Chapter 24.1-24.2 Prokaryotic Diversity PDF
Document Details
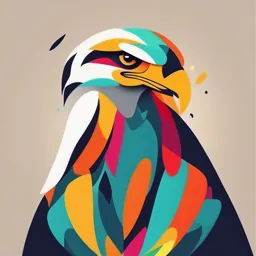
Uploaded by JubilantDrums9581
Oklahoma Christian University
Tags
Related
- Lecture 12 - Bacteria, Archaea and Fungi PDF
- Prokaryotes: Bacteria and Archaea PDF
- Chapter 2: The Prokaryotes (Domains Bacteria and Archaea) PDF
- Chapter 2: The Prokaryotes (Domains Bacteria and Archaea) PDF
- Lecture 5: Prokaryotes - Biodiversity of Bacteria & Archaea
- Chapter 25: Bacteria and Archaea (Biology Eighth Edition) PDF
Summary
This document's contents cover the key concepts of prokaryotes, highlighting crucial differences between bacteria and archaea, such as their cell walls, membrane lipids, and gene expression. It emphasizes the significance of these organisms in evolutionary history, their diversity, and the roles they play in various environmental contexts.
Full Transcript
24.1 notes Key Concepts 1. The Tree of Life The Tree of Life is a phylogenetic tree that represents the evolutionary relationships among all life forms. It is divided into three main domains: Bacteria Archaea Eukarya (includes plants, animals, fungi, protists) The divergence between Bacteria and Ar...
24.1 notes Key Concepts 1. The Tree of Life The Tree of Life is a phylogenetic tree that represents the evolutionary relationships among all life forms. It is divided into three main domains: Bacteria Archaea Eukarya (includes plants, animals, fungi, protists) The divergence between Bacteria and Archaea is an ancient event, and these two domains represent early forms of life that are distinct from eukaryotes. 2. Prokaryotes: Bacteria and Archaea Prokaryotes are unicellular organisms that lack a nucleus and membrane-bound organelles. Despite sharing the absence of a nucleus, Bacteria and Archaea differ in key biochemical and genetic ways. 3. Key Differences Between Bacteria and Archaea Cell Wall Composition: Bacteria typically have peptidoglycan in their cell walls. Archaea do not have peptidoglycan; their cell walls are made of other compounds like pseudomurein or S-layer proteins. Membrane Lipids: Bacteria have ester bonds linking their membrane lipids. Archaea have ether bonds, which are more stable and are thought to help them survive in extreme environments. Gene Expression: Archaea and eukaryotes share more similarities in RNA polymerase and protein synthesis machinery than Bacteria do. Some archaea even have histone proteins (like eukaryotes), which help package their DNA. Ribosomal RNA (rRNA): Analysis of rRNA sequences is one of the main methods used to distinguish between Bacteria, Archaea, and Eukarya. Archaea and Eukarya share more similarities in their rRNA sequences than either shares with Bacteria. 4. Extremophiles in Archaea Archaea are well-known for living in extreme environments, earning them the nickname extremophiles. Examples of extremophilic environments include: Thermophiles: High-temperature environments (e.g., hot springs, hydrothermal vents). Halophiles: Salt-rich environments (e.g., salt ponds, salt mines). Acidophiles: Acidic environments (e.g., sulfuric hot springs). Methanogens: Anaerobic environments where methane is produced (e.g., in the guts of animals, wetlands). 5. Evolutionary Significance of Bacteria and Archaea Both Bacteria and Archaea are thought to have evolved early in Earth's history, with some of the oldest fossil evidence of life (e.g., stromatolites) attributed to cyanobacteria (a type of bacteria). The last universal common ancestor (LUCA) is the hypothetical organism from which all modern life descended, and it is thought to have been more similar to archaea than bacteria. The presence of extremophiles in Archaea suggests that life on Earth may have begun in extreme conditions. 6. Genetic and Metabolic Diversity Bacteria and Archaea show incredible genetic diversity and metabolic pathways: Bacteria are more diverse in terms of metabolic pathways and can be found in almost every environment on Earth. They are involved in essential ecological functions such as nitrogen fixation, decomposition, and disease. Archaea also have diverse metabolic pathways but are especially important in processes like methanogenesis (producing methane), fermentation, and the cycling of sulfur and nitrogen. 