CHAPTER-2 hydrometeorology.pptx
Document Details
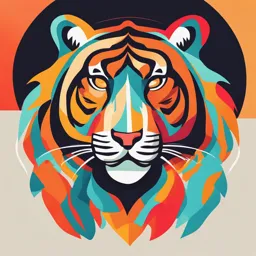
Uploaded by IlluminatingTantalum2662
Western Philippines University
Tags
Full Transcript
2. Weather and Hydrology 2.1 Solar and earth 2.2 Heat balance at earth’s surface 2.3 General atmospheric circulation 2.4 Temperature, humidity and wind 2.5 Patterns affecting Philippine weather and climate 2.1 Solar and earth The heat source for our planet is the su...
2. Weather and Hydrology 2.1 Solar and earth 2.2 Heat balance at earth’s surface 2.3 General atmospheric circulation 2.4 Temperature, humidity and wind 2.5 Patterns affecting Philippine weather and climate 2.1 Solar and earth The heat source for our planet is the sun. Energy from the sun is transferred through space to earth's atmosphere to the earth's surface Since this energy warms the earth's surface and atmosphere, some of it is or becomes heat energy. There are three ways heat is transferred into and through the atmosphere: radiation conduction convection RADIATION is the transfer of heat energy through space by electromagnetic radiation. Most of the ER that comes to the earth from the sun is invisible. Only a small portion comes as visible light. Light is made of waves of different frequencies. Our brains interpret these different frequencies into colors(rainbow).Waves from the sun are infrared, which have lower frequencies than red, and ultraviolet, which have higher frequencies than violet light. It is infrared radiation that produces the warm feeling on our bodies. Most of the SR is absorbed by the atmosphere and much of what reaches the earth's surface is radiated back into the atmosphere to become heat energy. CONDUCTION is the transfer of heat energy from one substance to another or within a substance(molecule to molecule or from atom to atom). It is a very effective method of heat transfer in metals. However, air conducts heat poorly. CONVECTION is the transfer of heat energy in a fluid. This type of heating is most commonly seen in the kitchen with a boiling liquid. Air in the atmosphere, acts as a fluid. The sun's radiation strikes the ground, thus warming the rocks. As the rock's temperature rises due to conduction, heat energy is released into the atmosphere, forming a bubble of air which is warmer than the surrounding air. This bubble of air rises into the atmosphere. As it rises, the bubble cools with the heat contained in the bubble moving into the atmosphere. 2.2 Heat balance at Earth’s surface Earth's energy budget refers to the tracking of how much energy is flowing into and out of the Earth's climate, where the energy is going, and if the energy coming in balances with the energy going out. Understanding the Earth's energy budget can help to predict future effects of global warming, and to understand the various flows of energy on the Earth. Additionally, knowing how Earth's energy budget balances can provide insight into how the energy from the Sun interacts with the atmosphere. For example, this is important when examining the effects of greenhouse gases in the atmosphere— to ensure conditions on Earth are habitable. Earth's Energy Balance The two major components that must be investigated to determine if the Earth's energy budget balances is the incoming energy from the Sun and the outgoing infrared radiation from the Earth and its atmosphere. Solar energy to the Earth The Solar energy to the Earth refers to this energy that hits the surface of the Earth itself. The amount of energy that reaches the Earth provides a useful understanding of the energy for the Earth as a system. This energy goes towards weather, keeping the temperature of the Earth at a suitable level for life, and powers the entire biosphere. Additionally, this solar energy can be used for solar power either with solar thermal power plants or photovoltaic cells Calculating Solar Energy to Surface Energy from Sun to Earth The Sun is generally considered to produce a constant amount of power with a surface intensity of 6.33×107 W/m2. As the Sun's rays spread into space this radiation becomes less and less intense as an inverse square law. The average radiation intensity that hits the edge of the Earth's atmosphere is known as the solar constant, Isc. About 7% between January 4th (perihelion), when the Earth is closest to the sun, and July 4th (aphelion), when the Earth is furthest away. Therefore a yearly average is used and is determined to be 1367 W/m2 To determine this value from solar flux, the distance from the Earth to the Sun is used. As well, the total solar flux must be determined. Then the total solar flux from the Sun is divided by the surface area of a sphere that has a radius equal to the distance from the Earth to the Sun. This accounts for the "spreading" of the solar energy. The expression to determine this value is: To determine the average amount of solar energy that reaches the Earth, we must consider what the Earth "looks like" to the Sun. When looking at Earth from the Sun, only one half of the Earth can be seen. Thus to make an appropriate estimate of the average amount of solar energy over the entire surface area of the Earth the value for Isc must be