Advanced Drug Delivery Systems - Pharmaceutical Polymers PDF
Document Details
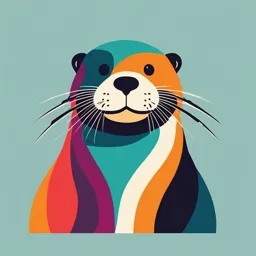
Uploaded by PropitiousBalance429
Al-Zaytoonah University of Jordan
Rania Hamed
Tags
Summary
This document provides an overview of advanced drug delivery systems and pharmaceutical polymers. It details the concepts of monomers, polymers, and different polymerization methods. The document also covers applications of polymers in various industries.
Full Transcript
Advanced Drug Delivery Systems Pharmaceutical Polymers Rania Hamed Professor Faculty of Pharmacy Al-Zaytoonah University of Jordan Introduction What is the difference between polymer and macromolecules? Polymer is made of repeating units. Macromolecule refers to any large molecu...
Advanced Drug Delivery Systems Pharmaceutical Polymers Rania Hamed Professor Faculty of Pharmacy Al-Zaytoonah University of Jordan Introduction What is the difference between polymer and macromolecules? Polymer is made of repeating units. Macromolecule refers to any large molecule, not necessarily just those made of repeating units. Polymers are a subset of macromolecules. Macromolecule (carbohydrate, Cellulose-polymer proteins, lipids, and nucleic acids) Introduction A monomer is a small molecule that combines with other molecules of the same or different types to form a polymer. If two, three, four, or five monomers are attached to each other, the product is known as a dimer, trimer, tetramer, or pentamer, respectively. An oligomer contains from 30 to 100 monomeric units. Products containing > 200 monomers called a polymer. Polymers cannot exist in the gaseous state because of their high molecular weight. They exist only as liquids or high solid materials. Introduction Synthetic and natural-based polymers are widely used in pharmaceutical and biomedical industries. The application of pharmaceutical polymers are growing at a fast pace. It is important to understanding the function of polymers as ingredients in drug products to develop new formulations for better delivery systems. History of polymers Polymers are more commonly referred to as “plastics”. Plastics have the ability to be molded, cast, extruded, drawn, thermoformed, or laminated into a final product such as plastic parts, films, and filaments. The first semisynthetic polymer was cellulose nitrate (1845), polyethylene (1933), poly (vinyl chloride) (1933), polystyrene (1933), polyamide (1935), Teflon (1938), and synthetic rubbers (1942). The plastics revolution advanced technologies in the 20th century and opened new fields of application in the pharmaceutical and biomedical sectors. Polymers in industries Polyethylene for insulation Rubber tires Teflon strip Nylon parachutes Polymers in drug delivery Polymers have been used in: Oral delivery for coatings, binders, taste maskers, protective agents, drug carriers, and release controlling agents. – Targeted delivery to the lower part of the gastrointestinal tract (e.g. colon) by using polymers that protect drugs during their passage through the harsh environment of the stomach. – Transdermal patches for backings, adhesives, or drug carriers in matrix. – Biodegradable polymers may be used for controlled delivery of proteins and peptides. – The key difference between early polymers and pharmaceutical polymers is biocompatibility. Polymer synthesis Monomers have to interact with each other to form a polymer. The structure of the monomer molecule will tell us how polymerization occurs. Addition polymerization: The unsaturated monomer that contain a double bond of σ (sigma) and π (pi) between a pair of electrons. The π bond generally requires low energy to break; polymerization starts at this site by the addition of a free radical on the monomer. Condensation polymerization: If a monomer does not contain a double bond but possesses reactive functional groups such as hydroxyl, carboxyl, or amines, they can interact via condensation. Polymer synthesis 1. Addition polymerization – Also known as free-radical or chain polymerization – The initiator is an unstable molecule that is cleaved into two radical-carrying species under the action of heat, light, chemical, or high-energy irradiation – Radical has the ability to attack the double bond of a monomer – Typically used for monomers with double bonds (ethylene- based) or sterically strained cyclic monomers – Fast, high MW chains are formed at the beginning of the reaction (e.g. Styrene and acrylic acid derivatives) Addition polymerization Polymerization involves three