Chapter 13_ Cardiac Function- Part 1.docx
Document Details
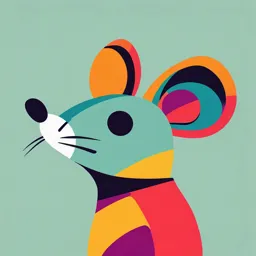
Uploaded by AppreciatedTurkey
Full Transcript
Chapter 13: Cardiac Function Overview of the Cardiovascular System The cardiovascular system includes: the heart the blood and the blood vessels. The heart is the pump (composed of cardiac muscle) that moves the blood through the blood vessels. It has both sensory and endocrine functions for the reg...
Chapter 13: Cardiac Function Overview of the Cardiovascular System The cardiovascular system includes: the heart the blood and the blood vessels. The heart is the pump (composed of cardiac muscle) that moves the blood through the blood vessels. It has both sensory and endocrine functions for the regulation of blood pressure and blood volume. The blood vessels serve as the tubular conduit system for blood transport in the body. They have a sensory function, and they also act as an effector organ that regulates blood distribution and blood pressure. The blood is the connective tissue fluid that circulates throughout the body carrying materials (nutrients and waste) to and from cells. It also facilitates communication between organs and systems by transporting hormones throughout the body. The cardiovascular system is an organ system because it consists of multiple organs working together for a common purpose. It is a closed system, meaning that it is completely internal and not open to the external environment. Its primary purpose is to control the process of delivery and removal of molecules to and from the body to meet its demands. This process is highly regulated by the nervous and endocrine systems, and the cardiovascular system is highly coordinated with the other systems in the body. Recall that cellular materials must be transferred between the internal and external environments. Everything that comes into the body, such as oxygen, must come from the external environment; everything that leaves the body, such as carbon dioxide, must somehow be transferred from the internal to the external environment. Diffusion only works over extremely small distances, so it is crucial that the blood vessels circulate blood throughout the body so that nutrients and gases can be exchanged. Each of the components of the cardiovascular system has a simple function. For example, the heart pumps blood, and the blood vessels provide an avenue for blood to circulate. However, these organs also have a multitude of other, more complex functions. We will discuss them in greater detail below. Some Basic Terminology It will help you to understand some basic terminology before we begin discussing the anatomy of the heart: An artery is a blood vessel that always carries blood away from the heart. A vein is a blood vessel that carries blood to the heart. An atrium is a chamber of the heart that receives blood. A ventricle is a chamber of the heart that expels blood. Anatomy of the Heart According to Dr. Nguyen, the heart is "basically an upside-down, backward-leaning cone." It is covered and lined with epithelial tissue. The apex (pointed top) of the heart points down and to the left (which is why the heart is said to be on the left side of your body). The bottom of the heart is fused to the diaphragm by parts of the pericardium, which is a serous membrane (a double-layered membrane with a small amount of fluid separating the layers). This surrounds the heart and helps lubricate it, because the heart's movements would otherwise create friction with surrounding organs, such as the lungs. You should familiarize yourself with the following diagram at the heart, as the anatomical parts will be used to help explain the physiological processes. Students are taught to pledge allegiance to the flag by putting their hand over their heart. If they wanted to be anatomically correct, they would rotate their hand so that their fingers are pointing somewhat down and to the left, reflecting the oblique placement of the heart. If they wanted to be even more anatomically correct, they would peel the tips of their fingers oft the chest a bit, indicating that the apex of the heart lies anterior to the heart's base. The heart has four chambers, divided into atria (singular: 'atrium')The heart has four chambers, divided into atria and ventricles. The atria receive blood from the vessels, and the ventricles pump blood from the heart. The walls of the ventricles are significantly thicker than the walls of the atria. There are two types of valves associated with the heart: the atrioventricular valves (AV valves) and the semilunar valves, which each function to establish and maintain unidirectional blood flow: Atrioventricular valves - The atrioventricular valves are flops of connective tissue that separate the atrium and ventricle on each side of the heart, and they permit the blood to flow from the atrium into the ventricle when the pressure in the heart causes them to open or close. When atrial pressure is higher than ventricular pressure, the valves open because the blood is moving from high to low pressure. The AV valve on the left side of the heart has two flaps (cusps), so it is referred to as the bicuspid