divided by 2. In addition to this, the total solar irradiance is the maximum power delivered to a surface assuming that the path of incoming light is perpendicular to that surface. Due to the fact that the Earth is a sphere, only places near the equator come close to this perpendicular angle. At all other locations on the Earth, incoming sunlight is at some angle. With this decreasing angle, the average solar irradiance decreases as well. To account for this, the solar irradiance level is again halved, leaving a final average solar irradiance of approximately Isc=340W/m2. This value, which represents the average solar irradiance hitting Earth's outer atmosphere, does not yet account for losses due to reflection and absorption, which is discussed in the following section. Energy Absorbed by the Earth Notall of the solar energy that reaches the Earth's atmosphere is absorbed by the Earth. This is due to something known as the Earth's energy budget. This budget accounts for the fact that some of the energy incident on the outer atmosphere of the planet is immediately reflected back into space. Due to reflection by the atmosphere, clouds, and Earth's surface we can approximate that 70% of solar energy incident on the edge of the Earth's atmosphere is actually absorbed by the Earth. Taking this into account, the actual average amount of solar energy absorbed by the Earth amounts to: Energy to the Surface Energy that is absorbed by the Earth is not the same as the energy incident on the Earth's surface. On a perfectly clear or cloudless day, when the Sun is directly overhead (or at the "zenith"), solar irradiation is still reduced due to absorption (16%) and reflection (6%) by particles in Earth's atmosphere. These particles include carbon dioxide (CO2), Oxygen (O2), Ozone (O3) and water vapor (H2O). Air Mass is used to define the solar spectrum that is incident at various altitudes and conditions on Earth. Air Mass 0, or AM0 spectrum is the solar radiation outside the atmosphere and represents a power density of 1367W/m2. AM1.5 is regarded as "normal" air mass and represents a power density of 1000W/m2. The quantity of 1000W/m2 is defined as a unit of radiation, called "one sun", and is commonly used as a standard to test and rate photovoltaic cells and solar panels. This means that a solar panel rated at 250 watts will output this rated power when exposed to a solar power density of 1000W/m2. o The energy that does pass through is absorbed by the atmosphere or the surface, and then moves around through convection, evaporation, or in the form of latent heat. o Finally, when the energy exits the Earth it can do so by emission from the surface of the Earth, by clouds, or by the atmosphere. o Some of the energy that is radiated by the surface of the Earth is absorbed by clouds and greenhouse gases in the atmosphere and then re-emitted downwards, which is how the surface of the Earth is heated and kept at a habitable temperature. o This process of heating is known as the greenhouse effect. Overall, the energy that exits the Earth in different forms, when added together is equal to the energy that is absorbed by different parts of the Earth. Earth's Energy Imbalance The incoming energy to the Earth and the outgoing energy from the Earth do not actually balance. This imbalance is partially caused by the incoming energy from the Sun—which varies with the seasons and changes in the composition of the Earth's atmosphere. Changes in the composition of Earth's atmosphere alters the quantity of energy absorbed and reflected by the atmosphere. Changing factors such as this, result in a very small, but significant energy imbalance on the Earth. As human activities increase the amount of carbon dioxide in the atmosphere, the energy imbalance continues to grow. Today, the energy imbalance amounts to approximately. This means that more energy is coming in (and being absorbed) than is leaving the Earth. Compared to flow values in the hundreds of watts per meter squared, this imbalance seems negligible. However, to account for this imbalance, the Earth's temperature will increase in response. As well, since the amounts of carbon dioxide and other greenhouse gases in our atmosphere are increasing, this value is projected to increase at a rate of per decade, contributing even more to increasing temperatures. It is this imbalance in the energy budget that results in increasing temperatures on the Earth, one of the most significant effects of climate change. 