Chapter 23: Protein Turnover and Amino Acid Catabolism PDF
Document Details
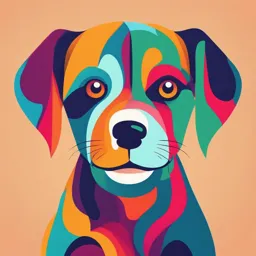
Uploaded by ReplaceableFir
Lakehead University
2019
Jeremy M. Berg, John L. Tymoczko, Gregory J. Gatto, Jr., Lubert Stryer
Tags
Related
- BIOCHEMISTRY AMINO ACIDS: NITROGEN DISPOSAL (Part 1) PDF
- BIOCHEMISTRY AMINO ACIDS: NITROGEN DISPOSAL (Part 1) PDF
- Lecture 14 Catabolism of Body Proteins PDF
- Amino Acid Metabolism and Urea Cycle PDF
- Harper's Biochemistry - Catabolism of Proteins & Amino Acid Nitrogen Chapter 28 PDF
- Protein and Amino Acid Metabolism Lecture Notes PDF
Summary
Chapter 23 from 'Biochemistry' Ninth Edition, focuses on protein turnover and amino acid catabolism. It covers topics such as fates of proteins and amino acids, essential and non-essential amino acids. The chapter also discusses metabolic integration of nitrogen, and specific disorders, for example, inherited defects of the urea cycle.
Full Transcript
Chapter 23 Protein Turnover and Amino Acid Catabolism © 2019 W. H. Freeman and Company CHAPTER 23 Protein Turnover and Amino Acid Catabolism 1 Ch.23 Learning Objectives By the end of this chapter, you should be able to: 1. E...
Chapter 23 Protein Turnover and Amino Acid Catabolism © 2019 W. H. Freeman and Company CHAPTER 23 Protein Turnover and Amino Acid Catabolism 1 Ch.23 Learning Objectives By the end of this chapter, you should be able to: 1. Explain the importance of the regulation of protein turnover. 2. Identify the role of ubiquitin and describe the enzymes required for ubiquitination. 3. Explain the function of the proteasome. 4. Describe the fate of nitrogen that is removed when amino acids are used as fuels. 5. Explain how the carbon skeletons of the amino acids are metabolized after nitrogen removal. 6. Identify metabolic errors in amino acid degradation. Van Nguyen 2 Ch.23 Outline 23.1 Proteins Are Degraded to Amino Acids 23.2 Protein Turnover Is Tightly Regulated 23.3 The First Step in Amino Acid Degradation Is the Removal of Nitrogen 23.4 Ammonium Ion Is Converted into Urea in Most Terrestrial Vertebrates 23.5 Carbon Atoms of Degraded Amino Acids Emerge as Major Metabolic Intermediates 23.6 Inborn Errors of Metabolism Can Disrupt Amino Acid Degradation Van Nguyen 3 Fates of Proteins and Amino Acids Amino acids are obtained from the diet when proteins are digested. In the cell, proteins are degraded to amino acids because of damage or for regulatory purposes. The first priority for use of amino acids is as precursors for proteins or other biomolecules. Amino acids are not stored, so any excess amino acids are degraded. The amino group is removed, processed in the urea cycle, and excreted as urea. The carbon skeletons are converted into metabolic intermediates. Van Nguyen 4 Fates of Proteins and Amino Acids Van Nguyen 5 Section 23.1 Proteins Are Degraded to Amino Acids Dietary proteins are degraded to amino acids, which are absorbed and distributed throughout the body via the blood. Essential amino acids cannot be synthesized by the body and must be obtained in the diet. Van Nguyen 6 Digestion and Absorption of Proteins stomach protease: pepsin,.. pancreatic proteases The amino acids are absorbed by transporters. Peptidases on the surface of intestinal cells cleave the oligopeptides into di- and tripeptides, which are transported into the intestinal cells by specific transporters and degraded to amino acids. The amino acids are subsequently released into the blood by transporters (red oval) for use by other tissues. Van Nguyen 7 Cellular Proteins Are Degraded at Different Rates Protein turnover, the degradation and resynthesis of proteins, is a constant cellular activity. Proteins can be damaged by errors in translation or by environmental factors. These proteins are selectively degraded. The half-lives of proteins vary from minutes to months to the life of the organism. Van Nguyen 8 Section 23.2 Protein Turnover Is Tightly Regulated Ubiquitin tags proteins for destruction. Ubiquitin is a small (76 aa), highly conserved protein. Multiple copies of ubiquitin, attached to proteins by an isopeptide