Chapter 11 Section 7.docx
Document Details
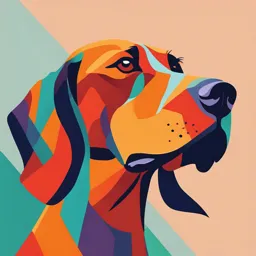
Uploaded by [email protected]
Tags
Full Transcript
Hello, future aviators, and welcome back to our journey through the skies. Previously, we explored how atmospheric conditions can significantly impact aircraft performance. Today, we\'ll ascend to new heights as we delve into the dynamics of Thrust and Power in Climbs. As we climb through today\'s l...
Hello, future aviators, and welcome back to our journey through the skies. Previously, we explored how atmospheric conditions can significantly impact aircraft performance. Today, we\'ll ascend to new heights as we delve into the dynamics of Thrust and Power in Climbs. As we climb through today\'s learning, you\'ll gain a solid understanding of these critical concepts, which are paramount for your progression as a commercial pilot. Let\'s begin by defining \'thrust\'. Thrust is the forward force produced by the aircraft\'s engines that propels it through the air. In the context of climbing, thrust is the muscle enabling our aircraft to overcome the forces of weight and drag, pushing us skyward. I want you to visualize thrust as the hand of Zeus, if you will, lifting our metal Icarus up from the earth. Now, \'power\' in aviation speaks to the rate at which work is done or energy is transformed. Think of it as the stamina that sustains thrust over time, allowing our aircraft to not just reach, but also maintain altitude during a climb. Power is the quiet strength that keeps our wings steady and our path upward constant. During a climb, the intricate dance between thrust and the aircraft\'s weight is a spectacle. It\'s integral to realize that as our weight increases, the amount of thrust required to maintain a climb also rises. For our visual learners, imagine thrust as the constant force battling against the relentlessness of gravity trying to pull our weight back to earth. Airspeed plays a strategic role in managing thrust and power. It\'s a Goldilocks situation where too little or too much airspeed can adversely affect engine performance and aircraft handling. Pilots must find that \'just right\' speed to optimize the balance between lift and engine output. Now, assessing climb performance often involves scrutinizing the thrust-to-weight ratio. This ratio is a critical metric --- think of it as your aircraft\'s personal powerlifting record, dictating how vigorously we can ascend into the firmament. A high thrust-to-weight ratio means we can climb steeper and faster, which can be crucial in certain flight scenarios. But what happens when we reach higher altitudes? Our engine performance might wane as the air becomes thinner, affecting our power output. This challenge requires pilots to have profound knowledge and proficiency to manage engine settings and airspeed, ensuring that our climb isn\'t prematurely curtailed. The types of climbs, whether we\'re discussing maximum angle or rate climbs, each demand their own specific power settings. Pilots must adapt, troubleshooting their flight path based on performance needs. Some of you may ask, "How do we find the finesse to optimize thrust and power during a climb?" It\'s a mix of scientific understanding and artful piloting. We adjust pitch, throttle, and sometimes sacrifice speed for altitude, or vice versa, to maintain the desired trajectory. Let\'s reflect on some real-world implications. Proper management of thrust and power during climbs not only ensures fuel efficiency and timely arrivals but also plays a part in noise abatement and satisfying airspace restrictions. It\'s a responsibility pilots carry to harmonize our flying machines with the world below. In conclusion, thrust and power in climbs are not just about getting from point A to point B vertically. It\'s about the grace with which we do it, the efficiency of our journey, and the safety of our passengers and crew. Mastering this is akin to an aerial ballet, where every move is precise, every decision calculated. Prepare for our next venture where we\'ll dissect Calculating Climb Performance in the following lecture. It\'s time to translate today\'s theoretical understanding into practical, numerical wisdom, ensuring your calculations are as sharp as your piloting skills. Thank you for joining me on today's ascent. Keep your charts handy and your mind ready for the climb ahead. See you in the skies. Welcome back, aviators! In our previous lecture, we discussed the essential concepts of pressure and density altitude. Today, we embark on a critical aspect of flight performance: Calculating Climb Performance. Understanding and mastering this is crucial for every aspiring commercial pilot. So, let\'s dive in and elevate our knowledge on this topic. First, let\'s briefly understand why climb performance matters in aircraft operations. Climb performance pertains to the aircraft\'s ability to gain altitude. In commercial flying, optimizing this ability can make the difference between a safe, efficient flight and one that challenges both fuel economy and safety margins. Now, what is the Maximum Angle of Climb (AOC)? AOC is the steepest climb angle an aircraft can achieve and is significantly essential when we need to clear obstacles after takeoff. It\'s all about gaining the most height in the least horizontal distance possible. Likewise, the Maximum Rate of Climb (ROC) is another critical measurement, focusing on gaining altitude quickly over time rather than distance. It represents the most efficient climb vis-à-vis fuel consumption and time. So, how do we calculate these? The formulas for AOC and ROC involve various factors such as weight, thrust, drag, and air density. Let\'s take a step-by-step approach and begin with AOC. To calculate the Maximum Angle of Climb, we need to understand the ratio of excess power to weight. Excess power is the available power beyond what is required for level flight. Don\'t worry if this sounds intricate; we\'ll go through an example shortly. For ROC, we focus on excess thrust. Calculating ROC involves determining the excess thrust available, which is the thrust