Cell Biology Past Paper PDF Fall 2024
Document Details
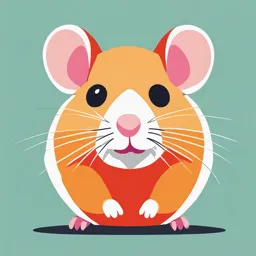
Uploaded by ConsistentEuphonium
Lincoln High School
2024
Tags
Summary
This document is a chapter on stem cells in tissue homeostasis and regeneration. The chapter discusses asymmetric and symmetric stem-cell divisions, Drosophila germ-line stem cells, stem cell aging and methods to distinguish the contribution of internal environmental factors to declines in function, parabiosis, and regeneration.
Full Transcript
BIOS 367 Cell Biology Fall 2024 Chapter 22 – Part 2 Stem Cells in Tissue Homeostasis and Regeneration Asymmetric and symmetric stem-cell divisions In the asymmetric stem-cell division schematized here, a cell-fate determinant (red) that mai...
BIOS 367 Cell Biology Fall 2024 Chapter 22 – Part 2 Stem Cells in Tissue Homeostasis and Regeneration Asymmetric and symmetric stem-cell divisions In the asymmetric stem-cell division schematized here, a cell-fate determinant (red) that maintains stem-cell identity is localized to the cell cortex at one side of the cell. When the cell divides, only one daughter cell inherits the determinant and remains a stem cell, while the other daughter commits to differentiation In the symmetric stem-cell division shown, both daughter cells inherit such determinants and remain stem cells. How the plane of division in a dividing, germ-line stem cell in the Drosophila testis determines which daughter cell maintains contact with the niche. The independent-choice mechanism for how the two daughters of a stem-cell division choose their fates The choice to differentiate or remain a stem cell might be determined by the local environment a daughter finds itself in In other cases, the choice to differentiate or remain a stem cell is made stochastically (randomly) With a choice made randomly by each daughter there is, for example, a 25% chance at the first division that both daughters will commit to differentiation, so that the clone will eventually disappear. Or, at this division or later, a preponderance of daughters might choose to remain stem cells, creating a clone that persists and increases in size. If this choice is not actually random, but influenced by a changing environment, this strategy can adjust to changing conditions Stem cells and aging Do stem cells get old? Serial transplantation of hematopoetic stem cells show that, as the stem cells get older, they gradually lose their ability to repopulate the hematopoetic system of the new hosts. Methods to distinguish the contribution of cell-autonomous versus (internal) environmental factors to declines in hematopoietic stem- cell function during aging Results indicate that a young stem cell niche cannot rescue old hematopoetic stem cells and an old stem cell niche can support young hematopoetic stem cells. So in this case, the age of the cells is more important In parabiosis, animals are joined to develop a shared circulatory system. Cells in one animal will be exposed to the systemic environment (blood) of the other animal This comparison provides a way of determining the contributions of different factors to a decline in stem cell functioning with age: the stem cells themselves, and circulating factors in the blood. Blood from old mice can decrease neurogenesis in the hippocampus of young mice Blood from young mice can restore skeletal muscle stem cell function in old mice, and can counteract age related dysfunction in liver, pancreas, bone, and heart Regeneration Some animals are much better at regenerating than humans are The planarian worm, Schmidtea mediterranea contains several tissue types Regeneration Some animals are much better at regenerating than humans are https://futurism.com/neoscope/planarians-regeneration-medicine-future Regeneration of a planarian from a single somatic cell. About 20% of the cells in a planarian are small, undifferentiated dividing cells called neoblasts which serve as stem cells. At least some of them are totipotent, able to give rise to all cell types (A) The distribution of dividing cells (neoblasts; blue) in the adult body Newt limb regeneration Most of the signaling pathways involved in limb regeneration are the same as those used in limb development. Why mammals are unable to do this is unknown. (humans can sometimes regenerate fingertips though!) The continuing production of neurons in an adult mouse brain The brain is viewed from above, in a cutaway section, to show the region lining the ventricles of the forebrain where neural stem cells are found. These cells continually produce progeny that migrate to the olfactory bulb, where they terminally differentiate into neurons Olfactory neurons are unusual in that they are continuously replaced In adult mice and humans, there is also a continuing turnover of neurons (about 1.75% per year) in the hippocampus (not shown), a region specially concerned with learning and memory A more dramatic neuronal turnover is observed in the brains of certain songbirds. In these birds, large numbers of neurons die each year and are replaced by