Chapter 3 Nutrient Cycles in the Biosphere PDF
Document Details
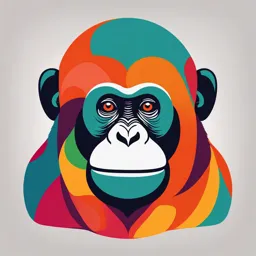
Uploaded by SecureIodine9855
Tags
Summary
This document discusses nutrient cycles in the biosphere, focusing on the roles of key elements like oxygen, carbon, hydrogen, and nitrogen. It also explores the interactions between biological organisms, their environment, and the movement of nutrients. It is likely part of a larger educational module for an undergraduate science course.
Full Transcript
CHAPTER 3: NUTRIENT CYCLES IN THE BIOSPHERE Four elements dominate the composition of the biosphere. These are oxygen, carbon, hydrogen, and nitrogen. These elements constitute all but a small fraction of the average terrestrial vegetation. This, in turn, makes up more than 99% of the wo...
CHAPTER 3: NUTRIENT CYCLES IN THE BIOSPHERE Four elements dominate the composition of the biosphere. These are oxygen, carbon, hydrogen, and nitrogen. These elements constitute all but a small fraction of the average terrestrial vegetation. This, in turn, makes up more than 99% of the world’s standing crop. The brief constituent of plant materials is wood, which is mainly cellulose. Carbon, hydrogen, and oxygen are the main constituents of cellulose. The chemical formula of cellulose is (C 6H10O5)n. Carbohydrate (CH2O)6, the principal product of photosynthesis is likewise made up of these three elements. Water, which is composed of hydrogen and oxygen, is another vital substance in the biosphere. Organic molecules in living organisms are dispensed in aqueous (water) medium. Animals (including humans) and most fruits and vegetables have about 65-70% water content. Hence, the biosphere is described as both wet and carbonaceous. Carbon makes up 49% of the dry weight of organisms. Nitrogen is present in two important biomolecules- proteins and nucleic acids (e.g., DNA and RNA). The other elements that are part of the biosphere are those that we commonly associate with nonliving origin (those that come from nonliving) except for two elements- sulfur and phosphorous. These inorganic elements appear in the ash that is left when organic matter is burned; carbon, hydrogen, oxygen, and nitrogen compounds are volatilized. Ash, which is about 12 parts per trillion (ppt) of the total; contains the elements calcium, potassium, silicon, sodium, and magnesium, and other minerals. These elements perform important biochemical roles. Sulfur is present in some proteins. The characteristic of odor of burning hair and spoiled egg is due to sulfur. Sulfur is considered the “stiffening” factor of proteins. Sulfur-sulfur bonds form between segments of a protein molecule, causing the chain to be folded and shaped in a particular way. Once its intrinsic shape is destroyed, a protein can no longer perform its function. This happens when the sulfur bonds are broken during denaturation. Phosphorous is essential for two body functions--- for the transfer of energy and as a component of genetic material. It is present in adenosine triphosphate (ATP), the universal fuel for biochemical processes in living cells. Biogeochemical Cycles The air, seas, and rocks interact and exchange components. The flow of chemical elements in the biosphere involves biological organisms (producers, consumers, and decomposers) in their geological environment (soil, air, and water). It involves both biotic and abiotic chemical reactions. Hence, the cyclic movements of these nutrients in the environment are called biogeochemical cycles. The sun is the primary source of energy that moves the nutrients around the biosphere. Material Page | 1 and energy conversions occur in the different stages of the nutrient cycles. Nutrient cycling changes nutrients from one forms to another as they circulated back and forth from one part of the environment to another. To understand the biogeochemical cycle of any biologically important element requires knowledge of chemical processes that operate in the biosphere, lithosphere, atmosphere, and hydrosphere. The biosphere is the locus of interactions of the five elements present in the atmosphere- --carbon, hydrogen, oxygen, nitrogen, and sulfur. These elements move either alone as free elements (e.g., O2 and N2) or in combination of compounds (e.g., H2O, CO2, SO2, H2S, nitrates, and carbohydrates). Their mobility depends on physical state (solid, liquid, and gas) and solubility in water. Soluble elements may be carried through the hydrologic or water cycle (Figure 1), even in parts. Near Earth’s surface, water is continuously recycled by runoff, evaporation, and precipitation. Water flows in rivers from lithosphere to the hydrosphere. From the hydrosphere, to the atmosphere, by evaporation and washes the land