or mitral valve. The valve on the right is the tricuspid valve. The valves are held in place by strong chordae tendineae, strands of connective tissue that anchor the cusps to the papillary muscles and prevent prolapse (in which the cusps are pushed into the atria). When the papillary muscles contract, they pull on the chordae tendineae, which prevents prolapse. Semilunar valves - The semilunar valves are thicker connective tissues ("cusps") that separate the ventricles from the arteries. The aortic semilunar valve is located between the aorta and the left ventricle, whereas the pulmonary semilunar valve separates the right ventricle from the pulmonary trunk, which leads to the lungs. These valves open when ventricular pressure is greater than arterial pressure and close when the ventricles relax and stop contracting. They are much more cartilaginous than the floppy AV valves. EXAM TIP: To remember that the atria receive blood from the body, remember that an atrium of a building is the room In which guests are received. When you enter into a building, you enter Into the atrium. The heart is a hollow organ, with three physical layers forming its walls: The myocardium is the thickest layer of cardiac muscle tissue, found in the middle of the wall. This layer is responsible for the heart's ability to contract and produce a pumping force, with cardiac myocytes forming the bulk of the tissue. The endocardium, or endothelium, is the inside lining of the heart, and is an arrangement of simple squamous epithelial tissue. This layer is continuous with the endothelium lining the blood vessels in the body. The epicardium, or the visceral pericardium, forms the top layer of the heart and is also formed from simple squamous epithelial tissue. The pericardium is a 'sac' that sits around the heart and helps to ensure that the heart stays in position within the mediastinum. Another function of the pericardium is to ensure that the heart does not rub against the lungs as it is pumping blood. The pericardium contains serous fluid, which is oily and allows for the organs to slide by each other without causing any friction. You might compare the pericardium to a pillowcase. Imagine taking a pillow out of its pillowcase and laying the pillowcase on your bed. The space where you can slide your hand into is what would be the pericardial cavity, which is where the serous fluid would be contained. The part of the pillowcase that is touching the bed would be called the visceral pericardium, which is the layer that is directly adhered to your heart. The side of the pillow case which is not touching the bed would be the parietal pericardium, which would be the layer that is touching the body wall. Just as the two sides of a pillowcase are connected at one end, the parietal and visceral pericardia are connected as well. There is a third layer of the pericardium which is not a port of the serous pericardium, and it is known as the fibrous pericardium. The fibrous pericardium is what helps the heart to stay in place in the mediastinum. The myocardial layer is thicker in some places around the heart than others. The thicker the myocardial wall, the higher contractile ability that part of the heart has, and the more force it can generate. The atria have thinner walls than the ventricles because they don't have to do as much work-they are located at the top part of the heart, so blood flows from the atria to the ventricles with the help of gravity. The ventricles, on the other hand, are working against gravity and must pump blood out of the heart and over longer distances, so their myocardial walls are thicker. The myocardial wall is thicker in the left ventricle than in the right ventricle because the right ventricle has to pump blood to the lungs (which are nearby), while the left ventricle has to pump blood throughout the entire body. Note that a thicker muscle has more muscle fibers and thus has the capacity to form more crossbridges. Recall from our discussion of muscles that the number of crossbridges that a muscle forms is the key determinant of its ability to generate tension. Anatomy of a Blood vessel The blood vessels of the body are collectively referred to as the body's vasculature. They are divided into three main types: Veins carry blood towards the heart and have a much greater proportion of connective tissue than arteries. Veins have a much larger lumen compared to arteries, and they also contain valves which help to prevent backflow. Arteries carry blood away from the heart and have a much thicker smooth muscle layer than veins do. The smooth muscle in arteries allows for vasoconstriction and vasodilation. Since arteries have a thicker layer of smooth muscle, their lumens are much smaller than the lumen in veins. Capillaries are the smallest vessels of all. They usually carry blood between arteries and veins. Note that capillaries are not arteries or veins, but rather their own separate type of vessels. Blood vessels are, by definition, hollow organs, and are composed of three layers-the endothelium inner lining, the thick smooth muscle wall, and the connective tissue which covers them and anchors them in