Chapter 18: Alkali Metals and Their Compounds PDF
Document Details
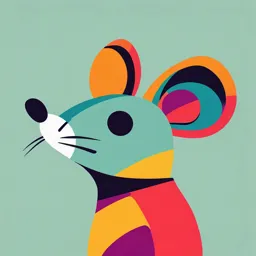
Uploaded by NourishingRoseQuartz
Tags
Summary
This chapter discusses the properties, extraction methods, and compounds of alkali metals found in group 1 of the periodic table, including lithium, sodium, potassium, rubidium, cesium, and francium. The document describes their electronic configurations, occurrence in ores, extraction methods, physical properties, and chemical reactions.
Full Transcript
60 Chapter E3 18 s and p-Block Elements (iii) Potassium : Sylime (KCl), carnallite (KCl.MgCl2.6H2O) and Felspar (K2O.Al2O3.6SiO2) ID Alkali Metals and Their Compounds (iv) Rubidium : Lithium ores Lepidolite, triphylite contains 0.7 to 3% Rb2O (v) Caesium : Lepidolite, Pollucite contains 0.2 to 7% Cs...
60 Chapter E3 18 s and p-Block Elements (iii) Potassium : Sylime (KCl), carnallite (KCl.MgCl2.6H2O) and Felspar (K2O.Al2O3.6SiO2) ID Alkali Metals and Their Compounds (iv) Rubidium : Lithium ores Lepidolite, triphylite contains 0.7 to 3% Rb2O (v) Caesium : Lepidolite, Pollucite contains 0.2 to 7% Cs2O U The group 1 of the periodic table contains six elements, namely lithium (Li), sodium (Na), potassium (K), rubidium (Rb), caesium (Cs) and francium (Fr). All these elements are typical metals. Francium is D YG radioactive with longest lived isotope 223 Fr with half life period of only 21 minute. These are usually referred to as alkali metals since their hydroxides form strong bases or alkalies. (1) Electronic configuration Elements Discovery Electronic configuration ( ns 1 ) 11 Na 19 37 K Rb [He ] 2 2 s 1 Davy (1807) [Ne] 10 3 s 1 Davy (1807) [Ar]18 4 s 1 Bunsen (1861) [Kr]36 5 s 1 Bunsen (1860) [Xe]54 6 s 1 Percy (1939) [Rn]86 7 s 1 ST 55 Cs Arfwedson (1817) U 3 Li 87 Fr (2) Occurrence : Alkali metals are very reactive and thus found in combined state some important ores of alkali metals are given ahead. (i) Lithium : Triphylite, Petalite, lepidolite, Spodumene [LiAl(SiO3)3], Amblygonite [Li(Al F)PO4] (ii) Sodium : Chile salt petre (NaNO3), Sodium chloride (NaCl), Sodium sulphate (Na2SO4), Borax (Na2B4O710H2O), Glauber salt (Na2 SO4.10H2O) (3) Extraction of alkali metals : Alkali metals cannot be extracted by the usual methods for the extraction of metals due to following reasons. (i) Alkali metals are strong reducing agents, hence cannot be extracted by reduction of their oxides or other compounds. (ii) Being highly electropositive in nature, it is not possible to apply the method of displacing them from their salt solutions by any other element. (iii) The aqueous solutions of their salts cannot be used for extraction by electrolytic method because hydrogen ion is discharged at cathode instead of an alkali metal ions as the discharge potentials of alkali metals are high. However, by using Hg as cathode, alkali metal can be deposited. The alkali metal readily combines with Hg to form an amalgam from which its recovery difficult. The only successful method, therefore, is the electrolysis of their fused salts, usually chlorides. Generally, another metal chloride is added to lower their fusion temperature. fusion Fused NaCl : NaCl Na Cl – Electrolys is : Anode : 2Cl Cl 2 2e of fused salt : Cathode : 2 Na 2e 2 Na (4) Alloys Formation (i) The alkali metals form alloys themselves as well as with other metals. among (ii) Alkali metals also get dissolved in mercury to form amalgam with evolution of heat and the amalgamation is highly exothermic. 301.5 453.5 370.8 336.2 312.0 1038.5 961.0 – boiling point (K) 1620 1154.4 978.0 (1) Physical state (ii) The lattice energy of these atoms in metallic crystal lattice relatively low due to larger atomic size and thus possess low melting point and boiling point on moving down the group, the atomic size increases and binding energy of their atoms in crystal lattice decreases which results lowering of melting point. (iii) Lattice energy decreases from Li to Cs and thus melting point and boiling also decreases from Li to Cs. (5) Ionisation energy and electropositive or metallic character (i) Due to unpaired lone electron in ns sub-shell as well as due to their larger size, the outermost electron is far from the nucleus, the removal of electron is easier and these low values of ionisation energy. (I.E.) (ii) Ionisation energy of these metal decreases from Li to Cs. K 152 186 Ionic radius of M+ – 60 ions (pm) Rb Cs 227 95 133 Fr 248 265 148 169 D YG Li ST U (3) Density (i) All are light metals, Li, Na and K have density less than water. Low values of density are because these metals have high atomic volume due to larger atomic size. On moving down the group the atomic size as well as atomic mass both increase but increase in atomic mass predominates over increase in atomic size or atomic volume and therefore the ratio mass/volume i.e. density gradually increases down the groups (ii) The density increases gradually from Li to Cs, Li is lightest known metal among all. Li = 0.534, Na = 0.972, K = 0.86, Rb = 1.53 and Cs = 1.87 g/ml at 200C. (iii) K is lighter than Na because of its unusually large atomic size. (iv) In solid state, they have body centred cubic lattice. (4) Melting point and Boiling point (i) All these elements possess low melting point and boiling point in comparison to other group members. Li Na K Ionisation energy Fr Rb Cs Li IE1 520 IE2 7296 4563 U Na Atomic radius (pm) 375 ID (ii) These form diamagnetic colourless ions since these ions do not have unpaired electrons, (i.e. M+ has ns0configuration). That is why alkali metal salts are colourless and diamagnetic. (2) Atomic and ionic radii (i) The alkali metals have largest atomic and ionic radii than their successive elements of other groups belonging to same period. (ii) The atomic and ionic radii of alkali metals, however, increases down the group due to progressive addition of new energy shells. No doubt the nuclear charge also increases on moving down the group but the influence of addition of energy shell predominates E3 to surface oxidation. – 60 Physical properties (i) All are silvery white, soft and light solids. These can be cut with the help of knife. When freshly cut, they have bright lustre which quickly tarnishes due Fr melting point (K) 495 Na K 418 403 3069 2650 A jump in 2nd ionisation difference) can be explained as, Re movalof Re moval of 2 s electron 1 s electron Rb 376 2420 energy Cs – – (huge Li :1s 2 2 s1 Li :1s 2 Li 2 : 1 s1 Removal of 1s electrons from Li+ and that too from completely filled configuration requires much more energy and a jump in 2nd ionisation is noticed. (iii) Lower are ionisation energy values, greater is the tendency to lose ns1 electron to change in M+ ion (i.e. M M++e–) and therefore stronger is electropositive character. (iv) Electropositive character increases from Li to Cs. Due to their strong electropositive character, they emit electrons even when exposed to light showing photoelectric effect. This property is responsible for the use of Cs and K in photoelectric cell. (6) Oxidation number and valency (i) Alkali metals are univalent in nature due to low ionisation energy values and form ionic compounds. Lithium salts are, however, covalent. (ii) Further, the M+ ion acquires the stable noble gas configuration. It requires very high values of energy to pull out another electron from next to outer shell of M+ ion and that is why their second ionisation energy is very high. Consequently, under ordinary (7) Hydration of Ions (i) Hydration represents for the dissolution of a substance in water to get adsorb water molecule by weak valency force. Hydration of ions is the exothermic process (i.e energy is released during hydration) when ions on dissolution water get hydration. (ii) The energy released when 1 mole of an ion in the gaseous state is dissolved in water to get it hydrated is called hydration energy Aq M (aq) ; H – ve. (iii) Smaller the cation, greater is the degree of hydration. Hydration energy is in the order of, Li+ > Na+ > K+ > Rb+ > Cs+ Specific heat (Cal/g) 0.049 – D YG Cs+ > Rb+ > K+ > Na+ > Li+ + + + + Relative hydrated ionic radii Li > Na > K > Rb > Cs Relative conducting power + Cs+ > Rb+ > K+ > Na + > Li+ U (8) Electronegativity, Electro positivity and metallic character. ST (i) These metals are highly electropositive and thereby possess low values of electronegativities. Metallic character and electro positivity increase from Li to Cs (Li < Na < K < Rb < Cs) (ii) Electronegativity of alkali metals decreases down the group as the trend of numerical values of electronegativity given below on Pauling scale suggests. Li Na K Rb Cs 0.82 0.82 0.79 Fr Electronegativity – 0.98 0.93 Rb Cs 0.941 0.293 0.17 0.08 (10) Conduction power : All are good conductors of heat and electricity, because of loosely held valence electrons. (11) Standard oxidation potential and reduction properties (i) Since alkali metals easily lose ns1 electron and thus they have high values of oxidation potential i.e., M aq M (aq) e (ii) The standard oxidation potentials of a alkali metals (in volts) are listed below, Li Na +3.05 +2.71 K +2.93 Rb Cs +2.99 +2.99 (iii) More is oxidation potential, more is the tendency to get oxidized and thus more powerful is reducing nature in aqueous medium that is why alkali metals liberate H2 from H2O and HCl. 2 H 2O 2 M 2 MOH H 2 ; 2 HCl 2 M 2 MCl H 2 (iv) However, an examination of ionisation energy for alkali metals reveals that Li should have the minimum tendency to lose electron and thus its reducing nature should be minimum. The greatest reducing nature of Li in aq. medium is accounted due to the maximum hydration energy of Li+ ion. For Lithium U (iv) Li+ being smallest in size has maximum degree of hydration and that is why lithium salts are mostly hydrated, LiCl. 2H2O also lithium ion being heavily hydrated, moves very slowly under the influence of electric field and, therefore, is the poorest conductor current among alkali metals ions It may, therefore, be concluded that it is the degree of hydration as well as the size of ion is responsible for the current carried by an ion. Relative ionic radii K ID M ( g) Na 60 (iii) Since the electronic configuration of M+ ions do not have unpaired electron and thus alkali metal salts are diamagnetic and colourless. Only those alkali metal salts are coloured which have coloured anions e.g. K2Cr2O7 is orange because of orange coloured Cr2O72- ion, KMnO4 is violet because of violet coloured MnO41- ion. Li Fr E3 conditions, it is not possible for these metals to form M2+ ion and thus they show +1 oxidation state. Fr being radioactive elements and thus studies on physical properties of this element are limited. (9) Specific heat : It decreases from Li to Cs. Li(s) Li(g) ; H1 = Heat of sublimation, Hs Li(g) Li (g) e; H2 = IE1 Li (g) Li (aq); Li(s) H 2O H3 = – Heat of hydration, Hh Li(aq) e; H H1 H 2 H 3 H s IE1 Hh Similarly, for sodium, Na(s) H 2 O Na (aq) e; H H(s) IE1 H h Hh for Li > Hh for Na. Therefore, large negative H values are observed in case of Li and this explains for more possibility of Li to get itself oxidized or have reducing nature. (12) Characteristic flame colours : The alkali metals and their salts give characteristic colour to Bunsen flame. The flame energy causes and excitation of the outermost electron which on reverting back to its initial position gives out the absorbed energy as visible light. These colour differ from each other Li –crimson, Na–Golden yellow, K – Pale violet , Rb-Red violet and Cs –Blue violet. These different colours are due to different ionisation energy of alkali metals. The energy released is minimum in the case of Li+ and increases in the order. Energy released : Li+ < Na+ < K+ < Rb+ < Cs+ released : Li+ > Na+ > K+ > Rb+ > Cs+ Frequency released : Li+ < Na+ < K+ < Rb+ < Cs+ (1) Formation of oxides and hydroxides (i) These are most reactive metals and have strong affinity for O2 quickly tranish in air due to the formation of a film of their oxides on the surface. These are, therefore, kept under kerosene or paraffin oil to protect them from air, M O2 M 2 O M 2 O 2 Peroxide (ii) When burnt air (O2), lithium forms lithium oxide (Li2O) sodium forms sodium peroxide (Na2O2) and