2.3 General atmospheric circulation Winds circulate around the globe because of the rotation of the earth and the energy from the sun. The general direction of the winds varies around the globe depending on factors like latitude and proximity to oceans. The direction of the wind at various levels in the atmosphere determines the local climate and steers around weather systems and severe weather. The circulation of wind in the atmosphere is driven by the rotation of the earth and the incoming energy from the sun. Wind circulates in each hemisphere in three distinct cells which help transport energy and heat from the equator to the poles. The winds are driven by the energy from the sun at the surface as warm air rises and colder air sinks. The circulation cell closest to the equator is called the Hadley cell. o Winds are light at the equator because of the weak horizontal pressure gradients located there. The warm surface conditions result in locally low pressure. o The warm air rises at the equator producing clouds and causing instability in the atmosphere. This instability causes thunderstorms to develop and release large amounts of latent heat. o Latent heat is just energy released by the storms due to changes from water vapor to liquid water droplets as the vapor condenses in the clouds, causing the surrounding air to become more warm and moist, which essentially provides the energy to drive the Hadley cell. o The Hadley Cell encompasses latitudes from the equator to about 30°. o At this latitude surface high pressure causes the air near the ground to diverge. This forces air to come down from aloft to "fill in" for the air that is diverging away from the surface high pressure. o The air flowing northward from the equator high up in the atmosphere is warm and moist compared to the air nearer the poles. This causes a strong temperature gradient between the two different air masses and a jet stream results. o At the 30° latitudes, this jet is known as the subtropical jet stream which flows from west to east in both the Northern and Southern Hemispheres. o Clear skies generally prevail throughout the surface high pressure, which is where many of the deserts are located in the world. 2.4 Temperature, humidity and wind 2.3 TEMPERATURE OF THE ATMOSPHERE ► The continuous flow of solar radiation from the sun into the earth’s surface causes its temperature to rise. Temperature is the relative hotness or coldness of a body. It is also technically defined as the measure of the average energy of molecular motion. When the earth’s surface is already heated, this in turn heats the atmosphere. ► Temperature is a fundamental weather element. In response to irregular disposal of the sun’s energy (insolation), the air temperatures show wide variations. These variations in turn cause other significant weather changes. 2.4 Temperature, humidity and wind ATMOSPHERIC PRESSURE The Standard Atmosphere Gas molecules exert forces on each other and their environment as they collide. The magnitude of these forces depend upon the temperature of the gas and the number of molecules involved. These collision forces are expressed in terms of quantity called pressure. Pressure differences are principally related to temperature differences and to the number of molecules exerting pressure forces. Atmospheric motion results from pressure variations. The atmospheric pressure on a given surface is the force exerted by an overlying column of air extending to the outer limit of the atmosphere per unit area. Traditionally, air pressure is expressed in units of length, based on long use of the mercurial barometer in which fluctuations of the height of the mercury column are functions of changing pressure in the atmosphere. To meet the need for a pressure unit involving force rather than length, the millibar came into meteorological use and stems from the bar, which is the conventional engineering unit of pressure. The pressure exerted by the entire atmosphere on one square centimeter is approximately one bar, defined as a force of one million dynes per square centimeter. However, the standard atmosphere, with a temperature of 15 deg C and a latitude of 45 degrees, has a normal pressure of 1,013.2 millibars. This is equivalent to 29.92 inches or 760 mm of mercury. Thus, 1 in. Hg is equivalent to 33.86 millibars or 25.40 mm Hg. 2.4 Temperature, humidity and wind Humidity is a measure of the amount of moisture in the air. It tells you how comfortable it is to be outside, and if there is enough moisture to create clouds and rain. Humidity has a large impact on human and animal health and the health of crops. It affects the ability of both plants and animals to cool themselves through evaporation. It is also important to precipitation formation. Humidity tells you the moisture content of the atmosphere, or how much water vapor there is in the air. When the humidity is high it feels oppressive outside because sweat doesn't evaporate and provide cooling. When the humidity is low, you feel cooler but your skin dries out and you get dehydrated more easily because more moisture is being evaporated from your body. The same thing applies to crops in high and low humidity. High water vapor content is necessary to produce clouds and precipitation. Relative humidity and dew point are the two main ways to describe humidity. Relative humidity is given as a percentage and tells you how close the air is to being saturated. If the relative humidity is 100%, the air is saturated. If the relative humidity is 50%, the air contains half the water vapor required for it to be saturated. If the amount of water vapor in the air increases, the relative humidity increases, and vice versa. However, relative humidity is dependent on air temperature, too. If the water vapor content stays the same and the temperature drops, the relative humidity increases. If