place. Capillaries only have two thin layers-endothelium (simple, squamous epithelium) and the basement membrane connective tissue. They lack smooth muscle. Blood flows from an artery to an arteriole, then through a network of capillaries to a venule. The blood then goes back to the veins. The arterioles, capillaries, and venules together are collectively called microcirculation because you can only see these structures under a Microscope. Many students incorrectly think of arteries as the vessels that carry oxygenated blood and veins as the vessels that carry deoxygenated blood. However, Dr. Nguyen warned students to not think about arteries and veins in this way. In the systemic circuit. arteries carry oxygen-rich blood to the tissues, and veins carry oxygen-poor blood to the heart. In the pulmonary circuit, however, the opposite is true: arteries carry oxygen-poor blood to the lungs, and veins carry oxygen-rich blood back to the heart. Although you'll likely see the terms oxygenated and deoxygenated used here and in the textbook, a better description of the blood is either oxygen-rich and oxygen-poor. The term "deoxygenoted" suggests that the blood returning to the heart in the systemic circuit or blood leaving the heart in the pulmonary circuit is completely devoid of oxygen. This isn't the case; it's just lower in oxygen than blood leaving the heart in the systemic circuit or blood coming back to the heart in the pulmonary circuit. Anatomy of Blood Blood is a connective tissue composed of three basic components: plasma, the buffy coat, and erythrocytes. Plasma consists of water with dissolved proteins and electrolytes. The plasma makes up about 55% of the blood's volume. The buffy coat is the layer of blood containing platelets (cell fragments with a clotting function) and leukocytes (white blood cells with immune function). It makes up less than 1% of the whole volume of blood. Erythrocytes are red blood cells containing hemoglobin. (Hemoglobin is a red-pigmented protein; this is why blood is red.) Red blood cells have a biconcave disc shape, which gives them the flexibility to fold and move single-file through the capillaries. This characteristic shape is important because, in many cases, blood cells are larger in diameter than the capillaries through which they travel. The fact that red blood cells move through capillaries in single-file manner allows them to maximize the contact of the red blood cells with the capillaries themselves, making gas exchange more efficient. The biconcave disc shape also increases the red blood cell's surface area, which allows it to carry more oxygen. (The fraction of our blood that is comprised of red blood cells is called the hematocrit, and it is often denoted as [HCT].) Dr. Nguyen mentioned that blood anatomy is covered in detail in Chapter 15, but that we won't cover that chapter in this course. The Two Circuits of the Human Circulatory System The human circulatory system consists of two circuits: The pulmonary circuit, in which oxygen-poor blood flows to the lungs and then oxygen-rich blood flows back to the heart. The systemic circuit, in which oxygen-rich blood flows to the capillary beds all over the body (except the lungs) and then oxygen-poor blood flows back to the heart. Blood from all over the body converges in the right atrium from the superior and inferior venae cavae. Because the various parts of the body use different levels of oxygen, the blood contains differing levels of oxygen, and so it is known as "mixed venous blood." The amount of blood pumped from each circuit must be the same, so the volume of blood that gets pumped from the right ventricle must be the same amount that gets pumped from the left ventricle. Blood moves through the cardiovascular system in two ways: In series blood flow, the blood flow happens one ofter the other, in series. One movement has to happen, and then the next, and then the next. Blood's path between the pulmonary and systemic circuits occurs in series. Blood flows from the heart, to the lungs, back to the heart, and to the rest of the body in linear fashion. Blood must be pumped to the lungs before it goes to the rest of the body because it must become oxygenated. The heart is often said to be a muscular double pump because it has to pump the same volume of blood twice: once to the pulmonary circuit and once to the systemic circuit.. Series blood flow also occurs with blood traveling to the GI tract and then to the liver. Blood flows to the GI tract, where it picks up nutrients. It then goes through a portal vein towards another capillary bed in the liver, which detoxifies and removes waste products. We also see series capillary flow in the kidneys. Parallel blood flow occurs when blood flows through the systemic circuit in a manner which does not have a strict order. The blood travels through the aorta and arteries, which branch off to reach each organ, before again flowing through the veins that come together at the superior or inferior venae cavae. Blood flows to many organs at the same time-it does not need to go to the liver before it goes to the colon, for example. Parallel blood flow in the systemic circuit ensures