other alkali metals form super oxide (Mo2 i.e. KO2,RbO2 or CsO2) 2 Li Potassium super oxide to form stable oxides. The monoxide, peroxides and superoxides have O2 D YG and O 22 , O 12 ions respectively. The structures of each........ are, [:O... :O...... O: [x. O.. O.x 1– Superoxide (O22–) O:] Monoxide (O2) Peroxide ]2– (O2–) O2–1 U The ion has a three electron covalent bond and has one electron unpaired. It is therefore superoxides are paramagnetic and coloured KO2 is light yellow and paramagnetic substance. (iii) The oxides of alkali metals and metal itself give strongly alkaline solution in water with evolution of heat 1 H2; 2 Li2O H 2O 2 LiOH ; ST M H 2 O MOH (v) The electrolysis of fused hydrides give H2 at anode. NaH fused Contains Na and H i.e., U oxygen anion i.e, (iv) The ionic nature of hydrides increases from Li to Cs because of the fact that hydrogen is present in the these hydrides as H– and the smaller cation will produce more polarisation of anion (according to Fajans rule) and will develop more covalent character. ID The reactivity of alkali metals towards oxygen to form different oxides is due to strong positive field around each alkali metal cation. Li+ being smallest, possesses strong positive field and thus combines with small anion O2– to form stable Li2O compound. The Na+ and K+ being relatively larger thus exert less strong positive field around them and thus reacts with larger O 12 (ii) The tendency to form their hydrides, basic character and stability decreases from Li to Cs since the electropositive character decreases from Cs to Li. (iii) The metal hydrides react with water to give MOH and H2 ; MH + H2 O MOH + H2 KO 2 O 22 and (i) These metals combine with H to give white crystalline ionic hydrides of the general of the formula MH. 2M+ H2 2MH ; Reactivity towards H2 is Cs < Rb < K < Na < Li. 1 O 2 Li2 O ; 2 Na O 2 Na 2 O2 2 Lithuim oxide K O2 (2) Hydrides E3 Oxide (v) The basic character of oxides and hydroxides of alkali metals increases from Li to Cs. This is due to the increase in ionic character of alkali metal hydroxides down the group which leads to complete dissociation and leads to increase in concentration of OH– ions. 60 Chemical properties (vi) Alkali metals also form hydrides like NaBH4, LiAlH4 which are good reducing agent. (3) Carbonates and Bicarbonates (i) The carbonates (M2CO3) & bicarbonates (MHCO3) are highly stable to heat, where M stands for alkali metals. (ii) The stability of these salts increases with the increasing electropositive character from Li to Cs. It is therefore Li2CO3 decompose on heating, Li2CO3 Li2O+CO2 0 300 C M 2 CO 3 H 2 O CO 2 low temperature, 2 MHCO 3 H ve 2 KO 2 2 H 2 O 2 KOH H 2 O 2(l) O 2(g) ; At anode: (iii) Bicarbonates are decomposed at relatively H ve Na 2 O 2 2 H 2 O 2 NaOH H 2 O 2(l) ; At cathode: Na+ +e– Na; 1 H H2 e 2 H ve H ve The peroxides and superoxides act as strong oxidising agents due to formation of H2O2 (iv) The reactivity of alkali metals towards air and water increases from Li to Cs that is why lithium decomposes H2O very slowly at 25oC whereas Na does so vigorously, K reacts producing a flame and Rb, Cs do so explosively. 1 M H 2 O MOH H 2 2 (iv) Both carbonates and bicarbonates are soluble in water to give alkaline solution due to hydrolysis of carbonate ions or bicarbonate ions. (4) Halides (i) Alkali metals combine directly with halogens to form ionic halide M X . (ii) The ease with which the alkali metals form halides increases from Li to Cs due to increasing electropositive character from Li to Cs. (iii) Lithium halides however have more covalent nature. Smaller is the cation, more is deformation of (v) Halides having ionic nature have high m.pt. and good conductor of current. The melting points of halides shows the order, NaF > NaCl > NaBr > Nal (vi) Halides of potassium, rubidium and caesium have a property of combining with extra halogen atoms forming polyhalides. KI + I2 KI3 ; In KI3(aq) the ions K+ and I–3 are present (5) Solubility in liquid NH3 (8) Reaction with non-metals (i) These have high affinity for non-metals. Except carbon and nitrogen, they directly react with hydrogen, halogens, sulphur, phosphorus etc. to form corresponding compounds on heating. 0 300 C 2NaH 2Na + H2 ; 2K + H22KH 2Na + Cl2 2NaCl ; 2K + Cl2 2KCl 2Na + S Na2S ; 2K + S K2S 3Na + P Na3P ; 3K + P K3P (ii) Li reacts, however directly with carbon and nitrogen to form carbides and nitrides. 2Li + 2C LiC2 ; 6Li + 2N2 2 Li3N (iii) The nitrides of these metals on reaction with water give NH3. M3N + 3H2O 3MOH + NH3 (9) Reaction with acidic hydrogen : Alkali metals react with acids and other compounds containing acidic hydrogen (i.e, H atom attached on F,O, N and triply bonded carbon atom, for example, HF, H2O, ROH, RNH2, CH CH) to liberate H2. 1 1 M H 2 O MOH H 2 ; M HX MX H 2 2 2 ID (i) These metals dissolve in liquid NH3 to produce blue coloured solution, which conducts electricity to an appreciable degree. (iv) The sulphates of alkali metals (except Li) form double salts with the sulphate of the trivalent metals like Fe, Al, Cr etc. The double sulphates crystallize with large number of water molecules as alum. e.g. K2SO4. Al2 (SO4)3. 24 H2O. 60 (iv) These are readily soluble in water. However, lithium fluoride is sparingly soluble. The low solubility of LiF is due to higher forces of attractions among smaller Li+ and smaller F– ions (high lattice energy). (iii) These sulphates on fusing with carbon form sulphides, M2SO4 + 4C M2S + 4CO E3 anion and thus more is covalent nature in compound. Also among lithium halides, lithium iodide has maximum covalent nature because of larger anion which is easily deformed by a cation. Thus covalent character in lithium halides is, LiI > LiBr > LiCl > LiF U (ii) With increasing concentration of ammonia, blue colour starts changing to that of metallic copper after which dissolution of alkali metals in NH3 ceases. D YG (iii) The metal atom is converted into ammoniated metal in i.e. M+ (NH3) and the electron set free combines with NH3 molecule to produce ammonia solvated electron. Na (x y)NH 3 [ Na( NH 3 )x ] [e( NH 3 )y ] Ammoniated cation Ammoniated electron (iv) It is the ammoniated electron which is responsible for blue colour, paramagnetic nature and reducing power of alkali metals in ammonia solution. However, the increased conductance nature of these metals in ammonia is due to presence of ammoniated cation and ammonia solvated electron. U (v) The stability of metal-ammonia solution decreases from Li to Cs. ST (vi) The blue solution on standing or on heating slowly liberates hydrogen, 2M + 2NH3 2MNH2 + H2. Sodamide (NaNH2) is a waxy solid, used in preparation of number of sodium compounds. (6) Nitrates : Nitrates of alkali metals (MNO3) are soluble in water and decompose on heating. LiNO3 decomposes to give NO2 and O2 and rest all give nitrites and oxygen. 2MNO3 2MNO2 + O2 (except Li) 4 LiNO3 2Li2O + 4NO2 + O2 (7) Sulphates (i) Alkali metals’ sulphate have the formula M2SO4. (ii) Except Li2SO4, rest all are soluble in water. M ROH ROH 1 1 H 2 ; M RNH 2 RNHNa H 2 2 2 (10) Complex ion formation : A metal shows complex formation only when it possesses the following characteristics, (i) Small size (ii) High nuclear charge (iii) Presence of empty orbitals in order to accept electron pair ligand. Only Lithium in alkali metals due to small size forms a few complex ions Rest all alkali metals do not possess the tendency to form complex ion. Anomalous behaviour of Lithium Anomalous behaviour of lithium is due to extremely small size of lithium its cation on account of small size and high nuclear charge, lithium exerts the greatest polarizing effect out of all alkali metals on negative ion. Consequently lithium ion possesses remarkable tendency towards solvation and develops covalent character in its compounds. Li differs from other alkali metals in the following respects, (1) It is comparatively harder than other alkali metals. Li can’nt be stored in kerosene as it floats to the surface, due to its very low density. Li is generally kept wrapped in parrafin wax. (i) It is not affected by air. (ii) It decomposes water very slowly to liberate H2. (iii) It hardly reacts with bromine while other alkali metals react violently. (4) Lithium is the only alkali metal which directly reacts with N2 to form Lithium nitride (Li3N) (5) Lithium when heated in NH3 forms amide, Li2 NH while other metals form amides, MNH2. (6) When burnt in air, lithium form Li2O sodium form Na2O and Na2O2 other alkali metals form monoxide, peroxide and superoxide. (7) Li2O is less basic and less soluble in water than other alkali metals. 2 LiOH Li 2 O H 2 O (9) LiHCO3 is liquid while other metal bicarbonates are solid. heat Li2 CO 3 Li2 O CO 2. D YG Na2CO3, K2CO3 etc. do not decompose on heating. (11) LiNO3 and other alkali metal nitrates give different products on heating 4LiNO3 = 2Li2O+4NO2 + O2 ; 2NaNO3 = 2NaNO2 + O2 Group II Li Be 3 Na Mg (1) Both Li and Mg are harder and higher m.pt than the other metals of their groups. (2) Due to covalent nature, chlorides of both Li and Mg are deliquescent and soluble in alcohol and pyridine while chlorides of other alkali metals are not so. (3) Fluorides, phosphates of Li and Mg are sparingly soluble in water whereas those of other alkali metals are soluble in water. (4) Carbonates of Li and Mg decompose on heating and liberate CO2 Carbonates of other alkali metals are stable towards heat and decomposed only on fusion. Li2CO3 Li2O + CO2 ; Mg CO3 MgO + CO2 (5) Hydroxides and nitrates of both Li and Mg decompose on heating to give oxide. Hydroxides of both Li and Mg are weak alkali. U (10) Only Li2CO3 decomposes on heating Group I 2 ID (8) LiOH is weaker base than NaOH or KOH and decomposes on heating. Period 60 (3) Unlike other alkali metals, lithium is least reactive among all. It can be noticed by the following properties, to Mg Its resemblance with Mg is known as diagonal relationship. Generally the periodic properties show either increasing or decreasing trend along the group and vice versa along the period which brought the diagonally situated elements to closer values. Following are the characteristic to be noted. E3 (2) It can be melted in dry air without losing its brilliance. (12) LiCl and LiNO3 are soluble in alcohol and other organic solvents. These salts of other alkali metals are, however, insoluble in organic solvents. U (13) LiCl is deliquescent while NaCl, KBr etc. are not. Lithium chloride crystals contain two molecules of water of crystallisation (LiCl. 2H2O). Crystals of NaCl KBr, KI etc do not conation water of crystallisation. ST (14) Li2SO4 does not form alums like other alkali metals. (15) Li reacts with water slowly at room temperature Na reacts vigorously Reaction with K. Rb and Cs is violent. (16) Li reacts with Br2 slowly. Reaction of other alkali metals with Br2 is fast. (17) Li2 CO3 Li2C2O4, LiF , Li3PO4 are the only alkali metal salts which are insoluble or sparingly soluble in water. Diagonal Relationship of Li with Mg Due to its small size lithium differs from other alkali metals but resembles with Mg as its size is closer 4 LiNO3 2Li2O + 4NO2 + O2 2Mg(NO3)2 2MgO + 4NO2 + O2 2LiOH Li2O + H2O ; Mg(OH)2 MgO + H2O Hydroxides of other alkali metals are stable towards heat while their nitrates give O2 and nitrite. 2KNO3 2KNO2 + O2 (6) Both Li and Mg combine directly with N2 to give nitrides Li3N and Mg3N2. Other alkali metals combine at high temperature, 6Li + N2 2Li3N; 3Mg + N2 Mg3N2. Both the nitrides are decomposed by water to give NH3 Li3N + 3H2O 3LiOH + NH3 ; Mg3N2 + 6H2O 3Mg(OH)2+ 2NH3 (7) Bicarbonates of Li and Mg are more soluble in water than carbonates whereas carbonates of alkali metals are more soluble. (8) Both Li and Mg combine with carbon on heating. 2Li + 2C Li2C2 ; Mg + 2C Mg C2 (9) The periodic properties of Li and Mg are quite comparable Electronegativity Li Mg 1.0 1.2 Atomic radii 1.36 1.23 Anode and cathode are separated by means of a wire gauze to prevent the reaction between Na and Cl 2. 0.60(Li+) Ionic radii 0.65(Mg+2) (3) Compound of sodium 12.97 c.c (i) Sodium chloride : It is generally obtained by evaporation of sea water by sun light. However NaCl so obtained contains impurities like CaSO 4 , CaCl 2 and Polarizing Power = Ionic charge / (ionic radius) 2. (11) Li and Mg Form only monooxide on heating in oxygen. 