bond, mark the proteins for degradation. Polyubiquitin is an especially effective destruction signal. Van Nguyen 9 3 Enzymes Required for Ubiquitin Conjugation Ubiquitin-activating enzyme (E1) adenylates Ub and transfers it to a cysteine residue on E1 Ubiquitin–protein ligase (E3), Ubiquitin-conjugating using the E2-Ub complex as a enzyme (E2) then substrate, transfers the Ub to the transfers Ub to one of its target protein own cysteine residues Van Nguyen 10 Structure of Tetraubiquitin 4 Ub are linked by isopeptide bonds (C=O of C terminus with the ε-H2N of a lysine residue). Dashed lines indicate the positions of the extended C- termini that were not observed in the crystal structure. This unit is the primary signal for degradation when linked to a target protein. Van Nguyen 11 Ubiquitin Tags Proteins for Destruction Specific amino acid sequences, termed degrons, determine the half-life of proteins. For many proteins, the N-terminal amino acid is an important degradation signal. Other degrons include cyclin destruction boxes and PEST sequences. Dependence of the Half-lives of In some cases, proteins must be Cytoplasmic Yeast Proteins on altered to expose the degrons. the Identity of their Amino- terminal Residues Van Nguyen 12 Importance of E3 Proteins to Normal Cell Function: Examples Proteins that are not broken down due to defective E3 enzyme may accumulate to create a disease of protein aggregation (e.g. juvenile or early-onset Parkinson disease). A defect in another member of the E3 family causes Angelman syndrome, a severe neurological disorder. Overexpression of this same ligase results in autism. Inappropriate protein turnover also can lead to cancer; inappropriate E3 activation can lead to ubiquitination and destruction of needed tumor suppressor proteins. Van Nguyen 13 The Proteasome Digests the Ubiquitin- tagged Proteins The proteasome is a large molecular complex of proteolytic enzymes that degrades ubiquitinated proteins. The 26S proteasome consists of two components: a 20S catalytic subunit and a 19S regulatory subunit. 26S Proteasome The regulatory subunit binds to polyubiquitin, cleaves off intact ubiquitin, unfolds the condemned protein, and inserts it into the catalytic subunit. 20S Proteasome Van Nguyen 14 The Ubiquitin Pathway and the Proteasome Have Prokaryotic Counterparts The ubiquitin–proteasome degradation process is found in all eukaryotes. Homologs of the proteasome are found in some prokaryotes. The archaeal proteasome consists of 14 identical α subunits and 14 identical β subunits. In the eukaryotic proteasome, gene duplication and specialization has led to 7 distinct subunits of Proteasome Evolution each type. The overall architecture of the proteasome is conserved. Van Nguyen 15 Protein Degradation Can Be Used to Regulate Biological Function Many biological processes are regulated by controlled, specific protein degradation. Bortezomib (Velcade) is used to treat multiple myeloma. It is a proteasome inhibitor, causing proteins build up and hence (cancer) cell dies. HT1171 is a suicide inhibitor of the proteasome of M. tuberculosis and shows promise as a treatment for tuberculosis. Van Nguyen 16 Processes Regulated by Protein Degradation Van Nguyen 17 The Proteasome and Other Proteases Generate Free Amino Acids Van Nguyen 18 Amino Acid Degradation Van Nguyen 19 Section 23.3 The First Step in Amino Acid Degradation Is the Removal of Nitrogen Aminotransferases (transaminases) transfer amino groups from an amino acid to α-ketoglutarate to generate glutamate. Glutamate dehydrogenase, a mitochondrial enzyme, releases NH4+ in the oxidative deamination of glutamate. Glutamate dehydrogenase Glutamate dehydrogenase is unusual in being able to use NAD+/NADH or NADP+/NADPH. Van Nguyen 20 Alpha-amino Groups Are Converted into Ammonium Ions by the Oxidative Deamination of Glutamate The coupled reactions of aminotransferases and glutamate dehydrogenase are In terrestrial vertebrates, the ultimate fate of the NH4+ is the formation of urea. Van Nguyen 21 Mechanism: Pyridoxal Phosphate PLP Aminotransferases require the coenzyme pyridoxal phosphate (PLP), a derivative of pyridoxine (vitamin B6): PLP exhibits tautomeric forms: Van Nguyen 22 Mechanism: Schiff-base Intermediates PLP forms a Schiff-base linkage with the ε-amino group at the enzyme active site. A new Schiff-base linkage occurs upon amino acid binding. An internal aldimine is replaced with an external aldimine. Van Nguyen 23 Transamination Mechanism The first half of the transamination reaction consist of three steps: 1. The external aldimine loses a proton to form a quinonoid intermediate. 