Nuclear Transport PDF
Document Details
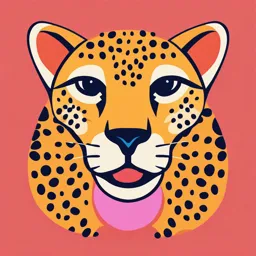
Uploaded by CalmFairy
جامعة البترا-الأردن & كلية الطب-جامعة الأزهر-مصر
Tags
Summary
This document discusses the transport of molecules between the nucleus and the cytosol. It details the structure of the nuclear envelope and nuclear pore complexes, and the mechanisms involved in transporting different types of molecules across the nuclear membrane. The document covers basic concepts of cell biology.
Full Transcript
THE TRANSPORT OF MOLECULES BETWEEN THE NUCLEUS AND THE CYTOSOL The nuclear envelope. The double membrane of the nuclear envelope is penetrated by nuclear pore complexes. Transmembrane proteins in the inner and outer nuclear membranes link the nuclear lamina to the cytosolic cytoskeleto...
THE TRANSPORT OF MOLECULES BETWEEN THE NUCLEUS AND THE CYTOSOL The nuclear envelope. The double membrane of the nuclear envelope is penetrated by nuclear pore complexes. Transmembrane proteins in the inner and outer nuclear membranes link the nuclear lamina to the cytosolic cytoskeleton. The outer nuclear membrane is continuous with the endoplasmic reticulum (ER). The ribosomes that are normally bound to the cytosolic surface of the ER membrane and outer nuclear membrane are not shown This nuclear envelope consists of two concentric membranes:. The inner nuclear membrane contains proteins that act as binding sites for the nuclear lamina, The Lamina: a meshwork of polymerized protein subunits ( intermediate filament family of cytoskeletal proteins) called nuclear lamins. The lamina provides structural support for the nuclear envelope and acts as an anchoring site for chromosomes and nuclear pore complexes. The lamina is also connected to the cytoplasmic cytoskeleton via protein complexes that span the nuclear envelope, thereby providing structural links between the DNA, nuclear envelope, and cytoskeleton. The outer nuclear membrane is continuous with the membrane of the ER and is studded with ribosomes engaged in protein synthesis. The proteins made on these ribosomes are transported into the space between the inner and outer nuclear membranes (the perinuclear space), which is continuous with the ER lumen. Nuclear pores conduct extensive bidirectional traffic between the cytosol and the nucleus. The many proteins that function in the nucleus—including histones, DNA polymerases, RNA polymerases, transcriptional regulators, and RNA-processing proteins—are selectively imported into the nuclear compartment from the cytosol, where they are made. All RNAs that function in the cytosol—including mRNAs, rRNAs, tRNAs, and miRNAs—are exported after they are synthesized and processed in the nucleus NPCs in the nuclear envelope Each NPC is composed of a set of approximately 30 different proteins, or nucleoporins. NPCs display eightfold rotational symmetry, with axial symmetry of the central core. The nuclear envelope of a typical mammalian cell contains 3000–4000 NPCs, Each NPC can transport a staggering 1000 macromolecules per second and can transport in both directions at the same time. The internal diameter of each NPC is ∼40 nm, large enough to accommodate ribosomal subunits and even viral particles. The central portion of the NPC, nucleoporins can be classified into: (1) Transmembrane ring proteins that span the nuclear envelope and anchor the NPC to the envelope (2) Scaffold nucleoporins that form layered ring structures (some scaffold nucleoporins are membrane-bending proteins that stabilize the sharp membrane curvature where the nuclear envelope is penetrated); and (3) Channel nucleoporins that line a central pore. These unstructured domains contain numerous repeats of phenylalanine– glycine (FG) motifs whose weak affinity for each other creates a gel-like mesh inside the NPC. This mesh acts as a sieve that restricts the diffusion of large macromolecules while allowing smaller molecules to pass. Researchers have determined the effective size of the sieve by injecting labeled water- soluble molecules of different sizes into the cytosol and then measuring their rate of diffusion into the nucleus The function of a nuclear localization signal. Sorting signals called nuclear localization signals (NLSs) are responsible for the selectivity of this active nuclear import process. The most commonly used signal consists of one or two short sequences that are rich in the positively charged amino acids lysine and arginine , with the precise sequence varying for different proteins. Many NLSs function even when linked as short peptides to the surface of a cytosolic protein, suggesting that the precise location of the signal within the amino acid sequence of a nuclear protein is not important. NPC transport occurs through a large, constitutively open, mesh-filled pore, rather than through a much smaller protein translocator whose aqueous pore is typically gated by the protein being transported. For this reason, fully folded proteins and large multiprotein complexes can be transported in either direction through the nuclear pore The particles first arrive at the tentacle-like fibrils that extend from the scaffold nucleoporins at the border of the NPC into the cytosol, and then proceed through the center of the NPC. This observation illustrates that NLSs impart the ability of large particles to navigate through the otherwise impermeable diffusion barrier posed by the disordered mesh inside the nuclear pore. The function of a nuclear localization signal. The normal T-antigen protein T-antigen with an altered nuclear contains the lysine-rich sequence localization signal (a threonine indicated and is imported to its site of replacing a lysine) remains in the action in the nucleus, as indicated by cytosol. immunofluorescence staining with antibodies against the T-antigen Nuclear Import Receptors Different nuclear import receptors bind different nuclear localization signals and thereby different cargo proteins. Cargo protein 4 requires an adaptor protein to bind to its nuclear import receptor. The adaptors are structurally related to nuclear import receptors and recognize nuclear localization signals on cargo proteins. They also contain a nuclear localization signal that binds them to an import receptor, but this signal only becomes exposed when they are loaded with a cargo protein Nuclear