4Li + O2 2 Li2O ; 2Mg + O2 2 MgO (12) Li2SO4 like MgSO4 does not form alums. (13) The bicarbonates of Li and Mg do not exist in solid state, they exist in solution only. (14) Alkyls of Li and Mg (R. Li and R.MgX) are soluble in organic solvent. of Cl ions increases and as a result pure NaCl gets precipitated due to common ion effect. (ii) Sodium hydroxide NaOH (Caustic soda) Preparation (a) Gossage process : Na 2CO 3 Ca(OH )2 2 NaOH CaCO 3 (10% solution) (b) Electrolytic method : Caustic soda is manufactured by the electrolysis of a concentrated solution of NaCl. ID (15) Lithium chloride and MgCl2 both are deliquescent and separate out from their aqueous solutions as hydrated crystals, LiCl. 2H2O and MgCl2. 2H2O. MgCl 2 which makes the salt deliquescent. It is then purified by allowing HCl gas to pass through the impure saturated solution of NaCl. The concentration 60 (10) Both have high polarizing power. E3 Atomic volume 13.97 c.c Uses of Lithium (2) It is used as an alloying metal with (i) Pb to give toughened bearings. Cl discharged; D YG (ii) Al to give high strength Al-alloy for aircraft industry. (iii) Mg (14% Li) to give extremely tough and corrosion resistant alloy which is used for armour plate in aerospace components. Sodium and its compounds (1) Ores of sodium : NaCl (common salt), NaNO 3 sodium borate, U or ST (2) Extraction of sodium : It is manufactured by the electrolysis of fused sodium chloride in the presence of CaCl 2 and KF using graphite anode and iron cathode. This process is called Down process. NaCl ⇌ Na Cl. Outer compartment – Brine solution is electrolysed ; Central compartment – 2% NaOH solution and H 2 Properties : White crystalline solid, highly soluble in water, It is only sparingly soluble in alcohol. (a) Reaction with salt : FeCl 3 3 NaOH because 2.71V). Fe(OH )3 (Insoluble hy droxide) 3 NaCl HgCl 2 2 NaOH 2 NaCl Hg(OH )2 H 2 O HgO yellow unstable AgNO 3 2 NaOH 2 NaNO 3 2 AgOH Ag 2 O H 2 O Brown Zn, Al, Sb , Pb, Sn and As forms insoluble hydroxide which dissolve hydroxide). in excess of NaOH (amphoteric (b) Reaction with halogens : Cl Cl Cl 2 Sodium cannot be extracted from aqueous NaCl EH0 2 O / H 2 Na heat NH 4 Cl NaOH NaCl NH 3 H 2 O At cathode : Na e Na ; At anode : Cl Cl e ; cathode: (c) Castner - Kellener cell (Mercury cathode process) : NaOH obtained by electrolysis of aq. solution of brine. The cell comprises of rectangular iron tank divided into three compartments. (chile salt petre), Na 2 SO 4.10 H 2 O (Glauber's salt), borax (sodium tetraborate (Na 2 B4 O7. 10 H 2 O). At U (1) It is used as a deoxidiser in metallurgy of Cu and Ni. At anode: discharged 0 (–0.83V) is more than E Na / Na (– X 2 2 NaOH (cold) NaX NaXO H 2 O sod. hy pohalite 3 X 2 6 NaOH (hot) 5 NaX NaXO 3 3 H 2O ; (Sod. halate) (X Cl, Br, I) (c) Reaction with metals : Weakly electropositive metals like Zn, Al and Sn etc. (d) Na 2 CO 3 H 2 O CO 2 2NaHCO 3 Zn 2 NaOH Na 2 ZnO 2 H 2 Uses : In textile and petroleum refining, Manufacturing of glass, NaOH soap powders etc. (d) Reaction with sand, SiO2 : H 2O (iv) Sodium peroxide (Na2O2) Preparation : It is manufactured by heating sodium metal on aluminium trays in air (free from CO 2 ) (e) Reaction with CO: o 150 200 C NaOH CO HCOONa Sod. formate NaOH breaks down the proteins of the skin flesh to a pasty mass, therefore it is commonly known as caustic soda. Caustic property : sodium hydroxide breaks down the proteins of the skin flesh to a pasty mass, therefore, it is commonly known as caustic soda. Na 2 O 2 2 Na O2 (air) 60 5 10 atm Properties : (a) When pure it is colourless. The faint yellow colour of commercial product is due to presence of small amount of superoxide (NaO 2 ). (b) On coming with moist air it become white due to formation of NaOH and Na 2 CO 3. E3 Na 2 SiO 3 Sod. silicate (glass) 2 NaOH SiO 2 (c) It is readily decomposed by acids with the evolution of CO 2 gas. 2 Na 2 O2 2 H 2 O 4 NaOH O2 ; Uses : Sodium hydroxide is used : (a) in the manufacture of soidum metal, soap (from oils and fats), rayon, paper, dyes and drugs, 2 NaOH CO 2 Na 2 CO 3 H 2 O (c) It is powerful oxidising agent. It oxidises Cr (III) hydroxide to sodium chromate, Mn (II) to sodium manganate and sulphides to sulphates. (iii) Sodium carbonate or washing soda, Na 2 CO 3 Uses : As a bleaching agent and it is used for the purification of air in confined spaces such as submarines because it can combine with CO 2 to give cotton to make U (c) as a reagent in the laboratory. ID cloth (b) for mercurinzing unshrinkable and It exists in various forms, namely anhydrous sodium carbonate Na2CO2 (soda-ash); monohydrate Na 2 CO 3.H 2 O Na 2 CO 3.7 H 2 O and D YG (crystal carbonate); hyptahydrate decahydrate Na 2 CO3.10 H 2 O (washing soda or sal soda). Preparation : (a) Solvay process : In this process, brine (NaCl ) , NH 3 and CO 2 are the raw materials. Na 2 CO 3 and oxygen, 2CO 2 2 Na 2 O2 2 Na 2 CO 3 O 2. (v) Micro cosmic salt [Na (NH4) HPO4. 4H2O] Prepared by dissolving equimolar amounts of Na 2 HPO 4 and NH 4 Cl in water in 1 : 1 ratio followed by crystallization NH 3 CO 2 H 2 O NH 4 HCO3 NH 4 Cl Na 2 HPO 4 Na(NH 4 )HPO 4 NaCl Crystalliz ation oC 30 NH 4 HCO 3 NaCl NaHCO 3 NH 4 Cl Na( NH 4 )HPO 4.4 H 2 O oC 250 2 NaHCO 3 Na 2 CO 3 H 2 O CO 2 U 2 NH 4 Cl Ca(OH )2 CaCl 2 2 H 2 O 2 NH 3 slaked lime ST CaCl 2 so formed in the above reaction is a by product of solvay process. Chemical properties : On heating M.C.S, NaPO 3 is formed. NaPO 3 forms coloured beads with oxides of transition metal cloudy SiO 2 Na(NH 4 )HPO 4 NaPO 3 H 2 O NH 3 (Sodium meta phosphate) Properties dry air (a) Na 2 CO 3.10 H 2 O Na 2 CO 3.H 2 O 9 H 2 O (decahydra te) (M onohydra te) Na 2 CO 3. H 2 O (Colourles s cry stal) CuNaPO 4 (blue bead) NaPO 3 CoO CoNaPO 4 (blue bend) Na 2 CO 3 It does not decompose on funrther heating even to redness (m.pt. 853°C) (b) It is soluble in water with considerable evolution of heat. Na 2 CO 3 H 2 O H 2 CO 3 2 Na 2OH Weak acid NaPO 3 CuO (Trans parent glassy bead) NaPO 3 MnO NaMnO 4 (blue bead) Uses : (a) For the formation of sodium meta phosphate and copper sodium phosphate (b) It is used for the detection of colured ion (vi) Sodium bi Carbonate (NaHCO3, Baking soda) Preparation : It is an inter mediate compound in manufacture of sodium carbonate by the solvay’s process NaCl NH 3 CO 2 H 2 NaHCO 3 NH 4 Cl Properties: o 50 100 C 2 NaHCO 3 Na 2 CO 3 H 2 O CO 2 88 [Rn ]7 s 2 Ra Radium was discovered in the ore pitch blende by madam Curie. It is radioactive in nature. (2) Occurrence : These are found mainly in combined state such as oxides, carbonates and sulphates Mg and Ca are found in abundance in nature. Be is not very abundant, Sr and Ba are less abundant. Ra is rare element. Some important ores of alkaline earth metals are given below, 60 (c) It is espacially used for testing silica with which a cloudy bead containing floating properties of silica is obtained. (i) Baryllium Phenacite (Be2SiO4) It is amphiprotic HCO 3 H ⇌ H 2 CO 3 HCO 3 ⇌ H CO 32 : Beryl (3BeO.Al2O3.6SiO2); Improved Baking powder contains 40% starch 30% NaHCO 3 , 20% NaAl (SO 4 )2 and 10% CaH 2 (PO 4 ) (iii) Calcium : Limestone (CaCO3); Gypsum : (CaSO4.2H2O), Anhydrite (CaSO4); Fluorapatite [(3Ca3(PO4)2.CaF2)] Phosphorite rock [Ca3(PO4)2] Uses : (a) Baking powder contains Ca(H 2 PO 4 )2 and starch. (iv) Barium : Barytes (BaSO4) ; witherite (BaCO3) (vii) Sodium Sulphate Na2SO4 or salt cake (v) Radium : Pitch blende (U3O8); (Ra in traces); other radium rich minerals are carnotite [K2UO2)] (VO4)2 8H2O and antamite [Ca(UO2)2] ID (b) In pharmacentical industry (Antacids etc.) (c) Fire extingerishers. E3 NaHCO 3 , (ii) Magnesium : Magnesite (MgCO3); Dolomite (CaCO3. MgCO3); Epsomite(MgSO4. 7H2O); Carnallite (MgCl2.KCl. 6H2O); Asbestos [CaMg3(SiO3)4] Preparation : It is the by-product of HCl industry Properties : When aqueous solution of Na 2 SO 4 is D YG cooled below 32 o C Glauber’s salt (Na 2 SO 4.10 H 2 O) gets crystallised and if cooled to 12 o C , Na 2 SO 4 7 H 2 O crystals are formed. (indry air) Na 2 SO 4.10 H 2 O Na 2 SO 4 10 H 2 O Uses : Na 2 SO 4 finds use in paper industry detergent and glass manufacturing. Alkaline Earth Metals and Their Compounds ST U The group 2 of the periodic table consists of six metallic elements. These are beryllium (Be), magnesium (Mg), calcium (Ca), strontium (Sr), barium (Ba) and radium (Ra). These (except Be) are known as alkaline earth metals as their oxides are alkaline and occur in earth crust. (1) Electronic configuration Element 4 Be Electronic configurations ( ns 2 ) [ He ] 2 s 2 (i) Be and Mg are obtained by reducing their oxides carbon, U 2 NaCl H 2 SO 4 Na 2 SO 4 HCl (3) Extraction of alkaline earth metals BeO + C Be + CO ; MgO + C Mg + CO (ii) The extraction of alkaline earth metals can also be made by the reduction of their oxides by alkali metals or by electrolysing their fused salts. (4) Alloy formation : These dissolve in mercury and form amalgams. Physical properties (1) Physical state : All are greyish-white, light, malleable and ductile metals with metallic lustre. Their hardness progressively decrease with increase in atomic number. Although these are fairly soft but relatively harder than alkali metals. (2) Atomic and ionic radii (i) The atomic and ionic radii of alkaline earth metals also increase down the group due to progressive addition of new energy shells like alkali metals. Be Mg Mg [ Ne ] 3 s 2 Atomic radius (pm) 222 – 112 12 Ca [ Ar ] 4 s 2 Ionic radius of M2+ 135 140 31 20 38 Sr [Kr] 5 s 2 ion (pm) 56 Ba [ Xe ] 6 s 2 Ca Sr Ba 160 197 215 65 99 113 Ra (ii) The atomic radii of alkaline earth metals are however smaller than their corresponding alkali metal size. (3) Density (i) Density decreases slightly upto Ca after which it increases. The decrease in density from Be to Ca might be due to less packing of atoms in solid lattice of Mg and Ca. 1.84 Mg 1.74 Ca 1.55 Sr Ba 2.54 3.75 Sr 920 1112 1378 1767 1041 1654 1413 (ii) The values are, however, more than alkali metals. This might due to close packing of atoms in crystal lattice in alkaline earth metals. (5) Ionisation metallic character energy and electropositive or U (i) Since the atomic size decreases along the period and the nuclear charge increases and thus the electrons are more tightly held towards nucleus. It is therefore alkaline earth metals have higher ionisation energy in comparison to alkali metals but lower ionisation energies in comparison to p-block elements. ST (ii) The ionisation energy of alkaline earth metals decreases from Be to Ba. Be Mg Ca Sr Ba Ra First ionisation energy (k J mol-1) 503 509 899 removal of 2 s electron D YG 2770 520 7296 899 1757 removal of 1 s Be : 1s2 , 2s2 Be+ : 1s2, 2s1 removal of 2 s electron 1s2 Li2+ : 1s1 electron removal of 2 s Be2+ : electron The removal of 2nd electron from alkali metals takes place from 1s sub shell which are more closer to nucleus and exert more nuclear charge to hold up 1s electron core, whereas removal of 2nd electron from alkaline earth metals takes from 2s sub shell. More closer are shells to the nucleus, more tightly are held electrons with nucleus and thus more energy is required to remove the electron. (v) All these possess strong electropositive character which increases from Be to Ba. (vi) These have less electropositive character than alkali metals as the later have low values of ionisation energy. U 973 boiling point (K) – 1560 2nd ionisation energy (kJ mol–1) Li : 1s , 2s1 Li +: 1s2 Ba Ra 1000 1st ionisation energy (kJ mol ) ID Ca Be This may be explained as, (i) Melting points and boiling points of alkaline earth metals do not show any regular trend. melting points (K) Li –1 2 6.00 (4) Melting point and Boiling point Mg earth metals are higher than alkali metals but a closer look on 2nd ionisation energy of alkali metals and alkaline earth metals reveals that 2nd ionisation energy of alkali metals are more Ra (ii) The alkaline earth metals are more denser, heavier and harder than alkali metal. The higher density of alkaline earth metals is due to their smaller atomic size and strong intermetallic bonds which provide a more close packing in crystal lattice as compared to alkali metals. Be (iv) No doubt first ionisation energy of alkaline E3 Be required to pull one more electron from monovalent cation. 