by precipitation. The cycling of nutrients around biosphere involves two basic processes—physical transport and chemical transformation. Physical transport moves the nutrients across the boundaries of the different physical environments. The water cycle, through precipitation, evaporation, or mere flow of water can carry along with its substances from one part of the environment to another. There are other physical agents that move nutrients across the boundaries of the geologic environment. These physical agent include the wind, ocean currents, geologic movements, upwelling of oceans, and movements of organisms. Ocean currents ensure the continuous mixing dissolved materials and their transport to far places. Organic debris deposited at the bottom of the oceans surfaces through seasonal upwelling. Geological events such as volcanic eruption expel from the bowels of Earth tons of buried minerals and release them to the surface and atmosphere. Sulfur dioxide and dust particles emitted by Mt. Pinatubo in 1991 were carried around the world by wind. Birds and humans that eat fish move nutrients from hydrosphere and deposit these into the lithosphere after these nutrients have taken part in the metabolic processes. The cycling of the typical element X is shown is Fig. 2, it shows the overall global dynamic interactions of living organisms with their chemical and physical environments. The element undergoes various chemical transformation as it moves through the living and nonliving components of the biosphere. The major producers, the autotroph, utilize the simple forms of element X (e.g. CO2 and H2O) in photosynthesis and transform it to more complex forms like carbohydrate and proteins. It is at this point that the energy flow in the biosphere links with the nutrients cycles. A chain of consumers, the heterotrophs, converts the complex form of X to less complex forms as products of their metabolic process. Wastes from both producers and consumers are acted upon decomposers, changing complex forms of X into simpler forms. The product of metabolic activity of decomposers may then return to any of the three geological stations of the environment. Later, they may be utilized again by producers when a new cycle starts. Page | 2 Carbon, Hydrogen, and Oxygen Cycles The carbon, hydrogen, and oxygen cycles intersect in green plants. Photosynthesis removes CO2 from its pool in the atmosphere and water from its pool near earth surface. The product of photosynthesis are fixed carbon compounds in the form of carbohydrates and other organic compounds. These product add to the biomass or environmental pool of stored organic matter. The other product, oxygen, is released to the atmosphere. Plants and organic compounds may be eaten by herbivores and go through their metabolic pathways. A small part of the biomass joins the pool of dead organic matter. The final product of both metabolism and composition are carbon dioxide (CO2) and water. When oxygen supply is limited, decomposition occurs anaerobically, yielding methane. Methane and carbon dioxide are both volatile and thus are highly mobile. They both return to the atmosphere. In time, methane may be oxidized to CO2. Water vapor, likewise, is released to the atmosphere through transpiration by plants, oxidative respiration, or decomposition of organic matter. Carbon Reservoirs and Fluxes The carbon cycle which is at the core of biogeochemistry, involves the movement of carbon atoms through the life support systems on the surface of the Earth. When talking about the carbon cycle, the phrases “reservoirs carbon” and “fluxes between reservoirs” are used. Reservoirs, defined simply, is storage. Examples reservoirs are oceans, the atmosphere, the biosphere, soil carbon, carbonate sediments, and organic sediments. The fluxes between reservoirs describe the rate at which atoms move from one reservoirs to another, such as for example, the rate of movement of carbon between the soil and the atmosphere. The Figure 4 shows the major carbon reservoirs in the environment. Carbon is stored in various areas above and below Earth. Also shown is the carbon flux from one carbon storage to another. GtC is gigation carbon, where 1Gtc= 1 billion metric tons = 10 12kg. The oceans estimated to have 50 times more carbon than the air. A large amount of carbon is present in the shells and exoskeletons of ocean’s invertebrates as calcium carbonate (CaCO 3). When these organisms die, part of these hard coverings be buried in the sand and mud and become isolated from biological activity. Through geological times, these carbon deposits formed our coral reefs and limestone rock. Fossil fuels are likewise the products of the long and slow transformation of carbon