Import Receptors Nuclear localization signals must be recognized by nuclear transport receptors. Karyopherin family members that mediate nuclear import are called nuclear import receptors, while those for nuclear export are called nuclear export receptors. Each import receptor can bind and transport the subset of cargo proteins containing the appropriate nuclear localization signal. Nuclear import receptors sometimes use adaptor proteins that form a bridge between the import receptors and the nuclear localization signals on the proteins to be transported. The import receptors are soluble cytosolic proteins that contain multiple low-affinity binding sites for the FG repeats found in the unstructured domains of several nucleoporins. The FG repeats in the fibrils of cytosol-facing nucleoporins serve to initially recruit import receptors and their bound cargo proteins to NPCs. The import receptors can then bind the FG repeats that form the mesh inside the nuclear pore to disrupt interactions between the repeats. In this way, the receptor-–cargo complex locally dissolves the gel-like mesh and can diffuse into and within the NPC pore. At this rate, a cargo in a complex with an import receptor could traverse the distance across an NPC in a few milliseconds, consistent with the rate of transport observed in cells. It is important to realize that in this model, diffusion is not directional; instead, the import receptor simply accelerates diffusion to provide cargo access to the nuclear compartment. As we will see, it is the selective dissociation of cargo only on the nuclear side of the NPC that confers directionality to the import process. The import receptor then returns back to the cytosol for transport of the next cargo. Interaction of nuclear import receptors with FG repeats. Nuclear import receptors contain various low-affinity Cargo receptors can rapidly partition into the FG repeat FG repeat–binding sites on their surface. This facilitates mesh by interacting with the FG repeats and locally melting their initial recruitment to NPCs because of interactions the mesh. This partitioning into and out of the mesh with FG repeats found on the cytosolic fibrils of the substantially accelerates the diffusion of the cargo receptor NPCs. The interior of the NPC is filled with a mesh of (and its bound cargo) through the NPC. Proteins without FG repeat–containing proteins whose weak interactions surface FG repeat–binding sites cannot melt the mesh, and with each other restrict nonspecific diffusion of proteins their diffusion through the NPC is comparatively slow and other macromolecules through the pore. Nuclear import and nuclear export both use the Ran GTPase cycle. Movement through the NPC of loaded nuclear transport receptors occurs along the FG repeats displayed by certain NPC proteins. The differential localization of Ran-GTP in the nucleus and Ran-GDP in the cytosol provides directionality to both nuclear import (A) and nuclear export (B). Ran GAP stimulates the hydrolysis of GTP to produce Ran-GDP on the cytosolic side of the NPC. The critical difference between Ran-mediated nuclear import and nuclear export is the nature of cargo binding by the cargo receptor. Ran is GTPases, a molecular switch that can exist in two conformational In nuclear import, cargo binding is mutually states: exclusive of Ran-GTP; in nuclear export, cargo a cytosolic GTPase-activating protein (GAP) triggers GTP hydrolysis binding requires Ran-GTP. and thus converts Ran-GTP to Ran-GDP, Thus, the locations where cargo is picked up and a nuclear guanine nucleotide exchange factor (GEF) promotes the released are exactly reversed in nuclear export exchange of GDP for GTP and thus converts Ran-GDP to Ran-GTP. compared to nuclear import. Ran GAP is located in the cytosol and Ran GEF is located in the nucleus, the cytosol contains mainly Ran-GDP, and the nucleus contains mainly Ran-GTP Transcription Through NPCs Can Be regulated by Controlling Access to the Transport Machinery Feedback regulation of cholesterol biosynthesis. SREBP (sterol response element binding protein), a latent transcription regulator that controls expression of cholesterol biosynthetic enzymes, is initially synthesized as an ER membrane protein. It is anchored in the ER if there is sufficient cholesterol in the membrane by interaction with a membrane protein complex composed of the proteins INSIG and SCAP (SREBP cleavage activation protein), which binds cholesterol. Transcription Through NPCs Can Be regulated by Controlling Access to the Transport Machinery Feedback regulation of cholesterol biosynthesis. If the cholesterol-binding site on SCAP is empty (at low cholesterol concentrations), SCAP changes conformation and dissociates from INSIG. Dissociation from INSIG frees the SCAP–SREBP complex so it can be packaged together into transport vesicles that are delivered to the Golgi apparatus. In the Golgi apparatus, two Golgi-resident proteases cleave SREBP to free its cytosolic domain from the membrane. The cytosolic domain, which is a transcription regulatory protein, then moves into the nucleus, where it binds to the promoters of genes that encode proteins involved in cholesterol biosynthesis and activates their transcription. In this way, more cholesterol is made when its concentration falls below a threshold. The breakdown and re-formation of the nuclear envelope and lamina during mitosis. Phosphorylation of the lamins triggers the disassembly of the nuclear lamina, which initiates the breakup of the nuclear envelope. Dephosphorylation of the lamins reverses the process. An analogous phosphorylation and dephosphorylation cycle occurs for some nucleoporins and proteins of the inner nuclear membrane, and some of these dephosphorylation events are also involved in the reassembly process. The lamin network beings to re-form around regions of individual decondensing daughter chromosomes. The lamins recruit membranes that contain interacting lamin receptors that were in the inner nuclear membrane. Eventually, as decondensation progresses, these membrane structures fuse to form a single complete nucleus. Mitotic breakdown of the nuclear envelope occurs in all metazoan cells. However, in many other species, such as yeasts, the nuclear envelope remains intact during mitosis, and the nucleus divides by fission.