60 of the same period. This is due to the fact that alkaline earth metals possess a higher nuclear charge than alkali metals which more effectively pulls the orbit electrons towards the nucleus causing a decrease in 737 590 549 Second ionisation energy (kJ mol-1) 1757 1450 1146 965 979 1064 (iii) The higher values of second ionisation energy is due to the fact that removal of one electron from the valence shell, the remaining electrons are more tightly held in which nucleus of cation and thus more energy is (6) Oxidation number and valency (i) The IE1 of the these metals are much lower than IE1 and thus it appears that these metals should form univalent ion rather than divalent ions but in actual practice, all these give bivalent ions. This is due to the fact that M2+ ion possesses a higher degree of hydration or M2+ ions are extensively hydrated to form [M(H2O)x]2+, a hydrated ion. This involves a large amount of energy evolution which counter balances the higher value of second ionisation energy. M M2+ , H = IE1 + E2 M2+ + xH2O [M(H2O)x]2+; H = – hydration energy. (ii) The tendency of these metals to exist as divalent cation can thus be accounted as, (a) Divalent cation of these metals possess noble gas or stable configuration. (b) The formation of divalent cation lattice leads to evolution of energy due to strong lattice structure of divalent cation which easily compensates for the higher values of second ionisation energy of these metals. (c) The higher heats of hydration of divalent cation which accounts for the existence of the divalent ions of these metals in solution state. (7) Hydration of ions (i) The hydration energies of alkaline earth metals divalent cation are much more than the hydration energy of monovalent cation. (1) Formation of oxides and hydroxides 353 The abnormally higher values of heat of hydration for divalent cations of alkaline earth metals are responsible for their divalent nature. MgCl2 formation occurs with more amount of heat evolution and thus MgCl2 is more stable. (ii) The hydration energies of M2+ ion decreases with increase in ionic radii. Be2+ Mg2+ Ca2+ Sr2+ Ba2+ Heat of hydration kJ mol–1 1275 2382 1906 1651 Chemical Properties 1484 2M + O2 2MO (M is Be, Mg or Ca ) 2M + O2 MO2 (M is Ba or Sr) (ii) Their less reactivity than the alkali metals is evident by the fact that they are slowly oxidized on exposure to air, However the reactivity of these metals towards oxygen increases on moving down the group. (iii) The oxides of these metals are very stable due to high lattice energy. (iv) The oxides of the metal (except BeO and MgO) dissolve in water to form basic hydroxides and evolve a large amount of heat. BeO and MgO possess high lattice energy and thus insoluble in water. ID (iii) Heat of hydration are larger than alkali metals ions and thus alkaline earth metals compounds are more extensively hydrated than those of alkali metals e.g MgCl2 and CaCl2 exists as Mg Cl2.6H2O and CaCl2. 6H2O which NaCl and KCl do not form such hydrates. (i) The elements (except Ba and Ra) when burnt in air give oxides of ionic nature M2+O2- which are crystalline in nature. Ba and Ra however give peroxide. The tendency to form higher oxides increases from Be to Ra. 60 Mg Mg Hydration energy or Heat of hydration (kJ mol–1) 1906 2+ E3 + The characteristic flame colour shown are : Ca brick red; Sr –crimson ; Ba-apple green and Racrimson. U (iv) The ionic mobility, therefore, increases from Be2+ to Ba2+, as the size of hydrated ion decreases. (8) Electronegativities D YG (i) The electronegativities of alkaline earth metals are also small but are higher than alkali metals. (ii) Electronegativity decreases from Be to Ba as shown below, Be Electronegativity 0.89 Mg Ca Sr Ba 1.57 1.31 1.00 0.95 (9) Conduction power : Good conductor of heat and electricity. U (10) Standard oxidation potential and reducing properties (i) The standard oxidation potential (in volts) are, Mg Ca ST Be 1.69 2.35 2.87 (v) BeO dissolves both in acid and alkalies to give salts i.e. BeO possesses amphoteric nature. Sr Ba 2.89 2.90 (ii) All these metals possess tendency to lose two electrons to give M2+ ion and are used as reducing agent. (iii) The reducing character increases from Be to Ba, however, these are less powerful reducing agent than alkali metals. (iv) Beryllium having relatively lower oxidation potential and thus does not liberate H2 from acids. (11) Characteristic flame colours BeO+2NaOHNa2BeO2+H2O ; BeO+2HClBeCl2+H2O Sod. beryllate Beryllium chloride (vi)The basic nature of oxides of alkaline earth metals increases from Be to Ra as the electropositive Character increases from Be to Ra. (vii)The tendency of these metal to react with water increases with increase in electropositive character i.e. Be to Ra. (viii) Reaction of Be with water is not certain, magnesium reacts only with hot water, while other metals react with cold water but slowly and less energetically than alkali metals. (ix) The inertness of Be and Mg towards water is due to the formation of protective, thin layer of hydroxide on the surface of the metals. (x) The basic nature of hydroxides increase from Be to Ra. It is because of increase in ionic radius down the group which results in a decrease in strength of M – O bond in M –(OH)2 to show more dissociation of hydroxides and greater basic character. (xi) The solubility of hydroxides of alkaline earth metals is relatively less than their corresponding alkali metal hydroxides Furthermore, the solubility of hydroxides of alkaline earth metals increases from Be to Ba. Be (OH)2 and Mg (OH)2 are almost insoluble, Ca (OH)2 (often called lime water) is sparingly soluble H solution = H lattice energy More negative is H compounds. + H hydration energy solution more is solubility of (xii) The basic character of oxides and hydroxides of alkaline earth metals is lesser than their corresponding alkali metal oxides and hydroxides. (xiii) Aqueous solution of lime water [Ca(OH)2] or baryta water [Ba(OH)]2 are used to qualitative identification and quantative estimation of carbon dioxide, as both of them gives white precipitate with CO2 due to formation of insoluble CaCO3 or BaCO3 (white ppt) (white ppt) Heat MCO 3 MO CO 2 ID Ca(OH)2 + CO2 CaCO3 + H2O ; Ba(OH)2 + CO2 BaCO3 + H2O 60 The trend of the solubility of these hydroxides depends on the values of lattice energy and hydration energy of these hydroxides. The magnitude of hydration energy remains almost same whereas lattice energy decreases appreciably down the group leading to more –Ve values for H solution down the group. (NH4)2 CO3 + CaCl2 2NH4Cl + CaCO3 Na2CO3 + BaCl2 2NaCl + BaCO3 (ii) Alkaline earth metal carbonates are obtained as white precipitates when calculated amount of carbon dioxide is passed through the solution of the alkaline metal hydroxides. M(OH)2 (aq) + CO2 (g) MCO3(s) + H2O(l) and sodium or ammonium carbonate is added to the solution of the alkaline earth metal salt such as CaCl2. CaCl2 (aq) + Na2CO3 (aq) CaCO3(s) +2 NaCl(aq) (iii) Solubility of carbonates of these metals also decreases downward in the group due to the decrease of hydration energy as the lattice energy remains almost unchanged as in case of sulphates. (vi) The carbonates of these metals decompose on heating to give the oxides, the temperature of decomposition increasing from Be to Ba. Beryllium carbonate is unstable. E3 whereas Sr(OH)2 and Ba (OH)2 (often called baryta water) are more soluble. (4) Halides (i) The alkaline earth metals combine directly with halogens at appropriate temperatures forming halides, MX2. These halides can also be prepared by the action of halogen acids (HX) on metals, metal oxides, hydroxides and carbonates. M + 2HX MX2 + H2 ; MO + 2HX MX2 + H2O M(OH)2 + 2HX MX2 +2H2O MCO3 + 2HX MX2 + CO2 + H2O ST U D YG U SO2 also give white ppt of CaSO3 and BaSO3 on passing through lime water or baryta water. However on passing CO2 in excess, the white turbidity of insoluble carbonates dissolve to give a clear solution again due to the formation of soluble bicarbonates, CaCO3 H2O + CO2 Ca(HCO3)2 (2) Hydrides (i) Except Be, all alkaline earth metals form hydrides (MH2) on heating directly with H2. M+ H2 MH2. (ii) BeH2 is prepared by the action of LiAlH4 On BeCl2 2BeCl2 + LiAlH4 2BeH2 + LiCl + AlCl3. (iii) BeH2 and MgH2 are covalent while other hydrides are ionic. (iv) The ionic hydrides of Ca, Sr, Ba liberate H2 at anode and metal at cathode. fusion CaH2 Ca2+ + 2H– – Anode : 2H H2 + 2e– Cathode : Ca2+ + 2e– Ca (v) The stability of hydrides decreases from Be to Ba. (vi) The hydrides having higher reactivity for water, dissolves readily and produce hydrogen gas. CaH2(s) + 2H2O Ca(OH) 2 + 2H2 (3) Carbonates and Bicarbonates (i) All these metal carbonates (MCO3) are insoluble in neutral medium but soluble in acid medium. These are precipitated by the addition of alkali metal or ammonium carbonate solution to the solution of these metals. Beryllium chloride obtained from oxide is however, conveniently 870 1070 K BeO C Cl 2 BeCl 2 CO (ii) BeCl2 is essentially covalent, the chlorides MgCl2, CaCl2 , SrCl2 and BaCl2 are ionic; the ionic character increases as the size of the metal ion increases. The evidence is provided by the following facts, (a) Beryllium chloride is relatively low melting and volatile whereas BaCl2 has high melting and stable. (b) Beryllium chloride is soluble in organic solvents. (iii) The halides of the members of this group are soluble in water and produce neutral solutions from which the hydrates such : MgCl2 6H2O, CaCl2.6H2O. BaCl2 2H2O can be crystallised. The tendency to form hydrated halides decreases with increasing size of the metal ions. (iv) BeCl2 is readily hydrolysed with water to form acid solution, BeCl2 + 2H2O Be (OH)2 + 2HCl. (v) The fluorides are relatively less soluble than the chlorides due to high lattice energies. Except BeCl2 and MgCl2 the chlorides of alkaline earth metals impart characteristic colours to flame. CaCl2 SrCl2 Brick red colour colour BaCl2 Crimson colour Grassy green M + S MS ; 3M + 2P M3P2 Structure of BeCl2 : In the solid phase polymeric chain structure with three centre two electron bonding with Be-Cl-Be bridged structure is shown below, Cl Cl In the vapour phase it tends to form a chlorobridged dimer which dissociates into the linear triatomic monomer at high temperature at nearly 1200 K. (5) Solubility in liquid ammonia : Like alkali metals, alkaline earth metals also dissolve in liquid ammonia to form coloured solutions When such a solution is evaporated, hexammoniate, M(NH3)6 is phosphorescent and are 2MS + 2H2O M(OH) 2 + M(HS)2 (10) Nitrates : Nitrates of these metals are soluble in water On heating they decompose into their corresponding oxides with evolution of a mixture of nitrogen dioxide and oxygen. 