is twice as thick as the bone. Tots thickness difference approximately cancels out the density difference between t he bone and soft tissue. However, because the difference in atomic number still exists·, in this example the bone would absorb 6,5 times as many photons as the.soft tissue. NOTE. The curved arrows show n in this figure are only for general illustrative purposes and are not calculat1onal. darker a shad e of gray it will display as a raw image. A generated radiographic image, overall, must have a sufficient amount of variation in shades of gray, fo r an observer to clearly visualize anatomic structures of interest. These principles are illustrated in F ig. 3.8. In Fig. 3.9, two finaliz-e d posteroanterior (PA) hand projections illustrate age-related changes in bo ne density resulting from changes in calcium content. I. lmagc A exhibits subslantial quantities of calcium in the boQes of a young person. 2. lmage 13 exhjbits the demineralized bo nes of an el- derly person. The lesser amount of x~ray absorption resul.ts from the decrease in bone calcium. Hence the elderly person's bones are almost nonattenuating in radiographic appearance. Pathologic conditions such Fig. 3.8 Structures within the patient that are more attenuating (because of their physical density. thickness. and atomic num- as degenerative arthritis also contribute to d iffer- ber) will be displayed as brighter on the fmahzed Image. (From ences in absorption. Technical radiographic expo- Lamprgnano J, kendrick LE: Bontrager's textbook of rad10- sure facto.rs must be adjusted to compensate for such graph1c posrtioning and related anatomy, ed 10, St Louis, changes. 2621, Elsevier.) 111111 CHAPTER 3 Interaction of X-Radiation With Matter Fig. 3.9 (A) Posteroanterior (PA) image of a young person's hand exhibiting substantial quant it ies of calcium in the bones. (8) PA image of an elderly person's hand exhibiting demineralized bone as a consequence of a decrease in bone calcium. This and other degenerative changes account for the almost transparent appearance of the bones. Impact of Photoelectric Absorption on Radiographic must make the decision as to what technical factors to Contrast. Within the energy range of diagnostic radiol- use for a particular patient based on patient conditions ogy, the greater the difference there is in the amount such as existing disease processes. Often, with the ~d_of of photoelectric absorption, the greater the contrast an experienced radiographer and a medical physicist, in the radiographic image will be between adjacent the manufacturer's protocols can be modified at ~; structures of differing atomic numbers. However, as time of commissioning of a new x-ray unit. Even WI absorption by structures increases, so does the potential this, there will be some occasions when the rad'10gra· for biologic damage. To ensure both radiographic image pher may be called upon to manually adjust teehmq_ t. ues.a quality and patient safety, the radiographer should One of the simplest and best ways of reducing paU~ choose the technical factors that permit adequate radio- dose through technique adjustment is to raise the k graphic contrast while delivering the smallest dose (never the opposite, which would substantially inc_ to the patient. Usually, the selection of technique factors patient dose). The higher energy x-ray beam will is predetermined by the equipment manufacturer. How- more penetrating, thereby permitting a lower. ever, the manufacturer's technical factors are only a setting. This higher energy will, however, lead to a s}igb guide for the radiographer. Ultimately, the radiographer but still acceptable lessening of image contrast beca ,.:f~. \.. 'I' , CHAPTER 3 Interaction of X-Radiation With Matter 47 ~ - - -- - of a decrease in the amount of photoelectric interac- media. In addition, the inner-shell electrons of iodine tions at the higher beam energy. and barium have a binding energy that is in the energy range of the x-ray photons that are most commonly Use of Contrast Media to Ensure Visualization of used in general-purpose radiography (30 to 40 keV). Anatomic Structures. If tissues or structures that are This means that photoelectric absorption of the pho- similar in atomic number and mass density must be tons in the x-ray beam is further increased. In the radio- distinguished, the photoelectric interaction by itself graphic image, positive contrast-enhanced structures will not be sufficient to produce the imaging differ- therefore appear lighter than adjacent structures that ences needed in those tissues or structures to visually did not receive the contrast. Fig. 3.10A presents an differentiate them in the