7. Endosymbiosis and the Origin of Eukaryotes There is evidence suggesting that eukaryotes (cells with a nucleus) may have evolved from an endosymbiotic relationship between early archaea and bacteria. Specifically, mitochondria and chloroplasts in eukaryotic cells are thought to have originated from free-living bacteria that were engulfed by an ancestral eukaryotic cell. 8. Modern Classification The traditional view of classifying life into two kingdoms (Animalia and Plantae) has been replaced by the three-domain system (Bacteria, Archaea, and Eukarya). Molecular techniques (e.g., 16S rRNA sequencing) have significantly changed how organisms are classified, highlighting the deep genetic distinctions between Bacteria and Archaea. Summary Bacteria and Archaea represent the earliest branches in the tree of life, having split early in evolutionary history. While they share some characteristics as prokaryotes, they differ significantly in their genetic makeup, cell structure, and biochemical pathways. Archaea are well-known for thriving in extreme environments and share some molecular similarities with eukaryotes. The study of bacteria and archaea provides critical insights into the early evolution of life on Earth and the origin of eukaryotes. Chapter 24.2: Prokaryotic Diversity Reflects the Ancient Origins of Life 1. Two Early Branching Lineages of Bacteria Live at Very High Temperatures Thermophiles (heat-loving organisms) are bacteria that thrive in extremely high-temperature environments such as hot springs, hydrothermal vents, and volcanic areas. These bacteria represent some of the earliest forms of life on Earth, showing that life may have originated in extreme conditions. Thermophiles have adaptations that allow them to survive in hot environments, such as specialized proteins and enzymes that function at high temperatures. 2. Firmicutes Include Some of the Smallest Cellular Organisms Firmicutes are a phylum of bacteria known for their small size and ability to form spores. Some of the smallest cellular organisms in the world belong to this group, such as Mycoplasma species, which lack a cell wall. Firmicutes include both pathogens (e.g., Staphylococcus, Clostridium) and beneficial microbes (e.g., those involved in fermentation and digestion). 3. Actinobacteria Include Major Pathogens as Well as Valuable Sources of Antibiotics Actinobacteria are a diverse group of bacteria, many of which are pathogenic (e.g., Mycobacterium causing tuberculosis and leprosy). Actinobacteria are also known for their production of antibiotics, such as streptomycin and tetracycline. These bacteria play a crucial role in the pharmaceutical industry due to their ability to produce substances that inhibit the growth of other bacteria. 4. Cyanobacteria Were the First Photosynthesizers Cyanobacteria (also known as blue-green algae) were among the first organisms to perform photosynthesis. They used sunlight, water, and carbon dioxide to produce oxygen as a byproduct, contributing to the Great Oxygenation Event (about 2.4 billion years ago), which significantly increased the oxygen levels in Earth's atmosphere. Cyanobacteria are responsible for oxygenating the planet, making it possible for aerobic life forms to evolve. 5. Spirochetes Move by Means of Axial Filaments Spirochetes are a group of bacteria characterized by their helical shape and unique method of locomotion. They move using axial filaments, which are flagella-like structures located in the periplasmic space (between the cell membrane and outer membrane), enabling the bacterium to move in a corkscrew motion. Spirochetes include pathogenic species such as Treponema pallidum (syphilis) and Borrelia burgdorferi (Lyme disease). 6. Chlamydias Are Extremely Small Parasites Chlamydias are a group of bacteria that are obligate intracellular parasites, meaning they must live within the cells of a host organism to survive. They are extremely small and lack many of the features found in other bacteria (such as peptidoglycan in their cell walls). Chlamydias include important human pathogens, such as Chlamydia trachomatis, which causes chlamydia, one of the most common sexually transmitted infections. 7. The Proteobacteria Are a Large and Diverse Group Proteobacteria represent one of the largest and most diverse groups of bacteria, comprising a wide range of shapes, metabolic pathways, and ecological niches. This group includes many important pathogens (e.g., Escherichia coli, Salmonella, Vibrio cholerae) as well as nitrogen-fixing bacteria that play essential roles in the nitrogen cycle. The Proteobacteria are further classified into five major classes: Alpha, Beta, Gamma, Delta, and Epsilon. 8. Gene Sequencing Enabled Biologists to Differentiate Archaea from Bacteria The advent of gene sequencing techniques, particularly 16S rRNA sequencing, has allowed biologists to study genetic relationships between organisms. Archaea and Bacteria were historically grouped together as prokaryotes but are now known to be distinct domains based on their genetic differences, including their rRNA sequences. This has led to a much clearer understanding of the evolutionary divergence between these two groups of microorganisms. 