1 M ( NO 3 )2 MO 2 NO 2 O 2 2 (11) Formation of complexes (i) Tendency to show complex ion formation depends upon smaller size, high nuclear charge and vacant orbitals to accept electron. Since alkaline metals too do not possess these characteristics and thus are unable to form complex ion. (ii) However, Be2+ on account of smaller size forms many complex such as (BeF3)1-, (BeF4)2-. ID formed. MS + dil. acid H2S ; M3P2 + dil. acid PH3 Sulphides are decomposed by water Cl Be while 60 263 pm Cl Be Sulphides on hydrolysis liberate H2S phosphides on hydrolysis evolve phosphine. E3 Cl 202 PM Cl Be 98o 82o (9) Action with sulphur and phosphorus : Alkaline earth metals directly combine with sulphur and phosphorus when heated to form sulphides of the type MS and phosphides of the type M3P2 respectively. D YG U (6) Nitrides (i) All the alkaline earth metals direct combine with N2 give nitrides, M3N2. (ii) The ease of formation of nitrides however decreases from Be to Ba. (iii) These nitrides are hydrolysed water to liberate NH3, M3N2 + 6H2O 3M(OH)2 + 2NH3 (7) Sulphates ST U (i) All these form sulphate of the type M SO4 by the action of H2 SO4 on metals, their oxides, carbonates or hydroxides. M + H2SO4 MSO4 + H2 ; MO+H2SO4 MSO4+H2O MCO3+ H2SO4 MSO4 + H2O+CO2 M(OH)2 + H2SO4 MSO4 + 2H2O (ii) The solubility of sulphates in water decreases on moving down the group BeSO4 and MgSO4 are fairly soluble in water while BaSO4 is completely insoluble. This is due to increases in lattice energy of sulphates down the group which predominates over hydration energy. (iii) Sulphate are quite stable to heat however reduced to sulphide on heating with carbon. MSO4 + 2C MS+2CO2 (8) Action with carbon : Alkaline metals (except Be, Mg) when heated with carbon form carbides of the type MC2 These carbides are also called acetylides as on hydrolysis they evolve acetylene. MC2 + 2H2OM(OH) 2 + C2H2 Anomalous behaviour of Beryllium Beryllium differs from rest of the alkaline earth metals on account of its small atomic size, high electronegativity Be2+ exerts high polarizing effect on anions and thus produces covalent nature in its compounds. Following are some noteworthy difference of Be from other alkaline earth metals, (1) Be is lightest alkaline earth metal. (2) Be possesses higher m.pt. and b.pt than other group members. (3) BeO is amphoteric in nature whereas oxides of other group members are strong base. (4) It is not easily effected by dry air and does not decompose water at ordinary temperature. (5) BeSO4 is soluble in water. (6) Be and Mg carbonates are not precipitated by (NH 4 )2 CO 3 in presence of NH4Cl. (7) Be and Mg salts do not impart colour to flame. (8) Be does not form peroxide like other alkaline earth metals. (9) It does not evolve hydrogen so readily from acids as other alkaline earth metals do so. (10) It has strong tendency to form complex compounds. (11) Be3N2 is volatile whereas nitrides of other alkaline earth metals are non-volatile. Be2C+4H2O2Be(OH)2 + CH4 Mg2C3 + 4H2O 2Mg(OH)2 + C3H6 CaC2 + 2H2O Ca(OH)2 + C2H4 Diagonal relationship of Be with Al Due to its small size Be differs from other earth alkaline earth metals but resembles in many of its properties with Al on account of diagonal relationship. (1) Be2+ and Al3+ have almost same and smaller size and thus favour for covalent bonding. (2) Both these form covalent compounds having low m. pt and soluble in organic solvent. (3) Both have same value of electronegativity (i.e. 1.5). Dolomite (MgCO 3.CaCO 3 ) , (MgSO 4.7 H 2 O) Carnallite Epsomite (epsom (MgCl 2. KCl. 6 H 2 O) salt) Asbestos (CaMg 3 (SiO 3 )4 ), Talc (Mg 2 (Si 2 O5 )2. Mg(OH )2 ). (2) Extraction of magnesium : It is prepared by the electrolysis of fused magnesium chloride which in turn is obtained from carnallite and magnesite. Carnallite (MgCl 2.KCl.6 H 2 O) can’t be directly ID (4) The standard O.P of these elements are quite close to each other ; Be2+=1.69 volts and Al3+= 1.70 volts. 60 (13) Berylium carbide reacts water to give methane whereas magnesium carbide and calcium carbide give propyne and acetylene respectively. Al2O3 + 3C +3Cl2 2AlCl3 + 3CO (15) Both BeCl2 and AlCl3 are soluble in organic solvents and act as catalyst in Friedel –Crafts reaction. (16) Both Be (OH)2 and Al (OH)3 are amphoteric whereas hydroxides of other alkaline earths are strong alkali. (17) The salts of Be and Al are extensively hydrated. (18) BeCl2 and AlCl3 both have a bridged polymeric structure. (19) Be and Al both form fluoro complex ions [BeF4]2– and [AlF6]3– in solution state whereas other members of 2nd group do not form such complexes. Magnesium and its compounds (1) Ores of magnesium : Magnesite (MgCO 3 ), E3 (12) It’s salts can never have more than four molecules of water of crystallization as it has only four available orbitals in its valence shell. (5) Both become passive on treating with conc. HNO3 in cold. the water of crystallisation cannot be removed by heating. Moreover, strong heating may change it to MgO. U (6) Both form many stable complexes e.g. (BeF3)–, (AlH4)–. converted into anhydrous MgCl 2 by heating because all D YG (7) Like BeO, Al2O3 is amphoteric in nature. Also both are high melting point solids. Al2O3 + 2NaOH 2NaAlO2 + H2O Al2O3 + 6HCl 2AlCl3 + 3H2O (8) Be and Al both react with NaOH to liberate H2 forming beryllates and alluminates. Be + 2NaOH Na2BeO2+H2 2Al + 6NaOH 2Na3AlO3 + 3H2 (9) Be2 C and Al4C3 both give CH4 on treating with water. U Be2C+ 2H2OCH4 + 2BeO Al4C3 + 6H2O3CH4 + 2Al2O3 ST (10) Both occur together in nature in beryl ore, 3BeO. Al2O3. 6SiO2. (11) Unlike other alkaline earths but like aluminium, beryllium is not easily attacked by air (Also Mg is not attacked by air) (12) Both Be and Al react very slowly with dil. HCl to liberate H2. (13) Both Be and Al form polymeric covalent hydrides while hydrides of other alkaline earth are ionic. (14) Both BeCl2 and AlCl3 are prepared is similar way. BeO+ C+ Cl2 BeCl2 + CO MgCl 2 2 H 2 O MgO 2 HCl H 2 O from In Dow’s process, magnesium chloride is obtained sea water as MgCl 2.6 H 2 O. It is rendered anhydrous by heating it in a current of dry HCl gas. The anhydrous magnesium chloride is fused with NaCl (to provide conductivity to the electrolyte and to lower the fusing temperature of anhydrous MgCl 2 ) and then electrolysed at 700 o C. (3) Compounds of magnesium (i) Magnesia (MgO) : It is used as magnesia cement. It is a mixture of MgO and MgCl 2. It is also called Sorel's cement. (ii) Magnesium hydroxide : It aqueous suspension is used in Medicine as an antacid. Its medicinal name is milk of magnesia. (iii) Magnesium sulphate or Epsom salt (MgSO 4. 7 H 2 O) : It is isomorphous with ZnSO 4. 7 H 2 O. It is used as a purgative in medicine, as a mordant in dyeing and as a stimulant to increase the secretion of bile. (iv) Magnesium chloride (MgCl 2.6 H 2 O) : It is a deliquescent solid. Hydrated salt on heating in air undergoes partial hydrolysis. Heat MgCl. 6 H O Mg(OH )Cl HCl 5 H O. 2 2 Calcium and its compounds 2 (1) Ores of calcium : Lime stone or marble or chalk Dolomite (CaCO 3 ), Gypsum (CaSO 4. 2H 2 O), (CaF2 ), phosphorite Ca 3 (PO4 )2. Calcium phosphate is a constituent of bones and teeth. (2) Manufacture : It is manufactured by the electrolysis of a molten mixture of calcium chloride containing some calcium fluoride. Calcium chloride is obtained as a by product of the solvay process. Gypsum when heated to about 200 o C is converted into anhydrous calcium sulphate. The anhydrous form (anhydrite) is known as dead burnt plaster because it does not set like plaster of paris when moistened with water. (v) Calcium Hydroxide Ca(OH )2 (slaked lime) CaO H 2 O Ca(OH )2 (3) Compounds of calcium (i) Calcium oxide or Quick lime or Burnt lime (CaO) : It's aqueous suspension is known as slaked lime. Ca(OH )2 CO2 CaCO 3 Ca(HCO3 )2 Suspension of Ca(OH )2 in water is called milk of lime. When exposed to oxy-hydrogen flame, it starts emitting light called lime light. (vi) Cement : (a) It is essentially a mixture of lime stone and clay. It is also called Portland cement because in presence of water it sets to a hard stone-like mass resembling with the famous Portland rock, a famous building stone of England. The approximate composition of cement is ID CaO is used as basic flux, for removing hardness of water, as a drying agent (for NH 3 gas) for preparing E3 Ca(OH )2 Cl 2 CaOCl 2 H 2 O hissing sound CaO H 2 O Ca(OH )2 Heat, slaked lime mortar (CaO+ sand +water). Calcium oxide (CaO ) 50 – 60 % Silica (SiO 2 ) 20 – 25% Alumina ( Al 2 O 3 ) 5 – 10% Magnesia (MgO ) 1 – 3% D YG U Mortar : Mortar used in making buildings is a mixture of lime (CaO) and sand in the ratio 1 : 3 with enough water to make a thick paste. When the mortar is placed between bricks, it slowly absorbs CO2 from the air and the slaked lime revers to CaCO3. Ca(OH )2 (s) CO 2 (g) CaCO 3 (s) H 2 O(l) Although the sand in the mortar is chemically inert, the grains are bound together by the particles of calcium carbonate and a hard material results. (ii) Calcium chloride (CaCl 2.6 H 2 O) : Fused CaCl 2 is a good dessicant (drying agent). It can't be used to dry alcohol or ammonia as it forms additional products with them. (iii) Calcium carbonate (CaCO3) : U Ca(OH )2 CO 2 CaCO 3 H 2 O. It is insoluble in water but dissolves in the presence of CO 2 due to the formation of calcium ST bicarbonate. CaCO 3 H 2 O CO 2 Ca(HCO 3 )2 It is a constituent of protective shells of marine animals. (iv) Gypsum (CaSO 4. 2 H 2 O) : On partially dehydrates to produce plaster of paris. oC 1 1 120 CaSO 4. 2 H 2 O CaSO 4. H 2 O 1 H 2 O 2 2 Gypsum Plaster of paris Plaster of paris : 1 Hardening H 2O H 2 O CaSO 4. 2 H 2 O CaSO 4.2 H 2 O 2 Setting orthorhomb ic Monoclinic (gypsum) Plaster of paris CaSO 4. dead burnt plaster Gy psum 60 (CaCO 3. MgCO 3 ), Fluorspar o 200 C CaSO 4 (anhydrous ) CaSO 4.2 H 2 O Ferric oxide (Fe 2 O 3 ) 1 – 3% The above compounds are provided by the two raw materials, namely lime stone (which provides CaO ) and clay which provides SiO 2 , Al 2 O 3 and Fe 2 O 3. In cement, almost entire amount of lime is present in the combined state as calcium silicates (2CaO.SiO 2 and 3CaO.SiO 2 ) and calcium aluminates (3CaO. Al 2 O 3 and 4 CaO. Al 2 O 3 ). (b) Cement containing excess amount of lime cracks during setting; while cement containing less amount of lime is weak in strength. (c) Cement with excess of silica is slow-setting and that having an excess of alumina is quick-setting. (d) Cement containing no iron oxide is white but hard to burn. Cement is manufactured by two processes, viz, wet and dry. A small amount (2–3%) of gypsum is added to slow down the setting of the cement so that it gets sufficiently hardened. Setting of cement is an exothermic process and involves hydration of calcium aluminates and calcium silicates. Boron Family Group 13 of long form of periodic table (previously reported as group III A according to (1) Electronic configuration Electronic Element 2 ( ns np ) [ Ne ] 3 s 2 3 p 1 Al [ Ar ] 3 d 10 4 s 2 4 p 1 31 Ga (9) Oxidation state (i) All exhibit +3 oxidation state and thus complete their octet either by covalent or ionic union. [Kr] 4 d 10 5 s 2 5 p 1 In [ Xe ] 4 f 14 5 d 10 6 s 2 6 p 1 81 Tl Boron : Borax (Tincal) (Na2B4O7.10H2O), Colemanite (Ca2B6O115H2O) calcite D YG Boronatro (CaB4O7.NaBO2.8H2 O), Kernite (Na2B4O7.4H2O), Boric acid Aluminium (Al2O3.H2O), (Na3AlF6). : Bauxite Corundum (Al2O3. (H3BO3) (Al2O3), 2H2O), (ii) Boron being smaller in size cannot lose its valence electrons to form B3+ ion and it usually show +3 covalence. The tendency to show +3 covalence however decreases down the group even Al shows +3 covalence in most of its compounds. U (2) Occurrence : The important of this group elements are given below, Boracite (2Mg3B8O15.MgCl2), (8) The atomic radius of Ga is slightly lesser than of Al because in going from Al to Ga, the electrons have already occupied 3d sub shell in Ga. The screening effect of these intervening electrons being poor and has less influence to decrease the effective nuclear charge, therefore the electrons in Ga experience more forces of attractions towards nucleus to result in lower size of Ga than Al ID 49 (7) The atomic radii of group 13 elements are smaller than the corresponding s-block elements. This is due to the fact that when we move along the period, the new incoming electron occupy the same shell whereas the nuclear charge increases regularly showing more effective pull of nucleus towards shell electrons. This ultimately reduces the atomic size. E3 [He ] 2 s 2 2 p 1 5B 13 configuration 1 electrons in its penultimate shell) than in B (it has 2 electrons in its penultimate shell) 60 Mendeleefs periodic table) includes boron (B) ; aluminium (Al) , gallium (Ga), indium (In) and thallium (Tl) Boron is the first member of group 13 of the periodic table and is the only non-metal of this group. The all other members are metals. The non-metallic nature of boron is due its small size and high ionisation energy. The members of this family are collectively known as boron family and sometimes as aluminium family. and Diaspore Cryolite Physical properties U (1) A regular increasing trend in density down the group is due to increase in size. ST (2) Melting points do not vary regularly and decrease from B to Ga and then increase. (3) Boron has very high melting point because it exist as giant covalent polymer in both