the water vapor content stays the same and the temperature rises, the relative humidity decreases. This is because colder air doesn’t require as much moisture to become saturated as warmer air. If you pay attention to the weather in the summer, you’ll notice that the relative humidity is actually higher in the morning than in the afternoon. This is because the cooler morning air is closer to saturation than the hot afternoon air, even with the same amount of water vapor. Surprisingly, there is no significant difference in daily average relative humidity between summer and winter. Since warm air is less dense than cold air, there is more room for water vapor in warm summer air as compared with cold winter air. Dew point is a better indicator of humidity than relative humidity because it is not a percentage dependent on temperature. The dew point is the temperature to which the air would have to be cooled to become saturated. Below the dew point, water will condense out of the air onto surfaces. In the early morning, grass surfaces will be coated with water if the night time temperature has dropped below the dew point. When humidity is high, the dew point temperature is only a few degrees below, or equal to, air temperature. In dry places, like deserts of the southwest, air temperature can be 50 or 60 degrees above the dew point. Generally, dew point is a more reliable indicator of humidity than relative humidity because dew point is not affected by a change in air temperature and doesn’t fluctuate much throughout the day. Humidity can also affect plant turgor pressure, which is an indicator of the amount of water in plant cells. When humidity is low, and dew points are in the 50s and low 60s, moisture evaporates from plants very quickly. When this happens, plants can wilt rapidly if too much water is pulled out of plant cells through transpiration. Conversely, when humidity and temperature are both high, plants can get overheated because transpiration is reduced, thus restricting evaporative cooling. Humidity also influences plant diseases, especially fungi and molds that grow and spread rapidly when humidity is high. Humidity can also affect the fruit set of some plant species. An example is the bean (Phaseolus spp.), which has been shown to respond negatively to low humidity during fruit set. Humidity is also an important consideration for post-harvest storage of crops. Cold temperature and low humidity are important for the long-term storage of grains (corn, wheat etc). Conversely, fleshy vegetables and fruits (apples, cucumbers) need to be stored in cold temperatures and high humidity in order to prevent water loss from the fruit that results in shrivelling. 2.4 Wind What is Wind? Wind is the horizontal motion of the air past a given point. It is measured in terms of direction and speed. It is a very influential factor in several hydrometeorological processes, such as evaporation, transpiration and snowmelt. In the desert areas, wind is an important agent of erosion. It is also important in the production of precipitation, since it is only through sustained flow of moist air into a storm What Is Wind? How Does Wind Form? Wind is the horizontal motion of the air past a given point. Winds begin with differences in air pressures. When air pressure is higher at one place than another, it sets up a force. This force pushes from the high pressure towards the lower pressure. The greater the difference in pressures, the stronger the force. It’s important to note that there’s no set number that divides high and low pressure. What Determines Wind Speed? Wind speed is determined by air pressure. Besides force, the distance between the area of high pressure and the area of low pressure also determines how fast the moving air accelerates. Meteorologists refer to the force that starts the wind flowing as the “pressure gradient force.” The higher the pressure gradient force, the faster the wind speed. Wind Observations Wind is observed in terms of three important parameters, as follows: 1. Wind run is the total run of wind in 24 hours, expressed in kilometers and tenths of kilometer. 2. Wind direction is the direction from where the wind is blowing or comes. The direction that is frequently recorded through the observation is termed as prevailing wind direction. The terms used to describe the wind direction are north, east, south and west. In terms of angular displacement, said directions are indicated as 360, 90, 180, and 270 degrees from the true north (zero point), respectively. In common parlance, the wind directions are referred to as compass points, such as N, S, SW, etc., or in old records, they are expressed in terms of degrees. OTHER WIND FACTORS Wind is used to describe the prevailing direction from which the wind is blowing with the speed given usually in miles per hour or knots. Also, friction along the ground and the Coriolis effect plays a significant part in the speed and direction of wind. Rougher surfaces have higher friction, which causes the winds to be weaker and change the direction towards low pressure. The Coriolis Effect is an effect whereby a mass moving in a rotation system experiences a force (the Coriolis force) acting perpendicular to the direction of motion and to the axis of rotation. WHAT ARE WIND GUSTS? The National Weather Service (NWS) defines wind gusts as sudden, brief increases in wind speed. 