9. Archaea Live in Extremely Diverse Environments Archaea are known for their ability to thrive in some of the most extreme environments on Earth, such as hot springs, acidic lakes, and salty environments (e.g., the Dead Sea). Methanogens (a type of archaea) produce methane and are often found in anaerobic environments such as wetlands or the digestive tracts of animals. Archaea also include halophiles (salt lovers), thermophiles (heat lovers), and acidophiles (acid lovers). Chapter Title: Ecological Communities Depend on Prokaryotes 1. Many Prokaryotes Form Complex Communities Biofilms: ○ Biofilms are structured communities of microorganisms, including prokaryotes, that attach to surfaces and are encased in a self-produced extracellular matrix of polysaccharides and proteins. ○ Biofilms are found in many environments, including medical devices (e.g., catheters, prosthetics), industrial pipes, and natural environments (e.g., riverbeds, ocean floors). ○ Prokaryotes in biofilms communicate through quorum sensing, a process where bacteria release signaling molecules to coordinate behavior, such as biofilm formation, virulence factor production, and nutrient acquisition. ○ Quorum sensing allows prokaryotes to act collectively, which can enhance their survival and resistance to environmental stress (e.g., antibiotics). Inter-species Communication: ○ In biofilms, different species of prokaryotes and sometimes eukaryotes can coexist and exchange genetic material, often leading to genetic diversity and adaptive evolution. ○ Prokaryotic communities in biofilms can exhibit cooperative behaviors (e.g., nutrient exchange) as well as competitive behaviors (e.g., production of antimicrobial compounds). 2. Microbiomes are Critical to the Health of Many Eukaryotes Microbiome Definition: ○ The microbiome refers to the community of microorganisms (bacteria, archaea, viruses, fungi) that inhabit various parts of an organism, including skin, gut, mouth, and other mucosal surfaces. Human Microbiome: ○ The human body hosts trillions of microorganisms that play critical roles in digestion, immune function, metabolism, and protection against pathogens. ○ Gut microbiota help in the breakdown of complex carbohydrates, synthesizing essential vitamins, and maintaining gut health. Mutualistic Relationships: ○ Many prokaryotes in microbiomes have symbiotic relationships with their host, benefiting from nutrients and a stable environment, while providing services to the host in return. ○ Examples of mutualistic interactions include nitrogen-fixing bacteria in plant roots and the bacteria in the human intestines that aid in digestion. 3. A Small Minority of Bacteria Are Pathogens Koch's Postulates: ○ Koch's postulates are criteria used to establish a causative relationship between a microorganism and a disease. They include: 1. The microorganism must be present in all cases of the disease. 2. It must be isolated from the host and grown in pure culture. 3. When introduced into a healthy host, the microorganism must cause the disease. 4. The microorganism must be re-isolated from the experimentally infected host. Endotoxins and Exotoxins: ○ Endotoxins are toxins associated with the outer membrane of Gram-negative bacteria (e.g., lipopolysaccharides) that are released when the bacteria die or divide. They can trigger immune responses, leading to inflammation and fever. ○ Exotoxins are proteins secreted by bacteria (both Gram-negative and Gram-positive) that can cause damage to host cells or disrupt normal physiological functions. Examples include botulinum toxin and cholera toxin. For an organism to be a successful pathogen it must: arrive at the body surface of a potential host, enter the host’s body, evade the host’s defenses, reproduce inside the host, and infect a new host. 4. Prokaryotes Have Amazingly Diverse Metabolic Pathways Diversity of Metabolic Pathways: ○ Prokaryotes have evolved a vast array of metabolic pathways, allowing them to occupy a variety of ecological niches, from extreme environments (e.g., hot springs, deep-sea vents) to more common habitats. Anaerobic versus Aerobic Metabolism: ○ Aerobic Respiration: Involves the use of oxygen as the final electron acceptor in the electron transport chain. It is highly efficient and produces large amounts of ATP. ○ Anaerobic Respiration: Some prokaryotes can use alternative electron acceptors like nitrate (NO₃⁻), sulfate (SO₄²⁻), or carbon