solid and liquid state. (4) Low melting point of Ga (29.80C) is due to the fact that consists of only Ga2 molecule; it exist as liquid upto 20000C and hence used in high temperature thermometry. (5) Boiling point of these elements however show a regular decrease down the group. (6) The abrupt increase in the atomic radius of Al is due to greater screening effect in Al (it has 8 (iii) Lower elements also show +1 ionic state e.g Tl +, Ga+. This is due to inert pair effect. The phenomenon in which outer shell ‘s’ electrons (ns2) penetrate to (n-1) d-electrons and thus become closer to nucleus and are more effectively pulled the nucleus. This results in less availability of ns2 electrons pair for bonding or ns2 electron pair becomes inert. The inert pair effect begins after n 4 and increases with increasing value of n. (iv) The tendency to form M+ ion increases down the gp. Ga+1 < Tl+1 (10) Hydrated ions : All metal ions exist in hydrated state. (11) Ionisation energy (i) Inspite of the more charge in nucleus and small size, the first ionisation energies of this group elements are lesser than the corresponding elements of s block. This is due to the fact that removal of electron from a p-orbitals (being far away from nucleus and thus less effectively held than s-orbitals) is relatively easier than s-orbitals. (ii) The ionisation energy of this group element decrease down the group due to increases in size like other group elements. (iii) However, ionisation energy of Ga are higher than that of Al because of smaller atomic size of Ga due to less effective shielding of 3d electrons in Ga. Thus (ii) Boron is semi metal, more closer to nonmetallic nature whereas rest all members are pure metals. (iii) Furthermore, these elements are less electropositive than s-block elements because of smaller size and higher ionisation energies. (13) Oxidation potential (i) The standard oxidation potentials of these element are quite high and are given below, B E0op for M M3++ 3e +1.26 – E0op for M M+ + e +0.34 – Al +1.66 +0.55 Ga In +0.56 – Tl +0.34 +0.18 (2) Reactivity towards air (i) Pure boron is almost unreactive at ordinary temperature. It reacts with air to form B2O3 when heated It does react with water. Al burns in air with evolution of heat give Al2O3. (ii) Ga and In are not effected by air even when heated whereas Tl is little more reactive and also form an oxide film at surface. In moist air, a layer of Tl (OH) is formed. (iii) Al decomposes H2O and reacts readily in air at ordinary temperature to form a protective film of its oxides which protects it from further action. (3) Oxides and hydroxides (i) The members of boron family form oxide and hydroxides of the general formula M2O3 and M (OH)3 respectively. (ii) The acidic nature of oxides and hydroxides changes from acidic to basic through amphoteric from B to Tl. B2O3 and B(OH)3> Al2O3 and Al(OH)3 > ID (ii) However Boron does not form positive ions in aqueous solution and has very low oxidation potential. (iii) The higher values of standard oxidation potentials are due to higher heats of hydration on account of smaller size of trivalent cations. (iv) Aluminium is a strong reducing agent and can reduce oxides which are not reduced even by carbon. This is due to lower ionisation energy of aluminium than carbon. The reducing character of these elements is Al > Ga > In > Tl. (14) Complex formation : On account of their smaller size and more effective nuclear charge as well as vacant orbitals to accept elements, these elements have more tendency to form complexes than-s block elements. Chemical properties (1) Hydrides (i) Elements of group 13 do not react directly with hydrogen but a number of polymeric hydrides are known to exist. (ii) Boron forms a large no. of volatile covalent hydrides, known as boranes e.g. B2H6,B4H10,B5H11,B6H10 Two series of borones with general formula B nHn+4 and BnHn+6 are more important. (iii) Boranes are electron deficient compounds. It is important to note that although BX3 are well known, BH3 is not known. This is due of the fact that hydrogen atoms in BH3 have no free electrons to form p–p back bonding and thus boron has incomplete octet and hence BH3 molecules dimerise to form B6H6 having covalent and three centre bonds. (iv) Al forms only one polymeric hydride (AlH3)n commonly known as alane It contains Al…..H……Al bridges. ether 4 LiH AlCl 3 Li[ AlH 4 ] 3 LiCl 60 (i) Electropositive character increases from B to Tl. (v) Al and Ga forms anionic hydrides e.g. LiAlH4 and LiGa H4, E3 valence shell exert more effective nuclear charge in Ga to show higher ionisation energies. (12) Electropositive character ST U D YG U (acidic) Ga2O3 (amphoteric) and Ga(OH)3> In2O3 In (OH)3> Tl2O3 Tl(OH)3 (amphoteric) (basic) (strong basic) B(OH)3 or H3BO3 is weak monobasic Lewis acid. (iii) Boric acid, B(OH)3 is soluble in water as it accepts lone pair of electron to act as Lewis acid. Rest all hydroxides of group 13 are insoluble in water and form a gelatinous precipitate. B(OH)3 + H2O B(OH)41–+H+ (iv) Al2O3 being amphoteric dissolves in acid and alkalies both. Al2O3 + 3H2SO4 Al2 (SO4)3 + 3H2O fuse Al 2 O 3 2 NaOH 2 NaAlO 3 Sodium meta aluminate H 2O (v) One of the crystalline form of alumina (Al2O3) is called corrundum. It is very hard and used as abrasive. It is prepared by heating amorphous form of Al2O3 to 2000 K. (4) Action of Acids (i) Boron does not react with non oxidizing acids, however, it dissolves in nitric acid to form boric acids. (ii) Al, Ga and In dissolve in acids forming their trivalent cations; however, Al and Ga become passive due to the formation of protective film of oxides. (iii) Thallium dissolves in acids forming univalent cation and becomes passive in HCl due to the formation of water insoluble TICl. 2B + 6NaOH (fused) 2Na3BO3 + 3H2 (ii) Al and Ga dissolves in fused as well as in aqueous alkalies, 2Al + 2 NaOH + 2H2O 2NaAl O2 + 3H2 (iii) Indium remains unaffected in alkalies even on heating. (6) Halides (i) All the group 13 elements from the trihalides, MX3 on directly combining with halogens. M + X2 MX3 (ii) All the trihalides of group 13 elements are known except Tl (III) iodide. (iii) Due to small size and high electronegativity of boron, all boron halides are covalent and Lewis acids. These exist as monomeric molecules having plane triangular geometry (sp2 hybridization). BX3+ 3H2O B(OH)3 + 3HX; [X=Cl, Br, I] However, BF3 forms as addition product with H2 O water,. H3O+ [BF3OH]- D YG BF3 having less tendency for hydrolysis as well as Lewis acid nature, is extensively used as a catalyst in organic reactions e.g. Friedel- Crafts reaction. (v) Boron atom, in BX3, has six electrons in the outermost orbit and thus it can accept a pair of electrons form a donor molecule like NH3 to complete its octet. Hence boron halides act as very efficient Lewis acids. The relative Lewis acid character of boron trihalides is found to obey the order ; BI3>BBr3>BCl3>BF3. U However, the above order is just the reverse of normally expected order on the basis relative electronegativities of the halogens. Fluorine, being the most electronegative, should create the greatest electron deficiency on boron and thus B in BF3 should accept electron pair from a donor very rapidly than in other boron trihalides. But this is not true. ST (vi) Lewis acid character of halides of the group 13 elements decreases in the order, B > Al > Ga > In. (vii) Boron halides form complex halides of the type, [BF4–], in which boron atom extends its coordination number to four by utilising empty porbital. It cannot extend its coordination number beyond four due to non availability of d-orbitals. However, the other trihalides of this group form complex halides of the type (AlF6)3–, (GaCl6)3– and (InCl6)3–, etc where the central atom extends its coordination number to 6 by the use of d-orbitals. (viii) The fluorides of Al, Ga In and Tl are ionic and have high melting points. The high melting points of metal fluorides can be explained on the basis that their cations are sufficiently large and have vacant dorbitals for attaining a coordination number of six towards the relatively small fluorine atom. U BF3 + H2OH+ [BF3OH]- Resonance in boron trifluoride is also evidenced by the fact that the three boron-fluorine bonds are indentical and are shorter than the usual single boronfluorine bond As a result of back bonding, the electron deficiency of boron is reduced and hence Lewis acid nature is decreased. The tendency for the formation of back bonding (p- p bond) is maximum in BF3 and decreases very rapidly from BF3 to BI3 This is probably due to the fact that overlapping of the vacant 2p orbitals of boron cannot take place easily with the porbitals of high energy levels (3p in Cl, 4p in Br and 5p in iodine). Thus BI3Br3 and BCl3 are stronger Lewis acids than the BF3. ID (iv) All Boron trihalides except BF3 are hydrolysed to boric acid. bonding or back donation. Thus the B- F bond has some double bond character. Back bonding may take place between boron and of the three fluorine atoms and thus boron trifluoride is regarded as a resonance hybrid of some structures. 60 (i) Boron dissolves only in fused alkalies, E3 (5) Action of Alkalies This anomalous behaviour has been explained on the basis of the relative tendency of the halogen atom to back-donate its unutilised electrons to the vacant p orbitals of boron atom. In boron trifluoride, each fluorine has completely filled unutilised 2p orbitals while boron has a vacant 2p orbital. Now since both of these orbitals belong to same energy level (2p) they can overlap effectively as a result of which fluorine electrons are transferred into the vacant 2p orbital of boron resulting in the formation of an additional p–p bond. This type of bond formation is known as back (ix) Other halides of Al, Ga, In and Tl are largely covalent in anhydrous state and possess low melting point. These halides do not show backbonding because of increases in the size of the element. However, the make use of vacant p-orbitals by co-ordinate bond i.e. metal atoms complete their octet by forming dimers. Thus aluminium chloride, aluminium bromide and indium iodide exist as dimers, both in the vapour state and in non-polar solvents. The dimer structure for Al2Cl6 is evidenced by the following facts, (a) Vapour density of aluminium chloride measured at 4000C corresponds to the formula Al2Cl6. (b) Bond distance between aluminium chlorine bond forming bridge is greater (2.21Å) than the distance between aluminum-chlorine bond present in the end (2.06 Å). The dimeric structure disappears when the halides are dissolved in water This is due to high heat of hydration which split the dimeric structure into [M(H2O)6]3+ and 3X– ions and the solution becomes good conductor of electricity. (13) Boron combines with metals to give borides e.g. Mg3B2. Other members form simply alloys. Al2Cl6 + 2H2O 2[Al(H2O)6]3++6Cl– ; Therefore Al2Cl6 is ionic in water. (14) Concentrated nitric acid oxidises boron to boric acid but no such action is noticed other group members. (x) BF3 and AlCl3 acts as catalyst and Lewis acid in many of the industrial process. Anomalous Behaviour of Boron relationship between Boron and Due to its small size and similar charge/mass ratio, boron differs from other group 13 members, but it resembles closely with silicon, the second element of group 14 to exhibit diagonal relationship. Some important similarities between boron and silicon are given below, (1) Both boron and silicon are typical non-metals, having high m.pt. b.pt nearly same densities (B=2.35gml–1 S=2.34 g//ml). low atomic volumes and bad conductor of current. However both are used as semiconductors. (2) Both of them do not form cation and form only covalent compounds. ID Like Li and Be, Boron – the first member of group 13 also shows anomalous behaviour due to extremely low size and high nuclear charge/size ratio, high electronegativity and non-availability of d electrons. The main point of differences are, Diagonal Silicon 60 Boron halides do not exist as dimer due to small size of boron atom which makes it unable to coordinate four large-sized halide ions. B + 3HNO3 H3BO3 + 3NO2 E3 The dimeric structure may also split by reaction with donor molecules e.g. R3N. This is due to the formation of complexes of the type R3NAlCl3 The dimeric structure of Al2Cl6 exist in vapour state below 473K and at higher temperature it dissociates to trigonal planar AlCl3 molecule. (3) Both exists in amorphous and crystalline state and exhibit allotropy. (2) Boron is a bad conductor of electricity whereas other metals are good conductors. (4) Both possess closer electronegativity values (B=2.0; Si=1.8). (3) Boron shows allotropy and exists in two forms – crystalline and amorphous. Aluminium is a soft metal and does not exist in different forms. (5) Both form numerous volatile hydrides which spontaneously catch fire on exposure to air and are easily hydrolysed. D YG U (1) Boron is a typical non- metal whereas other members are metals. (4) Like other non-metals, the melting point and boiling point of boron are much higher than those of other elements of group 13. (5) Boron forms only covalent compounds whereas aluminium and other elements of group 13 form even some ionic compounds. U (6) The hydroxides and oxides of boron are acidic in nature whereas those of others are amphoteric and basic. ST (7) The trihalides of boron (BX3) exist as monomers On the other hand, aluminium halides exist as dimers (Al2X6). (8) The hydrides of boron i.e. boranes are quite stable while those of aluminium are unstable. (9) Dilute acids have no action on boron Others liberate H2 from them. (6) The chlorides of both are liquid, fume in most air and readily hydrolysed by water. BCl3 + 3H2O B(OH)3 + 3HCl SiCl4 + H2O Si(OH)4 + 4HCl (7) Both form weak acids like H3BO3 and H2SiO3. (8) Both form binary compounds with several metals to give borides and silicide. These borides and silicide react with H3PO4 to give mixture of boranes and silanes. 