steps: Initiation: Radical is transferred to the monomer and a monomer radical is produced. Propagation: The monomer radical is able to attack another monomer and then another monomer, and so on and so forth. A macroradical is formed. Termination: Macroradicals can undergo another reaction with another macroradical or with another inert compound which terminates the macroradical. Addition polymerization Addition polymerization of styrene Initiation Propagation Termination Polymer synthesis 2. Condensation polymerization – Also called step polymerization – Two or more monomers with different reactive functional groups (hydroxyl, carboxyl, or amines) interact with each other, resulting in the liberation of a small molecule such as water, gas, or salt – Slower, high MW polymer is formed near the end of the polymerization when most of the monomer has been depleted – e.g. Nylon Condensation polymerization Example: A monomer containing a reactive hydrogen from the amine residue can react with another monomer containing a reactive hydroxyl group (a residue of carboxyl group) to generate a new functional group (amide) and water as a side product. Synthesis of nylon-6,6 Nylon is prepared via condensation polymerization of a diamine and diacid chloride. Addition vs. Condensation Condensation polymerization is a step-wise reaction in Addition reaction is which smaller species are characterized by fast growth initially formed first and then of macroradicals. High– combined to make higher- molecular-weight chains are molecular-weight species. formed at the beginning of This reaction tends to be the reaction. slow generally lasting for several hours. Polymer synthesis techniques 1. Bulk polymerization Homogeneous 2. Solution polymerization 3. Suspension and inverse suspension polymerization Heterogeneous 4. Emulsion and inverse emulsion polymerization (Dispersed systems) Bulk polymerization Advantages The simplest technique Highest polymer purity High yield and easy polymer recovery Requires only a monomer, a monomer-soluble initiator, and sometimes a chain-transfer agent to control the MW. If the monomer is water-soluble, a linear water-soluble polymer is prepared. If the monomer is oil-soluble, a linear oil-soluble polymer is prepared. Limitations: removing residual monomer, heat dissipation especially in free-radical polymerization. Solution polymerization To solve the problems associated with exothermic heat in bulk polymerization, polymerization can be conducted in solution. The solvent or diluent molecules reside in between the monomer molecules, reduce the amount of interaction between the two neighboring monomers. Less amounts of heat are generated in a given period of time The solvent should be able to dissolve the monomer and initiator. Solvent should have melting and boiling points suitable for the reaction conditions and solvent removal. Disadvantages include the relatively low yield and the need for a solvent removal step. Dispersion polymerization Two incompatible phases of water and oil are dispersed into each other (dispersed phase and continuous phase). The active material (monomer) can be water-soluble or oil- soluble. The monomer (in the dispersed phase) is dispersed into the continuous phase using a surface-active agent. The surfactant should be soluble in the continuous phase. Two factors control the nature of the dispersion system: Surfactant concentration Surface tension of the system Emulsion systems that use water as a continuous phase are known as latex Dispersion polymerization Copolymers and polymer blends Polymer systems can be physically blended or chemically reacted if one polymer system cannot address the needs of a particular application. Copolymerization: a polymerization reaction in which more than one type of monomer is involved. Homopolymer: when one monomer is involved in the polymerization process (e.g. polyethylene) The monomer sequence can range from random to perfectly alternating; the actual sequence is determined by the relative reactivities of the monomers e.g. Eudragit polymers……………Exercise # 1: More about this polymer Copolymers If the two monomers have similar reactivity, no preference for which monomer is added next, polymer product is called a random copolymer. When one monomer is preferentially added to another monomer, the monomers are added to each other alternatively, polymer product is called an alternate copolymer. Monomers preferentially add onto themselves and a block copolymer is formed, this happens when one monomer has a very