2. Reprotonation yields a ketimine. 3. The ketimine is hydrolyzed to yield the α-ketoacid product and a substituted enzyme intermediate, pyridoxamine phosphate (PMP). Van Nguyen 24 Transamination Mechanism The second half of the reaction is the reverse of the first half. – First half: – Second half: The sum of these reactions is Van Nguyen 25 Blood Levels of Aminotransferases Serve a Diagnostic Function The presence of alanine aminotransferase (ALT) and aspartate aminotransferase (AST) in the blood indicates that liver damage has occurred. Viral hepatitis and alcohol abuse can damage liver cells such that cellular proteins, including the aminotransferases, leak into the blood. Van Nguyen 26 Aspartate Aminotransferase Is an Archetypal Pyridoxal-dependent Transaminase Mitochondrial aspartate aminotransferase functions as a dimer. PLP is bound to lysine 258 in a Schiff-base linkage. PLP is found in a pocket near the interface of the two subunits. An arginine residue in the active site helps orient substrates by binding to their α-carboxylate groups. Aspartate aminotransferase Only one of the enzyme’s two subunits is shown. 27 Pyridoxal Phosphate Enzymes Catalyze a Wide Array of Reactions PLP-dependent enzymes catalyze decarboxylations, deaminations, racemizations, and aldol cleavages at the α- carbon of amino acids. PLP-dependent enzymes also catalyze elimination and replacement reactions at the β- carbon and γ-carbon atom of amino acids. Common features of PLP catalysis: 1. A Schiff base is formed between a substrate amino group and PLP. 2. PLP is an electrophilic catalyst acting as an electron sink. 3. The product Schiff base is cleaved at the completion of the reaction. Van Nguyen 28 Bond Cleavage by PLP Enzymes Pyridoxal phosphate enzymes labilize one of three bonds at the α-carbon atom of an amino acid substrate. For example, bond a is labilized by aminotransferases, bond b by decarboxylases, and bond c by aldolases (such as threonine aldolases). PLP enzymes also catalyze reactions at the β- and γ-carbon atoms of amino acids. Van Nguyen 29 Stereoelectronic Effects The bond to be broken in the substrate must be perpendicular to the π orbital of the PLP electron sink. This means of choosing one of several catalytic results is called stereoelectronic control. The orientation about the N−Cα bond determines the most favored reaction catalyzed by a pyridoxal phosphate enzyme. The bond that is most nearly perpendicular to the plane of delocalized π orbitals (represented by dashed lines) of the pyridoxal phosphate electron sink is most easily cleaved. Van Nguyen 30 Reaction Choice In aspartate aminotransferase, the Cα−H bond is most nearly perpendicular to the π-orbital system and is cleaved. In serine hydroxymethyltransferase, a small rotation about the N−Cα bond places the Cα−Cβ bond perpendicular to the π system, favoring its cleavage. Van Nguyen 31 Serine and Threonine Can Be Directly Deaminated Serine dehydratase and threonine dehydratase directly deaminate their respective amino acids. Dehydration precedes deamination. Van Nguyen 32 Peripheral Tissues Transport Nitrogen to the Liver Muscle uses branched-chain amino acids as fuel. The nitrogen from these amino acids is transported to the liver by the glucose–alanine cycle. Nitrogen can also be transported as glutamine formed from glutamate by glutamine synthetase. Van Nguyen 33 Pathway Integration: The Glucose–alanine Cycle During prolonged exercise and fasting, muscle uses branched-chain amino acids as fuel. The removed nitrogen is transferred (through glutamate) to alanine, which is released into the bloodstream. In the liver, alanine is taken up and converted into pyruvate for the subsequent synthesis of glucose. 