3Mg+2BMg3B2; Mg3B2+H3PO4 Mixture of boranes (Magnesium boride) 2Mg + Si Mg2Si ; Mg2Si + H3PO4 Mixture of silanes (magnesium silicide) (10) Borates are more stable than aluminates. (9) The carbides of both Boron and silicon (B4C and SiC) are very hard and used as abrasive. (11) Boron exhibit maximum covalency of four e.g., BH–4 ion while other members exhibit a maximum covalency of six e.g., [Al(OH)6]3-. (10) Oxides of both are acidic and can be reduced by limited amount of Mg In excess of Mg boride and silicide are formed. (12) Boron does not decompose steam while other members do so. B2O3+3Mg 3MgO+2B ; SiO2+2Mg 2MgO+Si (11) Both the metals and their oxides are readily soluble in alkalies. 2B + 6NaOH 2Na3BO3 + 3H2 Heat 3Mg + 2B (silicate) B2O3 + 6NaOH 2Na3BO3 + 3H2O SiO2 + 2NaOH Na2SiO3 + H2O Both borates and silicates have tetrahedral structural units BO 4n and SiO 4n respectively. Boro H 3 BO 3. B + 3 HNO3 H 3 BO 3 + 3 NO 2 2B + 3 H 2 SO 4 2 H 3 BO 3 + 3 SO 2 Fused alkalies (NaOH, KOH) forming borates, liberating hydrogen. dissolve boron Fused 2B + 6KOH 2 K3 BO 3 + 3H 2 (4) Uses of Boron : Boron is used in atomic reactors as protective shields and control rods, as a semiconductors for making electronic devices in steel industry for increasing the hardness of steel and in making light composite materials for air crafts. (5) Compounds of Boron (i) Boron Hydrides Boron forms hydrides of the types Bn H n 4 and Bn H n 6 called boranes. Diborane is the simplest boron ID silicates are known in which boron replaces silicon in the three dimensional lattice. Boron can however form planar BO3 units. (12) Acids of both these elements form volatile esters on heating with alcohol in presence of conc. H2SO4. B(OH)3 + 3ROHB(OR)3 + 3H2O Si(OH)4 + 4ROH Si(OR)4 + 4H2O Boron and its compounds Boron is the first member of group –13 (IIIA) of the periodic table. Boron is a non- metal. It has a small size and high ionization energy due to which it can not lose its valence electrons to form B 3 ion. Its compounds especially the hydrides and halides are electron deficient and behave as Lewis acid. (1) Ores of boron (i) Borax or tincal : Na2 B4O7. 10H2O (ii) Kernite or Rasorite : Na2 B4O7. 4H2O (iii) Colemanite : Ca2 B6O11. 5H2O (iv) Orthoboric acid : H3BO3 (It occurs in the jets of steam called soffioni escaping from ground in the volcanic region of the Tuscany). Boron is present to a very small extent (0.001%) in earth’s crust. (2) Isolation : Elemental boron in the form of dark brown powder is obtained either by reduction of boric oxide with highly electropositive metals like K, Mg, Al, Na, etc. in the absence of air and boron halides with hydrogen at high temperature eg. Water, steam and HCl have no action on B. oxidising acids (HNO 3 , H 2 SO 4 ) convert boron to 60 Si + 2NaOH + H2O Na2SiO3 + 2H2 Mg 3 B2 Magnesium boride E3 (borate) hydride which is a dimer of BH 3. Preparation 450 K B 2 H 6 6 LiBF4 (a) 8 BF3 6 LiH D YG U (b) 4 BCl 3 LiAlH 4 2 B 2 H 6 3 AlCl 3 3 LiCl Heat B2O3 + 6K 2B + 3K2O 2BCl3 + 3H2 2B + 6HCl. By thermal decomposition of boron triiodide over red hot tungsten filament and boron hydrides for example, U 1270 K 2BI3 2B + 3I2 ; B2H6 2B + 3H2 (3) Properties : It exists in mainly two allotropic forms i.e. amorphous dark brown powder and crystalline black very hard solid. It occurs in two isotopic forms, i.e., 5 B 10 (20% abundance) and 5 B 11 ST W, heat Heat (80% abundance). With air, boron forms B2 O3 and BN at 973K, with halogens, trihalides (BX 3 ) are formed, with metals borides are formed. eg. Heat 4B+ 3O2 2 B2 O3 Boron trioxide Heat 2B + N 2 2B + 3X 2 2BN Boron nitride 2BX 3 Boron trihalide (c) In the laboratory, it is prepared by the oxidation of sod. Borohydride with I2. Poly ether 2 NaBH 4 I2 B 2 H 6 2 NaI H 2 Properties : (a) Since Boron in boranes never complete its octet of electrons hence all boranes are called as electron-deficient compounds or Lewis acids. (b) All boranes catch fire in the presence of oxygen to liberated a lot of heat energy. Thus, they can also be used as high energy fuels. B 2 H 6 3 O 2 2 B 2 O 3 3 H 2 O; H 1976 KJ / mole (c) Boranes are readily hydrolysed by water. B 2 H 6 6 H 2 O 2 H 3 BO 3 6 H 2 (d) With carbon monoxide B 2 H 6 2CO (BH 3 CO )2 (e) Boranes are used for formation of hydroborates or borohydrides such as LiBH 4 or NaBH 4 , which are extensively used as reducing agents in organic synthesis. Diethy l ether 2 LiH B2 H 6 2 Li [BH 4 ] Diethy l ether 2 NaH B 2 H 6 2 Na [BH 4 ] Structure of diborane : B2 H 6 has a three centre electon pair bond also called a banana shape bond. (a) B H t : It is a normal covalent bond (two centre electron pair bond i.e., 2c 2e ). (b) B Hb : This is a bond between three atoms, (three centre electron pair bond i.e., B H b B, 3 c 2e ). Na 2 B 4 O7.10 H 2 O Na 2 B 4 O7 2 NaBO 2 B 2 O3 Bridged Hydrogen Hb Ht 97o B B Ht 10 H 2O Borax bead is used for the detection of coloured basic radicals under the name borax bead test. Borax bead test : Borax bead is a mixture of NaBO 2 and B2 O3. B2 O3 on heating combines readily Ht with a number of coloured transition metal oxides such as Co, Ni, Cr, Cu, Mn, etc. to form the corresponding metaborates which possess characteristic colours, 120 o or B CoSO 4 CoO SO 3 ; CoO B2 O3 Co (BO 2 )2 H Cobalt meta borate (Blue) t B Colours of some important metaborates are : Cupric metaborate, Cu (BO 2 )2 is dark blue, chromium metaborate, H Hb Ht 60 Hb.... t Cr (BO 2 )2 is green, nickel metaborate, Ni(BO 2 )2 is brown and manganese metaborate, Mn(BO 2 )2 is pink violet. Structure of diborane (B2H6) The other B5 H 9 , B4 H10 , B5 H11 etc. boron hydrides are Heat 2B X 3 (X F, Cl, Br, I) 2B + 3 X 2 while BI 3 is a solid. D YG In these halides, the central boron atom has three shared pairs of electrons with the halogen atoms. Therefore, these have two electrons less than the octet and are electron deficient compounds. They acts as Lewis acids. H F F H | | | | F B : N H F B N H | | | | F H F H Lewis base U The relative acidic strength of boron trihalides decreases as : BI 3 BBr3 BCl 3 BF3. (iii) Borax ( Na 2 B 4 O7. 10 H 2 O ) It occurs naturally as tincal (Suhaga) which contains about 50% borax in certain land, lakes. It is also obtained from the mineral colemanite by boiling it with a solution of Na 2 CO 3. ST gives volatile vapours of triethyl borate which burns with a green edged flame. Na 2 B 4 O7 H 2 SO 4 5 H 2 O Na 2 SO 4 4 H 3 BO 3 H 3 BO 3 3C 2 H 5 OH B(OC 2 H 5 )3 3 H 2 O Triethyl borate This reaction is used as a test for borate radical in qualitative analysis. Uses : (a) In making optical and hard glasses. (b) In the laboratory for borax bead test. (c) In softening of water. (d) In the preparation of medicinal soaps due to its antiseptic character. (iv) Boric acid or orthoboric acid ( H 3 BO 3 ) U BF3 and BCl 3 are gases, BBr3 is a volatile liquid Ca 2 B6 O11 2 Na 2 CO 3 Na 2 B 4 O7 2CaCO 3 2 NaBO 2 Colemanite (c) When heated with C2 H 5 OH and conc. H 2 SO 4 it ID (ii) Boron Halides Boron reacts with halogens on strong heating to form boron halides. Lewis acid Borax bead Ht Hb Ht ( B2 O3 ), E3 Terminal Hydrogen sodium metaborate ( NaBO 2 ) and boric anhydride Borax Properties : (a) Its aqueous solution is alkaline due to hydrolysis, Na 2 B4 O7 + 7 H 2 O 2NaOH+4 H 3 BO 3. (b) On heating borax loses its water of crystallization and swells up to form a fluffy mass. On further heating, it melts to give a clear liquid which solidifies to a transparent glassy bead consisting of It is obtained from borax by treating with dil. HCl or dil. H 2 SO 4 , Na 2 B 4 O7 2 HCl 5 H 2 O 2 NaCl 4 H 3 BO 3 It can also be obtained from the mineral colemanite by passing SO 2 through a mixture of powdered mineral in boiling water, Ca 2 B6 O11 + 4 SO 2 11 H 2 O 2Ca(HSO 3 )2 6 H 3 BO 3 Properties : (a) It is a very weak monobasic acid, does not act as a proton doner but behaves as a Lewis acid i.e. it accepts a pair of electrons from OH ion of H 2 O , H 3 BO 3 H 2 O [B(OH )4 ] H It acts as a strong acid in presence of polyhydroxy compounds such as glycerol, mannitol etc. and can be titrated against strong alkali. (b) With NaOH it forms, sodium metaborate, H 3 BO 3 NaOH NaBO 2 2 H 2 O (c) With C2 H 5 OH and conc. H 2 SO 4 , it gives triethyl borate Conc. H 2 SO 4 B(O C2 H 5 )3 + 3 H 2 O H 3 BO 3 +3 C2 H5 OH (d) Action of heat : The complete action of heat on boric acid may be written as, 373 K H 3 BO 3 HBO 2 Boric acid 433 K Red hot H 2 B 4 O7 B 2 O 3 Metaboric acid Bauxite Boron oxide Tetra boric acid Structure : In boric acid, planar BO 33 units are (Finely powdered) (white) Coke N 2 Silica reduced to Alumina form AIN Heated to Si which volatalis es aluminium nitride o 1800 C Heated Pure Al 2 O3 Al (OH )3 Hydrolysis joined by hydrogen bonds to give a layer structure. Uses : (a) As a food preservative. (b) As a mild antiseptic for eye wash under the name boric lotion. (c) For the preparation of glazes and enamels in pottery. (v) Borazine or Borasole or Triborine triamine ( B3 N 3 H 6 ) (iv) Hall and Heroult process : It is used for extraction of aluminium. In this process a fused mixture of alumina (20%), cryolite (60%) and fluorspar (20%) is electrolysed using carbon electrodes. Whereas cryolite makes Al 2 O3 conducting It is a compound of B, N and H. It is a colourless liquid and is also called inorganic benzene. Aluminium is refined by Hoope's electrolytic process. 60 fluorspar decreases the melting point of alumina. o (3) Compounds of Aluminium (i) Aluminium oxide or Alumina ( Al 2 O3 ) : It 180 C 2 B 2 H 6 6 NH 3 2 B3 N 3 H 6 12 H 2. It has a six membered ring of alternating B and N atoms, each is further linked to a H- atom. B H—N N—H H—B B—H (ii) Aluminium chloride ( Al 2 Cl 6 ) : It is prepared by passing dry chlorine over aluminium powder. N Al 2 O3 3C 3Cl 2 ID H Borazine (vi) Boron nitride (BN) It is prepared by treating BCl 3 with an excess of 750 o C BN + 3HCl. BCl 3 + NH 3 [ H 3 N BCl 3 ] Excess NH 3 D YG It is a colourless, good insulator, diamagnetic and almost unreactive solid Aluminium and its compounds (1) Ores of Aluminium : Bauxite (Al 2 O3.2H 2 O), Cryolite (KAlSi 3 O8 ), (Na 3 AlF6 , Felspar (Al 2 O3. 2SiO 2.2H 2 O) , Mica (K2 O.3 Al 2 O3. 6 SiO 2.2H 2 O), Corundum ( Al 2 O3 ) , Diaspore (Al 2 O3.H 2 O), Alunite or alum stone [K2SO 4. Al 2 (SO 4 )3. 