high reactivity toward itself. A monomer and a polymer are generally used to make graft copolymers. Pluronic surfactants (EO-PO-EO terpolymers) are composed of block units of ethylene oxide and propylene oxides attached to each other. Copolymer examples Polymer blends Polymer properties can be simply changed by mixing or blending one or two different polymers in molten or in solution state. E.g. Some thermoplastic polymers have rigid structures will break apart upon stresses Adding a flexible polymer such as rubber improves their resistance and prevents the cracks from growing. Interpenetrating polymer networks (IPNs) Semi-IPNs are prepared by dissolving a polymer into a solution of another monomer An initiator and a cross-linker is added into the solution and the monomer is polymerized and cross-linked in the presence of the dissolved polymer The result will be a structure in which one cross-linked polymer interpenetrates into a non–cross-linked polymer system (Semi-IPNs). In complete IPNs, two different monomers and their corresponding cross-linkers are polymerized and cross- linked simultaneously This results in a doubly cross-linked polymer system that interpenetrates into one another. Interpenetrating polymer networks (IPNs) a) Two different polymers b) Semi-interpenetrating polymer network c) Interpenetrating polymer network Polymer topology The topology of a polymer describes whether the polymer structure is linear, branched, or cross-linked Topology can affect polymer properties in its solid or solution states In a linear polymer, non-covalent (weak) intermolecular forces hold the polymer chains together. A linear polymer can show dual behavior: – Linear chains can freely move, which offer the polymer a low melting temperature – Linear chains have a higher chance of approaching each other in their solid state, which increases their crystallinity and melting temperature Polymer topology In branched polymers, chains move with difficulty because of the steric hindrance induced by the side groups but they also possess weak intermolecular forces, which can help them move freely In cross-linked polymers, the chains are chemically linked and will be restricted from moving depending on the level of cross-linking. Highly cross-linked polymers form very rigid structures. Exercise # 2: Crosslinking vs. entanglement, give examples Polymer isomerism 1. Structural isomerism – Occurs when there are unsaturated sites along a polymer chain – Results in cis and trans isomers – Different glass transition temperature (Tg) and melting temperature (Tm). Polymer isomerism 2. Sequence isomerism – Monomers with pendant groups can attach to each other in head-to-tail, head-to-head, or tail-to-tail conformation. Polymer isomerism 3.Stereoisomerism Applies to polymers with chiral centers Three different configurations: Isotactic: pendant groups located on one side Syndiotactic: pendant groups located alternatively on both sides Atactic: pendant groups located randomly on both sides Thermoplastics Are polymers with a linear or branched structure. Can be reversibly refabricated and reshaped with heat (undergo melting) Are originally solids that can flow upon application of heat. The process of thermomelting and solidification can be repeated indefinitely – Ex. Polystyrene, polyethylene, polypropylene, poly(vinyl chloride) Thermosets Are formed from cross-linked polymers (combination of a cross-linker and heat or combination of heat and reaction of internal functional groups). Resist thermal softening and cannot be reshaped. Their flow behavior is temperature independent. No reversible melting and solidifying (very useful when a thermoresistant polymer is desirable). A cross-linked polymer loses its processability as chain movement is hindered with the addition of cross-linker. Addition of cross-links to the polymer will reduce their solubility in that solvent and swell when they are placed in a compatible solvent. – Ex. Epoxy, Fiberglas® Crystalline and amorphous polymers Polymers display different thermal, physical, and mechanical properties depending on their structure, molecular weight, linearity, intra- and intermolecular interactions. In linear polymers, polymer chains can pack together in regular crystalline lattice or crystalline state and exhibit a sharp melting temperature. Crystalline and amorphous polymers When the polymer structure is irregular, polymers form glassy (amorphous) domains instead of crystal domains. Amorphous structure is formed due to rapid cooling of a polymer melt