radiographic image. To re- anteroposterior (AP) projection of the abdomen with- solve the problem, the use of contrast media has been out the aid of a positive contrast medium to visualize adopted. Very simply, positive contrast m edia consist the urinary system, whereas Fig. 3.l0B presents an AP of solutions ( e.g., barium or iodine based) containing projection of the abdomen with a positive contrast elements having a higher atomic number than sur- medium that shows each contrast-filled structure in round ing soft tissue. These solutions are either swal- the urinary system to be distinguished. lowed to enhance the GI tract or directly injected into Caution must be exercised in the use of contrast the other tissues or structures to be visually enhanced. media because some patients may have adverse reac- The high atomic number of the contrast media (barium, tions to its presence. The use of a positive contrast Z = 56; iodine, Z = 53) significantly increases the medium also leads to an increase in absorbed dose occurrence of photoelectric interaction relative to adja- in the body structures that contain it. A negative con- cent similar structures that do not have the contrast trast medium such as air or gas can be used for some Fig. 3.10 (A) Anteroposterior (AP) proiection of an abdomen w ithout the aid of a positive contrast medium. Parts of the urinary system other than the kidneys, which have their own unique density, are not radiograph- ically demonstrated. (B) AP projection of an abdomen, following intravenous injection of an appropriate posi- tive contrast medium that permits visualization of the entire urinary system, thereby allowing each contrast- filled structure to be distinguished. (From Lampignano J, Kendrick LE: Bontrager's textbook of radiographic positioning and related anatomy, ed 10, St. Louis, 2021, Elsevier.) CHAfl"ffR ::J Interaction of X -Radiation With Matter radiologic examinations. These negative agents which discussion of this type of interaction). The freed are far easier to penetrate result in areas of decreased tron, called a Compton sca ttered electron ors elec.. ' econda brightness on the radiographic image. or recoil, electron, possesses excess energy a d ry, is potentially capable of ionizing other biolo. n thUs Compton SciJ~~li'i~g. I. g1c atoms In genera l, 1t oses its energy by a series of 11... co 1s1on Compton scattering is also known by the following with nearby atoms and finally recombines with s. an atom terms: that needs another electron. This usually occurs With'.. Incoherent scattering a few micrometers ( I 0·6 meters) of the site of the. ~.. orig1- Inelastic scattering nal Co mpton mteractlon. Modified scattering The incident x-ray photon that surrendered some of. It is responsible for most of the scattered radiation its energy (see Appendtx G) to free the loosely bo d produced during radiologic procedures. This scatter outer-shell electron from its orbit continues on its:: may be directed forward as small-angle scatter, to the but in a new direction, and is now called a Compt~ rear as backscatter, and laterally as side scatter. The scattered photon. It has the potential to interact with intensity of radiation scatter in various directions is other atoms, either by the process of photoelectric a major factor in planning protection for medical imag- absorption o r by subsequent Compton scattering. The ing personnel during a radiologic examination. photons can also emerge from the patient, possibly contributing to degradation of the radiographic image Process of Compton Scattering in a Patient. In a by adding to it an additional multidirectional off-focus typical Compton interaction event within a patient, exposure (radiographic fog). In fluoroscopy, these an incoming x-ray photon interacts with a loosely deflected exit photons may also expose personnel who bound outer electron of an atom (Fig. 3.11). On are present in the room to scattered radiation. This encountering the electron, the incoming x-ray photon scattering therefore mandates that such personnel wear surrenders a · portion of its energy in dislodging the protective lead shielding. If the presence of personnel electron from its outer-shell orbit, thereby ionizing is not immediately required in the x-ray room, they the biologic atom (see Appendix G for an extended should stand behind a protective barrier (Figs. 3.12 and 3.13). The Compton interaction's probability of occurrence Compton scattered has no explicit dependence on atomic number. Instead. electron it shows something of an energy and density depen- \ dence. Density dependence just means that the~~ , ,'.,...e atoms or targets per unit volume, the greater the~ Incoming