34 Section 23.4 Ammonium Ion to Urea Ammonium Ion Is Converted The Urea Cycle into Urea in Most Terrestrial Vertebrates Organisms that excrete excess NH4+ as urea are called ureotelic organisms. In humans, the urea cycle occurs in the liver. Van Nguyen 35 The Urea Cycle Begins with the Formation of Carbamoyl Phosphate The first step in the urea cycle is the coupling of ammonia with bicarbonate This reaction, which occurs in the mitochondria, is catalyzed by carbamoyl phosphate synthetase I. Van Nguyen 36 Carbamoyl Phosphate Synthetase Is the Key Regulatory Enzyme for Urea Synthesis Carbamoyl phosphate synthetase requires N-acetylglutamate (NAG) for activity, which is synthesized by N-acetylglutamate synthase. The synthase is activated by arginine. Thus, NAG is synthesized when amino acids are abundant. Van Nguyen 37 Carbamoyl Phosphate Reacts with Ornithine to Begin the Urea Cycle The carbamoyl group is transferred to ornithine by ornithine transcarbamoylase to form citrulline. Citrulline is transported out of the mitochondria into the cytoplasm in exchange for ornithine. Van Nguyen 38 Carbamoyl Phosphate Reacts with Ornithine to Begin the Urea Cycle In the cytoplasm, citrulline condenses with aspartate, the donor of the second nitrogen of urea, to form argininosuccinate. This reaction is catalyzed by argininosuccinate synthetase. Van Nguyen 39 Carbamoyl Phosphate Reacts with Ornithine to Begin the Urea Cycle Argininosuccinate is cleaved into arginine and fumarate by argininosuccinase. Van Nguyen 40 Carbamoyl Phosphate Reacts with Ornithine to Begin the Urea Cycle Arginine is cleaved by arginase into urea, which is excreted, and ornithine, which is transported into the mitochondria. Van Nguyen 41 The Urea Cycle Is Linked to Gluconeogenesis The stoichiometry of the urea cycle is Fumarate can be converted into oxaloacetate by the citric acid cycle and then into glucose by the gluconeogenic pathway. Van Nguyen 42 Metabolic Integration of Nitrogen Metabolism The urea cycle, gluconeogenesis, and the transamination of oxaloacetate are linked by fumarate and aspartate. Van Nguyen 43 Urea-cycle Enzymes Are Evolutionarily Related to Enzymes in Other Metabolic Pathways An isozyme of carbamoyl phosphate synthetase (CPS II) is used in pyrimidine synthesis. In this enzyme, NH3 is supplied by the hydrolysis of glutamine. – The glutamine binding site in CPS I has evolved into an allosteric site for binding N-acetylglutamate. Also, ornithine transcarbamoylase is homologous to aspartate transcarbamoylase, which catalyzes the first step in pyrimidine biosynthesis, and the structures of their catalytic subunits are quite similar. This shows that two consecutive steps in the pyrimidine biosynthetic pathway were adapted for urea synthesis. Van Nguyen 44 Inherited Defects of the Urea Cycle Cause Hyperammonemia and Can Lead to Brain Damage All defects in the urea cycle lead to elevated levels of NH4+ in the blood (hyperammonemia). Argininosuccinase deficiency is treated by supplementing the diet with arginine. Excess nitrogen is excreted in the form of argininosuccinate. Carbamoyl phosphate synthetase deficiency and ornithine transcarbamoylase deficiency lead to the accumulation of nitrogen in glycine and glutamine. Addition of benzoate to the diet leads to the excretion of glycine-nitrogen as hippurate, while the addition of phenylacetate results in the excretion of phenylacetylglutamine. Van Nguyen 45 Treatment of Argininosuccinase Deficiency Argininosuccinase deficiency can be managed by supplementing the diet with arginine. Nitrogen is excreted in the form of argininosuccinate. Van Nguyen 46 Treatment of Carbamoyl Phosphate Synthetase and Ornithine Transcarbamoylase Deficiencies Both deficiencies can be treated by supplementing the diet with benzoate and phenylacetate. Nitrogen is excreted in the form of hippurate and phenylacetylglutamine. 