materials under very high pressure and temperature. The major bulk of the near-surface carbon consist of Page | 3 geological deposits. About 99% of the total carbon is in the form of fossil deposits – peat, shale coal, petroleum, and the remains of mollusk shells and protozoan in carbonate rocks-that required hundreds or even million years to form. As reservoirs of carbon, oceans tend to regulate the amount present in the atmosphere. Carbon dioxide diffuse in and out of the ocean’s surface. CO2 ⇋ CO2 The residence time for carbon reservoir is the average time the carbon remains in that reservoir at a steady state. This turnover time can be determined by dividing the size of the reservoir by the size of the outgoing flux. As mention earlier, some CO2 in the air may ride on the water cycle as dissolved carbonic acid (H2CO3). Carbonic acid exists in the equilibrium with CO2, bicarbonates (HCO3-), and carbonates (CO 3-). CO2 + H2O ⇋ H2CO3 ⇋ H+ + HCO3 ⇋ 2H+ + CO3 -2 Respired CO2 adds to the small amount already present in the atmosphere. This is in balance with CO2 in the oceans and in other parts of hydrosphere. Through other interactions, CO 2 may be removed from circulation as CaCO 3 precipitated from solution. Carbon dioxide thus sequestered may eventually return to the atmosphere when limestone formed by consolidation of CaCO3 sediments, emerges from the sea and is dissolved by some future rainfall. Carbon circulation on Land. Forest are not only main CO2 consumers on land but they are also the main reservoirs of biologically fixed carbon. Forests contain roughly 100-500 billion tons of carbon or 2/3 the amount present as CO 2 in the atmosphere (700 billion tons). With 30 years as the average lifetime of a tree, about 15 billion tons of carbon in the form of CO 2 is annually transformed into wood. The carbon fixed by photosynthesis on land returns to the atmosphere through the decomposition of dead organic matter. This happens after it has gone through a series of decay processes in soil. Carbon-14 dating provides information on the rate of decomposition of organic matter. Carbon circulation in the Sea. Most of the carbon in the sea is self-contained. The oxygen released by the photosynthesis of phytoplankton is consumed by zooplankton and other sea animals. These, in turn, consumed by larger animals, which ultimately decompose and release CO2 into solution. There is a dynamic exchange of CO2 and O2 between the atmosphere and the sea. This is brought about by the action of the wind and the waves. At the given moment, the concentration of CO2 and O2 gases dissolved in the surface layers of the sea is closely equilibrium with their concentration in the atmosphere. Oxygen Pools. Oxygen has a more complex cycle because of its reactivity. The three major nonliving sources of oxygen atoms are CO 2, H2O, and O2. These molecules exchange oxygen atoms and can be considered as a common pool. Some mineral oxides such as nitrated and sulfates are also sources of oxygen for living organisms, which reduce these oxides to NH 3 and H2S respectively. Another source of oxygen in the atmosphere is photolysis. This reaction is ultraviolet dissociation of water vapor in the atmosphere into hydrogen and oxygen, followed by the escape of the hydrogen from Earth’s gravitational field. Photolysis is usually regarded as a trivial oxygen source based on the oxygen-carbon balance sheet. In addition, inhaling oxygen and exhaling carbon dioxide, humans decrease the oxygen level and increase the carbon dioxide level by burning fossil fuels and paving green lands. Oil spills and pesticides may affect the photosynthetic capacity of phytoplankton in the oceans. Page | 4 Nitrogen, Sulfur, and Phosphorus Cycles The atmosphere is the major pool of nitrogen. It has 78% nitrogen by volume. The nitrogen cycle (Fig. 5) involves the removal of the free element (nitrogen gas) from the atmosphere, the utilization by living organisms of complex nitrogen compounds, and the removal of the simpler ammonia compound from the soil and decaying organic matter. Plants take up nitrogen from the soil in the form of nitrate (NO3). The nitrogen from the soil is converted into organic nitrogen by plants in the form of proteins and other macromolecules, and passed on to animals by consumption. When plants and animals are dead, through decomposers like microorganism and some fungi, the organic nitrogen is converted into ammonia (NH3) by the process of Ammonification. In the soil, the ammonia is then converted to nitrite (NO2) and back to nitrate by the process of Nitrification. The formation of nitrate are the result of the activities of nitrifying bacteria of the genera Nitrosomonas and Nitrobacter. In taking up nitrate