dioxide (CO₂) in the absence of oxygen, although less energy-efficient. ○ Fermentation: In the absence of oxygen, many bacteria switch to fermentation pathways, which produce ATP without oxygen, but at a much lower yield. Obligate anaerobes- oxygen sensitive organisms where oxygen is poisonous Facultative anaerobes- alternate between an anaerobic mode and aerobic mode Aerotolerant anaerobes- cannot conduct cellular respiration but they are not damaged by oxygen when it is present. Obligate aerobes- unable to survive for extended periods in the absence of oxygen. Metabolic Flexibility: Prokaryotes can switch between aerobic and anaerobic metabolic pathways depending on environmental conditions, making them incredibly adaptable. 5. Nutritional Categories of Prokaryotes Photoautotrophs: ○ These organisms use light energy to drive the synthesis of organic compounds from carbon dioxide (CO₂). They contain pigments like chlorophyll (e.g., cyanobacteria). ○ Example: Cyanobacteria, which also produce oxygen as a by-product of photosynthesis. Photoheterotrophs: ○ These organisms use light energy but require organic carbon compounds as their carbon source. They do not fix CO₂. ○ Example: Purple non-sulfur bacteria. Chemoautotrophs: ○ These organisms obtain energy by oxidizing inorganic compounds (e.g., hydrogen sulfide, ammonia, iron) and use CO₂ as their carbon source. ○ Example: Nitrifying bacteria, sulfur bacteria, and iron bacteria. Chemoheterotrophs: ○ These organisms obtain both energy and carbon by consuming organic compounds. Most pathogenic bacteria, as well as many decomposers, are chemoheterotrophs. ○ Example: Most bacteria that decompose organic matter in the environment. 6. Prokaryotes Play Important Roles in Element Cycling Nitrogen Cycle: ○ Prokaryotes are key players in the nitrogen cycle, converting nitrogen into different forms that can be utilized by plants and other organisms. ○ Nitrogen Fixation: Certain bacteria (e.g., Rhizobium) fix atmospheric nitrogen (N₂) into ammonia (NH₃), which is then used by plants. ○ Nitrification: Nitrifying bacteria convert ammonia into nitrites (NO₂⁻) and nitrates (NO₃⁻), which plants can also use. ○ Denitrification: Denitrifying bacteria reduce nitrates back into nitrogen gas (N₂), completing the nitrogen cycle. Carbon Cycle: ○ Prokaryotes play a major role in breaking down organic matter, releasing carbon in the form of CO₂ through respiration and decomposition. Sulfur and Phosphorus Cycles: ○ Sulfur bacteria participate in the sulfur cycle, converting hydrogen sulfide (H₂S) into sulfur or sulfate (SO₄²⁻), which can be utilized by plants. ○ Phosphorus is cycled through the environment by phosphate-solubilizing bacteria, which make phosphorus available to plants. Summary Prokaryotes are essential to life on Earth, not only for their roles in health and disease but also in their incredible metabolic diversity and their central involvement in biogeochemical cycles. They form complex communities (biofilms), have critical roles in host microbiomes, and exhibit diverse nutritional strategies. The few pathogenic bacteria are responsible for disease, but most prokaryotes contribute positively to ecosystems by cycling essential elements and sustaining life processes. Chapter Title: Viruses Have Multiple Evolutionary Origins 1. Many RNA Viruses Probably Represent Escaped Genomic Components of Cellular Life RNA Viruses and Their Origins: 1. RNA viruses are thought to have evolved from genetic material that "escaped" from cellular organisms. This theory is based on the fact that RNA can serve as both genetic material and functional molecules, much like certain mobile genetic elements (e.g., plasmids, transposons). 2. Some RNA viruses are likely remnants of ancient cellular life that became independent, adapting to a parasitic lifestyle by infecting host cells. Types of RNA Viruses: 1. RNA viruses are classified by their genome type, which dictates the method of replication within host cells. The four main categories are: 2. Negative-Sense Single-Stranded RNA Viruses (−ssRNA): These viruses carry RNA that is complementary to the messenger RNA (mRNA). The viral RNA must first be converted into positive-sense RNA by an RNA-dependent RNA polymerase before protein synthesis can begin. Examples: Influenza virus, Rabies virus, Ebola virus. The genome of these viruses typically encodes a polymerase enzyme that replicates the viral RNA. 3. Positive-Sense Single-Stranded RNA Viruses (+ssRNA): The RNA in these viruses serves directly as mRNA for protein synthesis in the host cell. The viral RNA can be immediately translated by the host ribosomes upon entry. Examples: Poliovirus, Zika virus, SARS-CoV-2. Some +ssRNA viruses also replicate their RNA genomes through a replication intermediate that is double-stranded RNA. 4. RNA Retroviruses: Retroviruses are unique in that they carry the enzyme reverse transcriptase, which converts their RNA genome into DNA after infection. The resulting DNA is integrated into the host genome, becoming a provirus. Examples: Human Immunodeficiency Virus (HIV), Human T-cell Leukemia Virus (HTLV). Retroviruses provide a possible link between viral and cellular genetic systems, as the process of reverse transcription allows them to integrate their genetic material into the host cell’s genome. 