that all of the body's tissues receive an adequate amount of oxygen. If blood flowed through the systemic circuit in series, the organs at the end of the series would always receive oxygen-poor blood. Parallel blood flow also allows the body to regulate blood flow by reducing blood flow to one organ and increasing blood flow to another. For example, during exercise, the body increases blood flow to the skeletal system and decreases blood flow to the urinary and digestive systems. The heart and brain generally stay at a stable rate of blood flow. Blood is generally depicted in the figures of the textbook in either red or blue coloration, when in reality, blood is always red, no matter how much oxygen it has. Note, however, that the body does not contain any blue pigment-the reason your veins appear blue is because the red blood is showing through the veins' thick layer of connective tissue. Dr. Nguyen also stressed that it is important not to associate the red and blue coloration with artery and vein, as you may have been taught in the past, but rather with oxygen-rich and oxygen-poor blood. Path of Blood Flow You will need to remember how blood flows. For example, if you are told that a red blood cell is in the left atrium, you need to know where it will go and where it came from. The following diagram shows the general path of oxygenated and deoxygenated blood through the heart: Suppose that we start in the left atrium, which contains oxygenated blood that has just been delivered by the pulmonary vein. Beginning in the left atrium, oxygenated blood flows through the bicuspid valve (also called the mitral or left AV valve) to the left ventricle. From the left ventricle, blood flows through the aortic semilunar valve to the aorta. Blood flows from the aorta to the systemic circuit. It is at this point that oxygen is taken out of the blood. The deoxygenated blood flows from the systemic circuit back to the heart through the superior and inferior venae cavae. Blood flows from the superior and inferior venae cavae to the right atrium. Blood flows from the right atrium through the tricuspid valve (or right AV valve) to the right ventricle. From the right ventricle, blood flows through the pulmonary semilunar valve to the pulmonary artery. Blood flows from the pulmonary artery to the lungs, where it becomes oxygenated. The oxygenated blood then flows in the pulmonary vein back to the left atrium of the heart. Electrical Activity of the Heart For the heart to pump blood through the vasculature, the cardiac muscle must contract in a highly synchronized manner. If any contraction occurs out of sync, the entire system is in jeopardy. The chambers do not contract at exactly the same time- rather, they contract in a coordinated manner, with the atria first, followed by the ventricles. These contractions are managed by a highly elaborate conduction system in the myocardium. The heart has myogenic contractile ability, which literally means "muscle generated." This is because the heart itself causes the contractions without the aid of a neuron. (Compare this to the neurogenic contractile ability of skeletal muscle. Skeletal muscle cannot contract without an external signal, usually from a neuron.) Myogenic contraction of the heart is accomplished through specialized cardiac Pacemaker cells spontaneously generate action potentials and re-establish the heart rhythm (also known as pace). They are found in the SA and AV nodes (which are discussed below). Conduction fibers are large-diameter cardiac myocytes that transmit action potentials throughout the heart. EXAM TIP: The autorhythmic cells In the heart are specialized myocytes - cardiac muscle cells. They are not neurons. The sinoatrial (SA) node is localized in the posterior superior wall of the right atrium, and contains many specialized myocytes called pacemaker cells. It is located near the right atrioventricular (AV) node, which is found near the wall, between the right atrium and ventricle. The pacemaker set of cells spontaneously generate an action potential that spreads across both atria through gap junctions. The SA node is the most important pacemaker cell cluster in the heart and sets the pace that all other nodes follow. The basic path of electrical activity in the heart is as follows: A pacemaker (the SA node) in the superior aspect of the right atrium generates a wave of signals that tells the heart to contract. The SA node is simply a chunk of specialized cardiac myocytes, which are small but otherwise look like normal cardiac muscle cells. They are striated and branched, and they have intercalated discs. The SA node will depolarize, and once this occurs the electrical signals will spread to neighboring cells through gap junctions in intercalated discs. The signal is delayed for a fraction of a second at the AV node, which lies buried in a fibrous capsule at the base of the atria. The signal passes to the atrioventricular (AV) bundle, also called the bundle of His, which connects the atria and the ventricles. The signal then passes to the heart's apex via bundle branches that are long cardiac myocytes whose only job is to act as an "electrical