from the soil, plants must compete with bacteria known as denitrifiers (e.g.,Thiobacillus denitrificans). By the process of Denitrification, these bacteria reduce nitrate to dinitrogen (N2), which is then returned to the atmosphere. Lastly, the atmospheric nitrogen is then fixed through biological, industrial, and electrical fixation back to the soil. Nitrogen Fixation Processes Atmospheric Fixation. Cosmic radiation, meteor trails, and lightning provide momentarily the high energy needed for nitrogen to react with oxygen. Nitric Oxide (NO) and nitrogen dioxide are formed, which then react with water, forming nitrous acid (HNO2) and nitric acid (HN03). When it rains, these acids are washed into the soil and then assimilated by plants. Industrial Fixation. In 1914, German Chemists Fritz Haber and Karl Bosch invented the industrial process of nitrogen fixation, nitrogen obtained from the atmosphere is catalytically reduced with NH 3 under high pressure and temperature. Biological Fixation. Free living bacteria (e.g., Blue-green algae) and bacteria that form symbiotic associations with plants (e.g., Azobacteracaea) are able to fix assimilated nitrogen and convert them into useful compounds. Terrestrial microorganism and symbiotic associations between microorganisms and plants are the largest single natural source of nitrogen. Nitrogen-deficient soil can be improved by crop rotation using leguminous plants. Environmental Problems Associated with Nitrogen Excessive of nitrogen compounds in streams and rivers can result in algal bloom and intensified biological activities. These can deplete the available oxygen and destroy the fish and other oxygen-dependent organisms in water. The series of steps leading to the gradual dying of water is called eutrophication. In some cases where fertilizers and nitrogenous wastes are not carefully managed in waterways, nitrogen could exceed the acceptable level of concentration. Page | 5 Sulfur Cycle The sulfur cycle (Fig. 7) involve both atmospheric and a sedimentary phase. The reduction of sulfur, together with the reductions of carbon and nitrogen, is critical step in the synthesis of proteins. Carbon is reduced during photosynthesis in the presence of sunlight. The reduction of sulfur and nitrogen, on the other hand occur in dark, oxygen-deficient places. The most well-known sulfur reducers, called obligate anaerobes, thrives in swamps marshes, and floors of eutrophic lakes. The hydrogen sulfide gas produced by reduction of sulfur escapes to the atmosphere. There, it is converted into oxides, which reacts with water to form sulfuric acid mists. This can damage marble, limestone, and mortar and make them pitted and discolored. A concentration of 0.1-0.5ppm SO2 in air is harmful to humans and plants. With a very high level, acid rain can form. Acid rain contains soluble sulfate and sulfuric acid. In recent years, people have contributed to the sulfur content of the atmosphere. About a third of the total sulfur in air comes from industrial sources specifically from the burning fossil fuels. Phosphorous Cycle Phosphorous is a scarce element. It cannot be carried by evaporation to the atmosphere because it has no common volatile forms. Its soluble forms track the hydrologic cycle pathway from lithosphere to the hydrosphere. Its primary reservoir are rocks and sediments. The phosphorous cycle (Fig. 8) operating in the ocean which is the sink for the element. Soluble phosphates in runoffs from land are incorporated as organic phosphates in marine organisms. When these organism die, decay returns soluble phosphates to water. Soluble phosphates can be taken up by plants and recycled through living organisms. Some phosphates may be immobilized in sediments by reaction with calcium, aluminum, manganese, and iron. Through the upwelling of the ocean, phosphates may resurface some time. This nutrient upwelling allows many forms of marine life to grow. Phosphorous moves around with an almost one-way interrupted flow from the lithosphere to the hydrosphere. Agricultural activities such as the application of phosphate fertilizers to farms return marine phosphates into the lithosphere in ever-increasing amounts. Phosphate is released to the environments as rock erode. Animals ingest phosphorous and excrete the excess in feces. Guano, or the excreta of bats and some birds, is a rich source of this element. Phosphates accumulate in areas with low rainfall, and they remain on the surface. Some detergents contain phosphates as builders. When discharged into waterways, the phosphorous can stimulate algal growth. When these algae die, they then decay, using up the dissolved oxygen in water and eventually lead to eutrophication of the polluted water. Page | 6