new neurons, as part of a process by which the birds refine their song for each new breeding season. Neural stem cells. Shown are the steps leading from mammalian fetal brain tissue or embryonic stem cells, via neurospheres (A), to a pure culture of neural stem cells (B). These stem cells can be kept proliferating as such indefinitely or, through a change of medium, can be caused to differentiate (C) into neurons (red) and glial cells (green) Neural stem cells. Neural stem cells can be grafted into an adult brain. Once there, they show a remarkable ability to adjust their behavior to match their new location. Stem cells from the mouse hippocampus, for example, when implanted into the migration pathway of the mouse olfactory-bulb precursor cells, give rise to neurons that become correctly incorporated into the olfactory bulb. This capacity of neural stem cells and their progeny to adapt to a new environment in the mammalian brain suggests applications in the treatment for central nervous system diseases or injury. Several clinical trials have used stem cells to treat stroke damage. The results are mixed Production and pluripotency of ES cells. The cultured ES cells can be taken at any time—after genetic manipulation, if desired—and injected back into a developing blastocyst. There they incorporate into the inner cell mass and take part in formation of a chimeric animal that is a mixture of ordinary and ES-derived cells The ES-derived cells can differentiate into any of the cell types in the body, including germ cells from which a new generation of mice can be produced, which are no longer chimeric, but consist of cells that all inherit half their genes from the cultured ES cell line. Reprogramming fibroblasts to iPS (induced Pluripotent Stem) cells with the OSKM factors The transcription regulatory proteins Oct4, Sox2, Myc, and Klf4 (OSK factors) induce both their own and each other’s synthesis (gray shading). This generates a self-sustaining feedback loop that helps to maintain cells in an ES cell–like state Forced expression of these 4 genes in mouse fibroblasts converts a small %age into Pluripotent stem cells which can be incorporated into a blastocyst to make a chimeric mouse, as in the previous slide. A strategy used to select cells that have converted into iPS cells This promoter is only active in embryonic stem cells G418 is an antibiotic that will kill eukaryotic cells. By introducing a G418 resistance gene under control of a promoter that is only active in embryonic stem cells, researchers can select for successful conversion to iPS cells. A summary of some of the major events that accompany the reprogramming of fibroblasts to iPS cells. Notably, the vast majority of fibroblasts expressing the OSKM factors fail to down- regulate somatic markers and fail to activate pluripotency genes and so do not convert to iPS cells Factors that have been observed to enhance efficiency of converting fibroblasts to iPS cells Many factors that improve conversion efficiency can alter chromatin states, with those in the top three rows having the most direct effects. ES and iPS cells can be guided to generate specific adult cell types and organoids If they are introduced to a later stage embryo or into adult tissue, they fail to receive the appropriate sequence of cues, and their differentiation is not properly controlled, often giving rise to a tumor called a teratoma (a tumor made of multiple tissues), containing a mixture of cell types that do not match the site in the body Production of differentiated cells from mouse or human ES or iPS cells in culture Cultured stem cells can be detached and allowed to form aggregates called embryoid bodies, which causes the cells to begin to specialize. Cells from embryoid bodies, cultured in media with different factors added, can then be driven to differentiate in various ways Cultured human ES cells can give rise to a three-dimensional organoid. Mouse or human pluripotent stem cells and their progeny in culture can proliferate, differentiate, and self-assemble into a three-dimensional eye-like structure (an optic cup), which includes a multilayered retina similar in organization to the one that forms during normal eye development in vivo Cultured human ES cells can give rise to a three-dimensional organoid. Fluorescence micrograph of an optic cup formed by human ES cells in culture. The structure includes a developing retina containing multiple layers of neural cells (stained green) and an underlying layer of pigmented epithelium, the apical surface of which is stained red. All nuclei are stained blue The left side of the diagram shows how differentiated cells that are generated from iPS cells derived from an individual with a genetic disease can be used for analysis of the disease mechanism and for discovery of therapeutic drugs. The right side of the diagram shows how the genetic defect might be repaired in the iPS cells, which could then be induced to differentiate in an appropriate way and grafted back into the individual without danger of immune rejection.