5. Double-Stranded RNA Viruses (dsRNA): These viruses have a double-stranded RNA genome, which typically has a segmented structure. The viral RNA-dependent RNA polymerase is used to transcribe the RNA into mRNA for translation. Examples: Rotavirus, Coltivirus. dsRNA viruses often have complex replication cycles involving both negative- and positive-sense RNA intermediates. 2. Some DNA Viruses May Have Evolved from Reduced Cellular Organisms DNA Virus Origins: 1. Some researchers suggest that certain DNA viruses could have evolved from reduced, parasitic cellular organisms, such as intracellular bacteria or archaea. These organisms might have lost many cellular functions over time, becoming simpler forms of life that depend entirely on a host for replication. 2. Evidence for this includes similarities between the genomes of certain DNA viruses and the genomes of certain cellular organisms, particularly in terms of gene sequences and protein functions. Types of DNA Viruses: 1. Double-Stranded DNA Viruses: These viruses have a double-stranded DNA genome, which is similar to the genomes of many cellular organisms. Their replication follows the typical DNA transcription and translation pathways, with the host cell's machinery involved in replication. Examples: Herpesviruses, Adenoviruses, Poxviruses. Some of these viruses have large genomes that encode for enzymes involved in their own replication, suggesting an evolutionary history linked to cellular life. 2. Single-Stranded DNA Viruses: These viruses have a single-stranded DNA genome, which must be converted into double-stranded DNA before it can be replicated and transcribed within the host. Examples: Parvoviruses, Circoviruses. The replication machinery for these viruses is often very minimal, indicating a simplified genome compared to complex cellular organisms, possibly pointing to an evolutionary reduction from a more complex ancestor. 3. Viruses Can Be Used to Fight Bacterial Infections Bacteriophages (Phages): ○ Bacteriophages are viruses that specifically infect and kill bacteria. They can be harnessed as a therapeutic tool to target bacterial infections, especially in cases where antibiotics are ineffective or resistance has developed. ○ Phages are highly specific to their bacterial hosts, making them a targeted approach for therapy without harming beneficial bacteria in the microbiome. Phage Therapy: ○ Phage therapy involves the use of bacteriophages to treat bacterial infections. This approach has been investigated as an alternative or supplement to traditional antibiotics. ○ Phages work by infecting bacteria and using the bacterial machinery to replicate, eventually causing the bacterial cell to lyse (break open), releasing new phages that can infect other bacterial cells. Advantages of Phage Therapy: ○ Specificity: Phages only infect certain bacteria, reducing the collateral damage to normal microbiota and preventing the broad-spectrum effects seen with antibiotics. ○ Evolutionary Pressure: Since phages target specific bacterial strains, they can evolve in response to bacterial resistance mechanisms, potentially offering a sustainable treatment strategy. ○ Reduced Antibiotic Resistance: By using phages to treat bacterial infections, the overuse of antibiotics can be reduced, decreasing the selection pressure for antibiotic-resistant strains. Challenges and Considerations: ○ Phage Resistance: Just as bacteria can develop resistance to antibiotics, they can also evolve mechanisms to resist phage infections, such as by modifying the receptors phages use to attach. ○ Regulatory Issues: The use of phages in medicine is still not as widespread as antibiotics due to regulatory challenges, the complexity of producing phage preparations, and the need for personalized treatments. ○ Phage Cocktail Development: To avoid resistance, phage therapies often use mixtures of different phages (called phage cocktails) targeting multiple bacterial strains.