47 Urea Is Not the Only Means of Disposing of Excess Nitrogen While ureotelic organisms excrete excess nitrogen as urea, ammoniotelic organisms, such as aquatic animals, simply excrete NH4+. Uricotelic organisms, such as birds, secrete excess nitrogen as uric acid, a purine. Van Nguyen 48 Section 23.5 Carbon Atoms of Degraded Amino Acids Emerge as Major Metabolic Intermediates The carbon skeletons of the amino acids are metabolized to seven major metabolic intermediates: pyruvate, acetyl CoA, acetoacetyl CoA, α-ketoglutarate, succinyl CoA, fumarate, and oxaloacetate. Amino acids metabolized to acetyl CoA and acetoacetyl CoA are called ketogenic amino acids because they can form fats but not glucose. Amino acids degraded to the remaining major intermediates are called glucogenic amino acids because they can be used to synthesize glucose. Only leucine and lysine are solely ketogenic. Van Nguyen 49 Fates of the Carbon Skeletons of Amino Acids Glucogenic amino acids (red), ketogenic amino acids (yellow). Several amino acids are both glucogenic and ketogenic. Van Nguyen 50 Pyruvate is an Entry Point into Metabolism for a Number of Amino Acids Alanine is converted into pyruvate by the action of alanine aminotransferase. Glutamate is subsequently oxidatively deaminated. The sum of these reactions is Serine is easily converted into pyruvate by the action of serine dehydratase. Other amino acids require more complicated pathways to form pyruvate. Van Nguyen 51 Pyruvate Formation from Amino Acids Pyruvate is the point of entry for alanine, serine, cysteine, glycine, threonine, and tryptophan. Van Nguyen 52 Oxaloacetate Is an Entry Point into Metabolism for Aspartate and Asparagine Aspartate is converted into oxaloacetate by a transamination reaction. Asparaginase hydrolyzes asparagine to NH4+ and aspartate, which is converted into oxaloacetate. Van Nguyen 53 Alpha-ketoglutarate Is an Entry Point into Metabolism for Five-carbon Amino Acids Glutamate is deaminated to form α-ketoglutarate. Histidine is converted into α-ketoglutarate in a reaction sequence that requires the coenzyme tetrahydrofolate. Glutamine is hydrolyzed by glutaminase to form glutamate. Proline and arginine are converted into glutamate γ- semialdehyde and then to glutamate. Van Nguyen 54 Histidine Degradation Proline and Arginine Degradation Van Nguyen 55 Succinyl Coenzyme A Is a Point of Entry for Several Amino Acids Methionine, leucine, and valine are converted into propionyl CoA, which is metabolized to succinyl CoA in a vitamin B12-dependent reaction. Van Nguyen 56 Methionine Metabolism Methionine is converted into succinyl CoA in nine steps. S-Adenosylmethionine, a common methyl donor, is an intermediate in the metabolism of methionine. Van Nguyen 57 Threonine Deaminase Initiates the Degradation of Threonine Threonine can be degraded by several different pathways. One involves the conversion to α-ketobutyrate and then propionyl CoA. Van Nguyen 58 The Branched-chain Amino Acids Yield Acetyl CoA, Acetoacetate, or Propionyl CoA The branched-chain amino acids—leucine, isoleucine, and valine—are converted into acetyl CoA and acetoacetyl CoA using reactions similar to those of the citric acid cycle and fatty acid oxidation. The branched-chain α-ketoacid dehydrogenase complex, which is similar to the pyruvate dehydrogenase complex and the α-ketoglutarate dehydrogenase complex, processes these amino acids to form isovaleryl CoA. Van Nguyen 59 The Branched-chain Amino Acids Yield Acetyl CoA, Acetoacetate, or Propionyl CoA Van Nguyen 60 Oxygenases Are Required for the Degradation of Aromatic Amino Acids The aromatic amino acids require monooxygenases (mixed- function oxygenases) for degradation. Monooxygenases use O2 as a substrate and incorporate one oxygen atom into the product and one into water. The monooxygenase phenylalanine hydroxylase converts phenylalanine into tyrosine with the assistance of the cofactor tetrahydrobiopterin. Van Nguyen 61 Oxygenases Are Required for the Degradation of Aromatic Amino Acids The reductant for the phenylalanine hydroxylase catalyzed reaction, tetrahydrobiopterin, is regenerated by the action of dihydropteridine reductase. Van Nguyen 62 Oxygenases Are Required