2.5 Patterns affecting Philippine weather and climate Weather- “the state of the atmosphere with respect to heat or cold, wetness or dryness, calm or storm, clearness or cloudiness”. Climate – “the average course or condition of the weather at a place usually over a period of years as exhibited by temperature, wind velocity, and precipitation”. Weather refers, generally, to day-to-day temperature and precipitation activity, whereas climate is the term for the average atmospheric conditions over longer periods of time. Climate of the Philippines Itis tropical and maritime high temperature high humidity and abundant rainfall. Temperature, humidity, and rainfall, are the most important elements of the country's weather and climate. TEMPERATURE Based on the average of all weather stations in the Philippines, excluding Baguio, the mean annual temperature is 26.6°C. The coolest months fall in January with a mean temperature of 25.5°C while the warmest month occurs in May with a mean temperature of 28.3°C. Latitude is an insignificant factor in the variation of temperature while altitude shows greater contrast in temperature. Thus, the mean annual temperature of Baguio with an elevation of 1,500 meters is 18.3°C. The difference between the mean annual temperature of the southernmost station in Zamboanga and that of the northermost station in Laoag is insignificant. In other words, there is essentially no difference in the mean annual temperature of places in Luzon, Visayas or Mindanao measured at or near sea level. HUMIDITY refers to the moisture content of the atmosphere. Due to high temperature and the surrounding bodies of water, the Philippines has a high relative humidity. The average monthly relative humidity varies between 71 percent in March and 85 percent in September. The combination of warm temperature and high relative and absolute humidities give rise to high sensible temperature throughout the archipelago. It is especially uncomfortable during March to May, when temperature and humidity attain their maximum levels. RAINFALL It is the most important climatic element in the Philippines. Rainfall distribution throughout the country varies from one region to another, depending upon the direction of the moisture-bearing winds and the location of the mountain systems. The mean annual rainfall of the Philippines varies from 965 to 4,064 millimeters annually. Baguio City, eastern Samar, and eastern Surigao receive the greatest amount of rainfall while the southern portion of Cotabato receives the least amount of rain. At General Santos City in Cotabato, the average annual rainfall is only 978 millimeters. THE SEASONS Using temperature and rainfall as bases, the climate of the country can be divided into two major seasons: the rainy season, from June to November; the dry season, from December to May. The dry season may be subdivided further into: (a) the cool dry season, from Dec.to Feb.; (b) the hot dry season, from March to May. Climate Types Based on the distribution of rainfall, four climate types are recognized, which are described as follows: Typhoons have a great influence on the climate and weather conditions of the Philippines. A great portion of the rainfall, humidity and cloudiness are due to the influence of typhoons. They generally originate in the region of the Marianas and Caroline Islands of the Pacific Ocean which have the same latitudinal location as Mindanao. Their movements follow a northwesterly direction, sparing Mindanao from being directly hit by majority of the typhoons that cross the country. This makes the southern Philippines very desirable for agriculture and industrial development. Type I: Two pronounced seasons, dry from December to May and wet from June to November. Maximum rain period is from June to September. Areas characterized by this climate type are generally exposed to the southwest monsoon and get a fair share of the rainfall brought about by the tropical cyclones occurring especially during the maximum rain period. Type II: No dry season with a very pronounced maximum rain period in winter. Maximum rainfall generally occur in December and January, although there is not a single dry month. Areas characterized by this climate type are generally along or very near the eastern coast, thus are open to the northeast monsoon. Type III: No very pronounced maximum rain period, with a short dry season lasting only from one to three months. This type is intermediate between the preceding two, although it resembles the first type more closely because it has a short dry season. Areas of this climate type are partly shielded from the northeast monsoon but are exposed to the southwest monsoon and are also benefited by the rainfall caused by the tropical cyclones. Type IV: Rainfall is more or less evenly distributed throughout the year. This is also intermediate between the first and second types, although it resembles the second more closely since it has no dry season.