highway" to the apex. The signals spread throughout the ventricles, from the apex back up the lateral walls of the ventricles. The change in voltage triggers a change in voltage in neighboring cells through the Purkinje fibers, causing a contraction. Note that the small delay of the signal at the AV node is important because it causes a delay between the contraction of the atria and the contraction of the ventricles. This gives the valves time to close, preventing backflow of blood into the atria. Also, it is physiologically significant that the electrical signal goes from the apex up. Recall that contraction starts from the bottom and goes up because that is the direction that blood flows. Additionally, the SA node has a much greater role in setting the pace of the heart; however, if it were to stop working, the heart could continue with the AV node, just at a much slower pace. Explaining the Heartbeat The electrical signal from the SA node at the tip of the right atrium passes to the AV node at the base of the right atrium, then jumps all the way down to the apex of the heart. The signal is then passed back up through the ventricles. This process can be depicted as follows: The electrical signal originates at the pacemaker (SA node). The signal passes to the AV node, at the base of the atria. The signal then passes to the apex of the heart. The signal spreads back up through the ventricles. Why does the signal jump to the apex of the heart and then travel back up the ventricles? You can analogize squeezing blood out of the heart to squeezing toothpaste out of a tube of toothpaste. When you squeeze toothpaste out of a tube, you don't squeeze toward the lip of the tube; you start at the bottom and push up. Likewise, when the heart squeezes blood out of the ventricles, it pushes up, because that's where the blood exits the heart. Electrocardiograms (ECGs) We evaluate the electrical activity of the heart because we want to know about the mechanical activity of the heart. The electrical activity of the heart can be recorded with an electrocardiogram (which is commonly referred to as an ECG or EKG). With this method, the electrical events of the heart can be recorded from the skin's surface through the use of electrodes arranged into an inverted equilateral triangle (referred to as Einthaven's Triangle) centered on the chest. (Recall that the body is primarily filled with water, and water is a good electrical conductor.) Each electrode is connected to a volt-meter recording device and arranged in leads, where one lead is a pair of electrodes (one positive electrode and one negative electrode). The leads are named with Roman numerals I, II, and Ill. The Lead I ECG is located between the right arm and the left arm, the Lead II ECG is located between the right arm and the left leg, and the Lead Ill ECG is located between the left arm and the left leg. The more synchronized the electrical activity, the larger the signal. Dr. Nguyen mentioned that there are other ECGs that use more electrodes. For example, a 12-lead ECG uses additional electrodes in the chest area to provide even better information about electrical activity. You won't need to worry about these other kinds of ECGs in this Class. The ECG can detect the direction and length of time of a signal, as well as how much tissue was depolarized. ECG readings are used to detect problems with the electrical activities in the heart. Also, an ECG reflects patterns of action potentials firing throughout all of the cells of the myocardium. The ECG records the difference in surface electrical potential at each electrode in a lead and compares them to one another. Electrical activity moving towards the positive electrode in the lead will be an upward blip on the ECG output, and it indicates a depolarization. If the wave is going towards the negative electrode in the lead, it will manifest as a downward blip on the ECG reading. Reading an ECG Output For the exam, you will be expected to read and understand the basic information found in an ECG output. It is important to use electrical terms rather than mechanical terms to discuss the results of on ECG-the ups and downs on the graph represent depolarization, not contraction! There is a gap in time between the depolarization and the actual contractile event. The larger the amplitude of the deflection on the ECG, the larger the number of depolarized cells. The ECG output can be divided into three basic parts: The P-wave represents the atrial depolarization that happens before contraction. The QRS complex represents ventricular depolarization. Atrial repolarization also occurs here, but it's dwarfed by the force of ventricular depolarization. The T-wave is the period of time when the ventricles repolarize. The size of the T-wave varies from person to person. Some people have distinctive T-waves, while others have barely any T- waves at all. The same is not true for the QRS complex or P-waves, which are similar in amplitude from person to person. The R-wave is so much larger than the other waves because the ventricle walls are very thick and the cells all depolarize together, thus causing a great ventricular depolarization.