for the Degradation of Aromatic Amino Acids Tetrahydrobiopterin is regenerated with NADPH. The sum of the reactions for the conversion of phenylalanine into tyrosine and the regeneration of tetrahydrobiopterin is Tyrosine is metabolized to fumarate and acetoacetate. Tryptophan degradation requires both monooxygenases and dioxygenases to metabolize the amino acid to acetoacetate. Dioxygenases, which incorporate both atoms of O2 into the product, are used to cleave aromatic rings. Van Nguyen 63 Phenylalanine and Tyrosine Degradation Van Nguyen 64 Tryptophan Degradation Van Nguyen 65 Protein Metabolism Helps to Power the Flight of Migratory Birds While fats are the main fuel for migratory birds, proteins are also degraded during their long flights for several reasons: 1. Since the birds are not feeding, proteins involved with digestion and transport of nutrients from the gut can be degraded. 2. Glucogenic amino acids can be used to provide building blocks for gluconeogenesis, providing glucose for the central nervous system. Also, some amino acids will be converted to citric acid cycle building blocks to facilitate fat metabolism. 3. Protein degradation will create a lighter body mass, which will reduce the energy cost of the flight. 4. The organism does not stop to hydrate during the flight, and protein catabolism provides much more water than does fat catabolism. Van Nguyen 66 Section 23.6 Inborn Errors of Metabolism Can Disrupt Amino Acid Degradation Alcaptonuria is a disease that results from the absence of homogentisate oxidase, an enzyme in the tryptophan degradation pathway. Homogentisate accumulates and, upon excretion, turns the urine dark. Van Nguyen 67 Inborn Errors of Metabolism Can Disrupt Amino Acid Degradation Maple syrup urine disease results when the oxidative decarboxylation of α-ketoacids derived from branched-chain amino acids is blocked due to lack of activity of the dehydrogenase complex. The disease can be detected by screening newborn urine with 2,4- dinitrophenylhydrazine, which reacts with α- ketoacids to form 2,4- dinitrophenylhydrazone derivatives. 68 Inborn Errors of Amino Acid Metabolism Van Nguyen 69 Phenylketonuria Is One of the Most Common Metabolic Disorders Phenylketonuria results if phenylalanine hydroxylase activity is missing or deficient. Excess phenylalanine is converted into phenylpyruvate. Untreated phenylketonurics show severely impaired mental ability. Van Nguyen 70 Determining the Basis of the Neurological Symptoms of Phenylketonuria is an Active Area of Research The bases for the clinical features of phenylketonuria are still obscure. Possibilities include a lack of tyrosine, a precursor for certain neurotransmitters. Moreover, the high concentration of phenylalanine in the blood may inhibit transport of any tyrosine present, as well as tryptophan, into the brain. It is also believed that high brain phenylalanine concentration inhibits glycolysis, disrupts myelination of nerve fibers, and disrupts synthesis of several neurotransmitters. Van Nguyen 71 Methylmalonic Acidemia Results from an Inborn Error of Metabolism In methylmalonic acidemia, the blood concentration of methylmalonic acid can be dangerously high, with affected infants experiencing vomiting, muscle weakness, and possible fatality. Methylmalonic acid is an intermediate when propionyl CoA is converted into succinyl CoA, and there are two main reasons for doing so: either in the metabolism of odd-chain fatty acids or in the metabolism of four of the essential fatty acids (methionine and the branched-chain fatty acids leucine, isoleucine, and valine). Van Nguyen 72 Methylmalonic Acidemia Results from an Inborn Error of Metabolism Treatment for methylmalonic acidemia is a diet that is low in those four amino acids, with just enough to support protein synthesis. There are two possible causes of the metabolic problem: either the enzyme (methylmalonyl CoA mutase) is missing or nonfunctional or the supply of the vitamin B12 needed by this enzyme is inadequate. In the latter case, vitamin supplementation rather than amino acid restriction is prescribed. 73