4 Al (OH )3 ]. ST U (2) Extraction : Aluminium is obtained by the electrolysis of the oxide (alumina) dissolved in fused cryolite. This involves following steps, Purification of ore (i) Baeyer's process Bauxite Roasted Caustic soda solution Roasted ore Finely powdered FeOFe2 O3 (red) Filtrate (Sod. Aluminate) High pressure (150 o C, 80 atm) filtered, Fe 2 O3 as residue Pure Al 2 O 3 Al (OH )3 Filtered Heat CO 2 (ii) Hall's process Bauxite CO 3 Na 2 Solution 2 (Finely powdered) Fused, extracted with water. Residue Fe 2 O 3 (red) CO 50 - 60 o C and filtered. Filtrate (Na 2 CO 3 ) Heat Precipitat e Al(OH)3 Pure Al 2 O3 (iii) Serpek's process 2 AlCl 3 3CO(g) (anhydrous ) It exists as dimer Al 2 Cl6 , in inert organic solvents and in vapour state. It sublimes at 100 o C under vacuum. Dimeric structure disappears when AlCl 3 is U NH3 and pyrolysing the resulting mixture in an atmosphere of NH3 at 750°C, Kaolinite occurs in nature as colourless corundum and several coloured minerals like ruby (red), topaz (yellow), Sapphire (blue), amethyst (violet) and emerald (green). These minerals are used as precious stones (gems). E3 H dissolved in water. It is hygroscopic in nature and absorbs moisture when exposed to air. (iii) Thermite : A mixture of aluminium powder and Fe 2 O3 in the ratio 1:3. It is used for welding of iron. The reaction between Al and Fe 2 O3 is highly exothermic, Al Fe 2 O3 Al 2 O3 Fe Heat (iv) Aluminium sulphate [Al2(SO4)3] : It is used for the preparation of alums e.g., potash alum Al 2 (SO 4 )3. K2 SO 4. 24 H 2 O. It is also used for making fire proof clothes. (iv) Alums : In general, the term alum is given to double sulphates of the type M 2 SO 4.M 2 (SO 4 )3.24 H 2 O where NH 4 ,M M Na , K is a univalent cation like is a trivalent cation like Al 3 , Fe 3 and Cr and 3. Some important points to be noted about the alums are (a) General formula is M 2 SO 4.M 2 (SO 4 )3.24 H 2 O M Monovalent metal; M Trivalent metal In alum crystals, 6 water molecules are held by monovalent ion, 6 water molecules are held by trivalent ion, 12 water molecules are held in the crystal structure. (b) All alums are isomorphous. Aqueous solutions of alums are acidic due to cationic hydrolysis of trivalent cation. (c) Double sulphates of divalent ions and trivalent ions with 24 water molecules in their crystals are known as Pseudoalums. MSO 4.M 2 (SO 4 )3.24 H 2 O General formula is M Bivalent metal; M Trivalent metal (d) alums. Pseudoalums are not isomorphous with (e) Feather alum or ‘Hair-salt’ Al 2 SO 4.18 H 2 O is a native form of aluminium sulphate. 60 (f) Potash alum is used for tanning of leather, as mordant in dyeing and calico printing, for sizing paper, as a syptic to stop bleeding and purification of water. Some important alums are Potash alum K 2 SO 4. Al 2 (SO 4 )3.24 H 2 O Ammonium alum (NH 4 )2 SO 4. Al 2 (SO 4 )3.24 H 2 O Chrome alum K 2 SO 4.Cr2 (SO 4 )3.24 H 2 O Carbon Family (1) Electronic configuration 14 Si 32 Ge Sn 82 Pb [ Ne ] 3 s 2 3 p 2 [ Ar ] 3 d 10 4 s 2 4 p 2 [Kr] 4 d 10 5 s 2 5 p 2 [ Xe ] 4 f 14 5 d 10 6 s 2 6 p 2 ST U 50 [He ] 2 s 2 2 p 2 D YG 6C Electronic configuration ( ns 2 np 2 ) U Elements ID Carbon is the first member of group 14 or IVA of the periodic table. It consists of five elements carbon (C), silicon (Si), germanium (Ge), tin (Sn) and lead (Pb). Carbon and silicon are nonmetals, germanium is metalloid and tin and lead are metals. E3 Sodium alum Na 2 SO 4. Al 2 (SO 4 )3.24 H 2 O 722 s and p-Block Elements C Physical properties Ge Non-metals Sn metalloid non-metallic Pb metal metal (2) Abundance : Carbon and silicon are most abundant elements in earth’s crust whereas germanium occurs only as traces. Tin and lead also occur in small amounts. Only carbon occurs in free state as coal, diamond and graphite and in combined state as carbonates, CO2 petroleum and natural gas Silicon is the second most abundant element after oxygen in earth’s crust in form of silicates and silica. Germanium Pb Atomic radius (pm) 144 0.77 111 122 141 Atomic volume (ml) 18.27 3.4 11.4 13.6 16.3 (ii) The atomic radii of group 14 elements are than their corresponding group 13 elements due to increase in nuclear charge in the same period. (iii) Some of the ionic radii involving six coordination of these group elements are given below, C Pb Si Element Pb C Si Sn 2.34 5.32 7.26 11.34 D YG Density (g/ml)3.51 (for diamond) Ge 2.22 (for graphite) (4) Melting point and boiling points (i) The melting point and boiling point of this group members decrease down the group. Element C Si Ge Sn Pb m.pt(K) 4373 1693 1218 505 600 – 3550 3123 2896 U b.pt.(K) 2024 ST (ii) The melting point and boiling point of group 14 elements are however, higher than their corresponding group 13 elements. This is due to the formation of four covalent bonds on account of four electrons in their valence shells which results in strong binding forces in between their atoms in solid as well as in liquid state. (5) Atomic radii and atomic volume (i) Both atomic radii and atomic volume increases gradually on moving down the group due to the effect of extra shell being added from member to member. Sn – – 73 118 Ionic radius (M++) in pm 78 – 40 53 69 (6) Electronegativity : The electronegativity decreases from C to Si and then becomes constant. C Si Ge Sn 2.5 1.8 1.8 Pb U (3) Density : The density of these elements increases down the group as reported below Ge Ionic radius (M2+) in pm 119 ID found in traces in coal and in certain deposits. It important constituent for making conductors and transistors The important ore of tin is tin stone (SnO2) or cassiterite. Lead is found is form of galena (PbS) anglesite (PbSO4) and cerussite (PbCO3) The abundance ratio in earth’s crust is given below, Sn 60 C Si The Ge E3 (1) Non-metallic nature : nature decreases along the group. Si Electronegativity on pauling scale 1.7 1.6 The electronegativity from silicon onwards is almost is almost constant or shows a comparatively smaller decreases due to screening effects of d 10 electrons in elements from Ge onwards. (7) Ionisation energy (i) The ionisation energy decreases regularly down the group; Pb however shows a higher value than Sn due to poor shielding of inner f-orbitals as a result of which effective nuclear charge experienced by outer shell electrons becomes more in Pb. Ionisation energy (kJ mol-1) Pb IE1 C 1086 Si Ge Sn 786 761 708 1577 1537 1411 715 IE2 2352 1450 (ii) The first ionisation energies of group 14 elements are higher than their corresponding group 13 elements because of smaller size. (iii) The electropositive character of these elements increases down the group because of decreases in ionisation energy. s and p-Block Elements (8) Oxidation state (k J/mol) (i) Presence of four electrons in outermost shell of these elements reveals that the members of this family can gain four electrons forming M4+ or M4- ions to show ionic nature or exhibit tetravalent covalent nature by sharing of four electron pairs in order to 723 (kJ/mol) 348 Si–Si 180 C–O 315 Si–O 372 C–H 414 Si–H 339 attain stable configuration. C–Cl 326 Si–Cl 360 (ii) The formation of M4+ or M4- ions require huge amount of energy which is normally not available during normal course of reactions, therefore, these elements usually do not form M4+ or M4- ions, but they usually form compounds with covalence of four. C–F 439 Si–F 536 (iv) Sn2+ and Pb2+ show ionic nature. (v) The tendency to form +2 ionic state increases on moving down the group due to inert pair effect. ID (i) The tendency of formation of long open or closed atom chains by the combination of same atoms in themselves is known as catenation. (i) Enantiotropy : When two forms of a solid substance exist together in equilibrium with each other at a particular temperature under normal pressure it is U called enantiotropy. (ii) The catenation is maximum in carbon and decreases down the group. D YG (iii) This is due to high bond energy of catenation. (iv) Only carbon atoms also form double or triple bonds involving p-p multiple bond within itself. > C = Calkaline earth metal oxides>transition metal oxides. (ii) generally Acidic acidic oxides : Non-metal oxides are - CO 2 , SO 2 , SO 3 , NO 2 , N 2 O5 , P4 O10 , Cl 2 O7 etc. (iii) Amphoteric oxides : Al 2 O3 , SnO 2 etc. (iv) Neutral oxides : H 2 O, CO, N 2 O, NO etc. s and p-Block Elements MgO strongl y basic basic Cl 2 O7 SO 2 Al 2 O3 SiO 2 ampho teric weakly acidic P4 O10 very strongl y acidic strongl y acidic acidic Basic to acidic character increases However, on moving down a group, acidic character of the oxides decreases. For example in the third group, the acidic character of oxides decreases as: B2 O3 Al 2 O3 Ga 2 O 3 In2 O3 , Tl 2 O3 acidic amphoteric (weakly basic) basic Acidic to basic character increases 2 O 3 H 285.4 kJ discharge Ozone is prepared in the laboratory by the following two types of ozonisers, (a) Siemen’s ozoniser, (b) Brodie’s ozoniser For the better yield of ozone : (a) Only pure and dry oxygen should be used. (b) The ozoniser must be perfectly dry. (c) A fairly low temperature ( 273 K) must be maintained. (d) The electric discharge must be sparkless. Physical properties : Ozone is a light blue coloured gas, having pungent odour. It is heavier than air. Its vapour density is 24. It is slightly soluble in water. Chemical properties : The important chemical properties of ozone are discussed below, (1) Decomposition : Pure ozone decomposes on heating above 475 K to form O 2 gas. U ID On the basis of oxygen content the oxides may be classified into the following types, Normal oxides : These contain oxygen atoms according to the normal oxidation number i.e. – 2. For example, MgO, H 2 O, CaO, Li2 O, Al 2 O3 etc. Silent electric 3O2 60 Na 2 O i.e. freon which is increasingly being used in aerosols and as a refrigerant. Preparation : Ozone is prepared by passing silent electric discharge through pure, cold and dry oxygen in a specially designed apparatus called ozoniser. The formation of ozone from oxygen is an endothermic reaction. E3 Trends of oxides in the periodic Table : On moving from left to the right in periodic table, the nature of the oxides change from basic to amphoteric and then to acidic. For example, the oxides of third period has the following behaviour, 741 D YG Polyoxides : These contain oxygens atoms more than permitted by the normal valency. Therefore, these contain oxygen atoms in oxidation state different than –2. Peroxides : These contains O 22 ion having oxidation number of oxygen as –1. For example, H 2 O2 , Na 2 O2 , BaO 2 , PbO 2 etc. Superoxides : These contains O 2 ion having oxidation number of oxygen as –1/2. For example, KO 2 , PbO 2 , etc. U Suboxides : These oxides contain less oxygen than expected from the normal valency. For example, N 2 O. ST Mixed oxides : These oxides are made up of two simple oxides. For example, red lead magnetic oxide of iron, Pb3 O4 (2PbO 2 PbO 2 ), Fe 3 O4 (FeO Fe 2 O3 ) and mixed oxide of manganese, Mn 3 O4 (MnO 2 2 MnO ). Ozone or trioxygen Ozone is an allotrope of oxygen. It is present in the upper atmosphere, where it is formed by the action U. V. radiation 2O 3. of U. V. radiations on O 2 , 3 O 2 Ozone O 3 protects us from the harmful U. V. radiations which causes skin cancer. Now a days, ozone layer in the atmosphere is depleting due to NO released by supersonic aircrafts and chlorofluoro carbons (CFC’S) 475 K 2O 3 3 O 2 H 285.4 kJ (2) Oxidising agent : Ozone is one of the most powerful oxidising agent with the liberation of dioxygen. In fact, ozone is a stronger oxidising agent than molecular oxygen because ozone has higher energy content and decomposes to give atomic oxygen as: O3 O2 O Atomic oxy gen Therefore, ozone oxidises a number of nonmetals and other reducing agents. e.g. 2 Ag O3 Ag 2 O O 2 ; S 3O3 SO 3 3O2 Metal Silver oxide Non metal PbS 4 O 3 PbSO 4 4 O 2 Compound Mercury is oxidised to mercurous oxide, 2 Hg O3 Hg 2 O O 2 Mercurous oxide During this reaction mercury loses its meniscus and starts sticking to the sides of the glass. This is known as tailing of mercury. Mercurous oxide formed in this reaction dissolves in mercury and starts sticking to the glass surface. (3) Bleaching agent : Due to the oxidising action of ozone, it acts as a mild bleaching agent as well as a steril