hood of an interaction occurring in that volume. Wi x-ray photon increasing x-ray photon energy however, the chance for \ -...... Outer-shell electr I Scattered photon of less energy Ag. 3.11 Compton scattering. On encountering a loosely bound outer-shell electron, the incoming x-ray photon surren- ders a portion of its energy to dislodge the electron from its. f r ,nost ofth8 orbit. The energy-degraded x-ray photon then continues on its Fig. 3.12 Compton interactions are responsi~le ~ r ~ way, but in a new direction. The high-speed electron ejected scattered radiation produced during a ra?1oloic ~y'.s ,aJIJ' 05 from its orbit is called a Compton scattered electron, or (From Radiobiology and radiation protection: 1 secondary, or "recoil " electron. graphic instructional series, St. Louis, 1999, Mosby. '. -, CHAPTER 3 Interaction of X-Radiation With Matter 49 approxim ate 1/E dependen ce for Compton scattering. It is found that Compton scattering and photoelec tric absorptio n in tissue are equally probable at approxi- mately 35 keV x-ray photon energy. Therefore in a 100- kVp x-ray beam, where the photons will normally have an average energy in the range of 30 to 40 keV, signifi- cant numbers of Compton events occur. A summary of facts about the process of Compton scattering is presented in Box 3.4 for quick reference. Pair Production Pair production is a process that does not occur unless the energy of the incident x-ray photon is at least 1.022 million electron volts (MeV). Although this energy range is far higher than that used in diagnosti c radiol- ogy, a brief and very elementa ry descriptio n of the pair productio n interaction is included in this chapter to provide the reader with a broadsca le understan ding of the possible interactions of x- radiation with matter. Process of Pair Production. In pair productio n, the incoming x-ray photon strongly interacts with the elec- tric field surround ing the nucleus of an atom of the irradiated biologic tissue and subseque ntly disappear s / Small-angle " -Image receptor (Fig. 3.14). In the process, the energy of the photon is scatter absorbed and transformed into matter compose d of Fig. 3.13 Compton scattering results in all-directional scatter. two particles: a negatron (an ordinary electron) and a This scatter may be directed forward as small-angle scatter, to the rear as backscatter, and laterally as side scatter. The 1ntens1ty of radiation scatter in various directions is a major factor in planning the protection for medical imaging personnel BOX 3.4 Summa ry of the Process. during a radiologic examination. of Compto n Scattering _.. · · Compton scattering ts also important in the energy range used in diagnostic radiology Because the scat- a billiard ball-like interactio n between the photon and tered x-ray photon produced from the interaction of the an outer atomic electron decreases. What must be incoming photon w ith an outer-shell electron of an atom strongly emphasized is that the Compton interaction's of human tissue results only in a partial transfer of lack of Z dependen ce implies that it does not differen- energy to that biologic atom, the scattered photon now tiate between equal amounts of bQne and soft tissue traveling in a different direction can exit the patient and thus does not serve as a useful contrast mecha- and become a potential health hazard for imaging nism for radiograp hic imaging. Fortunately, as long as personnel by increasing their occupational radiation the radiograp her selects appropria te kVp technical exposure. In the event that Com pton scattered photons factors, the photoelec tric interactio n will provide that reach the image receptor, they can decrease contrast mechanis m. of the image by adding an undesirable add1t1onal off focus exposure called radiograph ic fog Because its In diagnostic radiology, the probability of occur- energy dependence decreases much more slowly w ith rence of Compton scattering relative to that of the pho- increasing energy than does the photoelectric interac- toelectric interactio n escalates as the energy of the x-ray tion, Compton scattering is very important even at 3 photon increases because of the 1/£ energy dependen ce therapeutic energies of the photoelec tric interactio n as contraste d to the CHAPTER 3 Interact ion of X-Radia tion With Matter (Mass (electron or positron ) = 9.1 x 10·31 kg Negatron 108 m/s, 1 MeV = 1.602 X 10-13 ii. C :::: 3 )( --- --r E(total) = E(electro n) + E(positro n) = sum of re t energy of each particle s mass = 2 x moc2 Incoming = 2 X (9.1 X 10·31 ) X (9 X 1016) x-ray photon (at least --.... \ \ = 16.38 x 10-14 joules ' electron = 1.022 MeV 1.022 MeV) \\ /~ ~-"" t