remaining after overcoming the drag in level flight, and then relating it to weight. But what variables affect these calculations? We need to account for air density, aircraft weight, and configuration, flap settings, engine performance, and even wind. Each of these can significantly alter the outcome of your climb performance calculations. Let\'s put theory into practice. When calculating AOC, we start by determining the excess power at a given aircraft weight and configuration. Next, we\'ll consider the density altitude to understand the available engine performance. With these numbers crunched, we can derive our AOC. Turning to ROC, we begin by calculating the thrust-to-weight ratio at our desired level flight speed. We then adjust for any thrust changes due to altitude and determine the excess thrust. This leads us to the ROC. Consider, for instance, a scenario where we\'re taking off from a high-altitude airport with a heavy load. Our calculations will show that both AOC and ROC would be less than at sea level due to thinner air and higher weight. But how do we use these calculations in flight planning? Pilots use AOC when obstacle clearance is a priority, such as in mountainous terrains. ROC is frequently used when establishing a safe climb to cruising altitude is essential for route efficiency and fuel conservation. To close, let\'s recap our climb performance calculations. We\'ve focused on two pivotal measurements---AOC and ROC, which are critical for flight performance and safety. Understanding how to calculate these using the various variables prepares you to make informed decisions during the planning and execution of your flights. In our next lecture, which is part of your climb performance analysis, we\'ll delve into Climb Performance Factors, where you\'ll learn how to optimize your climb given different operational conditions. This learning journey is bound to intensify your abilities in planning and executing critical flight strategies. Thank you for engaging in today\'s climb performance calculations, and I look forward to guiding you through the continued ascent in our next video. Climb performance in aircraft is a critical aspect that every commercial pilot must understand and manage effectively. The ability to climb efficiently to cruising altitude affects not only the flight time but also the fuel consumption and overall safety of the flight. Several factors play a role in determining an aircraft\'s climb performance, and understanding these factors allows pilots to optimize their flight profile. \*\*Thrust and Power\*\* Thrust and power are two fundamental concepts that are crucial in understanding aircraft climb performance. Thrust is the forward force produced by the aircraft\'s engines that allows it to overcome air resistance and gravity. During a climb, thrust must exceed the drag and weight components that act along the flight path to achieve a positive rate of climb. Power, on the other hand, is the rate at which work is done or energy is transferred. In aviation, it is the product of thrust and the aircraft\'s velocity. For a given aircraft weight, more power is required to climb at a steeper angle or at a higher rate. \*\*Maximum Angle of Climb (AOC)\*\* The Maximum Angle of Climb is the steepness of the climb path and is mainly used when clearing obstacles after takeoff. It is achieved when the excess thrust over drag is maximal, allowing the aircraft to gain altitude quickly over a short horizontal distance. The AOC is critical during departures from airports with significant obstacles in the takeoff flight path, and it ensures that the aircraft maintains a safe clearance above these obstacles. Calculating the AOC involves understanding the thrust-to-weight ratio, drag characteristics, and current atmospheric conditions. Pilots must refer to performance charts that account for the current weight and balance, ambient temperature, and altitude to determine the precise climb angle. \*\*Maximum Rate of Climb (ROC)\*\* The Maximum Rate of Climb is concerned with how quickly an aircraft can gain altitude, measured in feet per minute (fpm). This rate is significant during the climb to cruising altitude as it affects the overall efficiency of the flight. A higher ROC means a shorter time to reach cruising altitude, leading to a more efficient flight path and fuel savings. To calculate the ROC, pilots must consider several performance factors, including aircraft weight, engine power available, atmospheric conditions, and the aircraft\'s aerodynamic efficiency. Like the AOC, the ROC is best determined by consulting the performance charts specific to the aircraft and the day\'s flying conditions. \*\*Optimizing Climb Performance\*\* Pilots can optimize climb performance by carefully managing weight, ensuring proper maintenance of engines for maximum power output, and adjusting the climb speed to match the best rate or angle of climb as suggested by the Aircraft Flight Manual (AFM) or Pilot's Operating Handbook (POH). Understanding weight\'s impact on climb performance is crucial. An aircraft that is overweight will have a reduced climb gradient and rate, making it more challenging to clear obstacles and reach cruising altitude efficiently. Pilots must ensure that the aircraft is loaded within its allowable weight limits and that the weight is appropriately distributed to maintain the correct center of gravity (CG). In-flight, adjusting the airspeed to the recommended climb speed can make significant differences in climb efficiency. Flying too fast or too slow can both result in a less-than-optimal climb rate or angle. Pilots must also be aware of the changing atmospheric conditions such as temperature, pressure, and wind, which can affect engine performance and lift. In conclusion, understanding the factors that influence climb performance and knowing how to optimize them is essential for safe and efficient flight operations. Pilots who master the concepts of power, thrust, AOC, and ROC will ensure that their aircraft is operated within the safest and most efficient parameters during the climb phase of flight.