diagnos tic x- ray imaging. The electron of the pai 1 en ergy by exciting and ionizing atoms in its P:t~ses " ' Resulting photons (0.5 11 MeV each) eventually loses enough energy that it may be capt · It by an atom in need of another electron. RegardinguthredC Fig. 3.14 Pair production. Pair production occurs when incom- · kn generat ed positron , as 1ar.as.1s own, no large quanti-. C ing x-ray photons with energy of at least 1.022 MeV interact.. fr with the nucleus of an atom. For a 1.022-Me V event. the end ~1es of ~os1tro ns ee1y exist _m the universe. A positron result of this interaction is the annihilation of a positron and an 1s classified as a form of antimatter because it is seen 1 electron with their rest masses converted into energy, which interact destruct ively with any nearby ordinary matt; appears in the form of two 0.511-MeV photons moving in the opposite direction. that it may be attracte d to, such as an atomic electron. During such an interact ion, the positron and the elec- positron (a positively charged electron ). The negatro n tron will annihila te each other, in the process converting and the positron have the same mass and magnitu de matter into energy. This energy is called annihilation of charge; the only difference is in the "sign" of their energy. This is the reverse of a pair production event in electrical charges. The incomin g photon must have which energy (that of a photon ) is converted to matter. enough energy to supply the combin ed rest mass* of Both types of process es happen in accordance with these two particles; otherwi se, the process does not Albert Einstein 's famous concep t of mass-energy equiv- happen. The minimu m energy required to produce an alence, mathem atically express ed as E = mc2 (c is the electron- positron pair is 1.022 MeV (Box 3.5). For this speed of light in a vacuum ). The energy that appears reason, pair product ion does not influenc e ordinar y from the annihil ation of the electron and positron does not disappe ar but rather, in accorda nce with the conser- vation laws of energy and momen tum, is carried off by two 0.511-M eV photon s moving in opposite direc- *Rest mass is a term associated with the mass a particle tions when a pair produc tion event occurs at 1.022 MeV. possesses or would be measured to have when it is at rest rela- tive to the frame of measurement. According to the theory Althoug h pair produc tion does not occur unless the of special relativity, the mass of an object is in general not a energy of the incomi ng photon is at least 1.022 MeV,its fixed value but rather is dependent on the magnitude of its probabi lity of occurre nce starts to become significant velocity "v" according to the relation: (i.e., noticea bly gr~ater than zero) at 10-MeV x-ra, m = m0 I (I - v 2/ c2 ) energie s and higher. where m0 is the rest mass value of the particle and c is the speed of light in a vacuum. As can be seen from this expres- Use of Annihilation Radiation in Positron EmissiOI sion, if the speed of the object is zero, then its mass is equal to Tomography. Annihi lation radiatio n is used in an m0 , but if the speed is not zero, then the mass of the particle imaging modalit y employ ed in Nuclear Medicine called will be substantially increasing as its speed approaches a p£1' significant fraction of the speed of light. The expression also positron emission tomography (PET). Briefly, in. the~~. scannin g of patients , the source of the pos1tro shows that a partide with a nonzero rest mass can never ·. unsta· be brought to the speed of light, for then its mass m would mJected radionu clides, whose atomic nuclei are. become infinite, and the amount of energy required to achieve ble because they contain too many protons rel~t'.VC this would also be infinite. to their numbe r of neutron s. To relieve this in 5labilit)', , : - ;--_·{(.. \ :, ':~.. ' \ , :- CHAPTER 3 Interaction of X-Radiation With Matter 51 -~- r ~ a surplus proton is converted in the nucleus into a neutron, and a positron and another particle called a 11eutri110 are ejected from the nucleus. Within a very short distance (several micrometers or less), the emitted positron interacts with a local electron, and the two mutually annihilate, yielding a pair of photons emerg- ing in opposite directions from the electron-positron interaction site. These annihilation photons are inter- cepted by a ring of detectors surrounding the patient. l I The positional information from these detectors is then used to build a cross-sectional image of the radioactiv- ity distribution within the patient. Some examples of Incident photon Fig. 3.15 Photodis1ntegration. An incoming high-energy pho- radionudidcs used in PET scanning are: ton colltdes with the nucleus of an atom and absorbs all the Fluorine- 18 ( 18F) photon's energy. This energy excess in the nucleus creates Carbon- 11 (1 1C) an instability that is usually alleviated by the emission of a Nitrogen-13 ( 13N) neutron. In addition, 1f suff1c1ent energy is absorbed by the nucleus, other types of emissions will be possible, such as a Photodisintegration proton or proton-neutron combination (deuteron), or even an alpha particle. Photodisintegration is another interaction that be- comes important at x-ray energies exceeding 10 McV during the operation of high-energy radiation therapy This energy excess in the nucleus creates an instability treatment machines. Because this energy range is also that in most cases is alleviated by the emission of a neu- far higher than useful diagnostic energies, only a brief tron by the nucleus. Other types of emissions-a proton account of this interaction process of radiation with or proton-neutron combination (deuteron) or even an matter is included. With this discussion and those alpha particle (two protons and two neutrons bound directly preceding it, the reader will have been intro- together)-are possible if sufficient energy is absorbed duced to all of the important types of radiation and by the nucleus. Because emission of charged and/or matter encounters. uncharged particles has occurred from a previously inactive nucleus, it can be said that the photodisintegra- Process of Photodisintegration. In photodisintegra- tion interaction has made a nucleus partially break tion, a high-energy photon collides with the nucleus of apart. Fig. 3.15 demonstrates one type of photodisinte- an atom, which directly absorbs all the photon's energy. gration event. I SUMMARY Peak kilovoltage (kVp) controls the quality, or pen- Variations in x-ray absorption properties of various etrating power, of the photons in the x-ray beam and body structures make radiographic imagi ng of to some degree also affects the quantity, or number human anatomy possible. of photons, in the beam. Radiographers receive less radiation exposure when Because radiographers select the technical exposure the patient's dose is minimal because less radiation factors, they are responsible for the radiation dose is scattered from the patient during an imaging the patient receives during an imaging procedure. procedure. The amount of energy absorbed by the patient per The anode of an x-ray tube is usually made of tung- unit mass is called the absorbed dose. sten or an alloy of tungsten and rhenium. Biologic damage in the patient may result from the The units kVp and kV refer to the voltage on the x-ray absorption of x-ray energy. However, some small tube, and keV refers to the energy of specific x-rays. amount of radiation dose is necessary to produce a Attenuation results when, through the processes of diagnostic image. absorption and scatter, the intensity of the primary CHAPTER 3 Interaction of X-Radiation With Maner Photons m · an x-ray beam decreases as it passes when kVp is decreased, the number of photoeleq. through m atter. interactions increases and the number of Corn flc. decreases,. h owever, t h e patient. Pton Scattered radiation can result in diminished contrast of interactwns absor bs the image by adding additional undesirable exposure more energy, and therefore the dose to the Pat·1 ent to the image receptor (radiographic fog). In fluoros- increases. copy, Compton scattered photons may expose person- When kVp is increased, the patient receives a lo wer nel who are present in the room to scattered radiation. dose, but image quality may b e compromised. Two interactions of x- radiation are central in diag- kVp selection is usually based on type of proced. ure nostic radiology: photoelectric absorption and and body part imaged. Compton scattering. The photoelectric absorption is Radiographers must balance other variables, such the basis of useful radiographic imaging, whereas as the type of im age receptor used, patient thick. Compton scattering is its bane. n ess, and degree of muscle tissue, to arrive For each radiographic procedure, an optimal peak technical exposure factors that will provide an a:~ kilovoltage (kVp) and milliampere-seconds (mAs) ceptable image yet stay within the standards of combination exists that minimizes the dose to the radiation protection. patient and produces an acceptable image. Coherent scattering is most likely to occur at less than Within the energy range of diagnostic radiology 10 keV; pair production and photodisintegration oc- (23 to 150 kVp), which also includes mammography, cur far above the range of diagnostic radiology. cu