in which crystallization is prevented by quenching Amorphous polymers do not generally display a sharp melting point; instead, they soften over a wide temperature range. Crystalline and amorphous polymers Polystyrene and poly (vinyl acetate) are amorphous with melting range of 35ºC to 85ºC and 70ºC to 115ºC, respectively. Poly (butylene terephthalate) and poly (ethylene terephthalate) are very crystalline with sharp melting range of 220 and 250ºC to 260ºC, respectively. Exercise # 3: Get the differential scanning calorimetry (DSC) thermograms for the polymers above indicating the melting range. Crystalline and amorphous polymers Polymer strength and stiffness increases with crystallinity as a result of increased intermolecular interactions From a pharmaceutical perspective: – Crystallinity increases the barrier properties of the polymer important for packaging and coating – Small molecules like drugs or solvents usually cannot penetrate or diffuse through crystalline domains – Diffusion and solubility are two important terms that are related to the level of crystallinity in a polymer. – A less crystalline or amorphous polymer is preferred when the release of a drug is desired Thermal transitions When a crystal melts, the polymer volume increases significantly as the solid turns to a liquid. The melting temperature (Tm) represents a first-order thermal transition in polymers. The volume of an amorphous polymer gradually changes over a wide temperature range or so-called glass transition temperature (Tg). This behavior represents a second-order thermal transition in polymers. Tm and Tg of a given polymer can be detected by differential scanning calorimetry (DSC) as an endothermic peak and a baseline shift, respectively. Thermal transitions in polymers Glass transition temperature (Tg) Tg is not an absolute property of a material and is influenced by the factors affecting the movement of polymer chains. At temperatures > Tg, polymers are rubbery and flow more easily. Tg values for linear organic polymers range from about −100°C to above 300°C. Glass transition temperature (Tg) Tg is an important factor for solid dosage forms A chewable dosage form needs to be soft and flexible at mouth temperature (37ºC) Thus polymer used as a chewable matrix should be softened at 37ºC Pharmaceutical polymers with their Tg values close to 37°C would be the best candidates. e.g. Nicotine gum (Nicorette®) gum is used as an aid in smoking cessation Exercise # 4: Give more information about Nicorette® Glass transition temperature (Tg) Segmental motion in polymers is facilitated by the empty space in between the polymer chain ends (free volume) As the free volume increases, polymer segments gain more freedom to move For example, low- and high density polyethylenes are different in the free volume inside their structures. At a given weight, a low density polymer occupies more volume as compared with its high-density counterpart, polymer chain can move more easily resulting in a lower Tg value Exercise # 5: Get the Tg for low- and high-density polyethylenes Glass transition temperature (Tg) Factors affecting Tg: 1. Chain length Polymers containing short chains or having lower molecular weight possess greater free volume more freedom to move lower Tg 2. Side groups (branching) Branching increases free volume lower Tg However, bulky side groups increase steric hindrance higher temperature is needed to induce polymer chain motion higher Tg (e.g. Tg of polystyrene vs. polypropylene) Exercise # 6 Polar side groups also increase Tg due to increased intermolecular interactions (e.g. Tg of Poly (vinyl chloride) vs. polyethylene) Exercise # 7 Glass transition temperature (Tg) 3. Chain flexibility More chain flexibility lower Tg (display higher entropy, desire to move) Flexible and rigid chains behave similar to liquid and solid, respectively. 4. Cross-linking Adding cross-links to linear polymer chains limits chain movement resulting in less entropy at a given temperature higher Tg For very highly cross-linked polymers, Tg values are expected to be very high to the extent that the polymer starts to decompose before it shows any segmental motion. 5. Plasticizers Plasticizer molecules can increase the entropy and mobility of the polymer chains lower Tg Plasticized polymers A plasticizer is added to a polymer to enhance its flexibility help in processing facilitate relative movement of polymer chains against each other Thus reduces Tg of the mixture. Plasticizers increase molecular motion, thus drug molecules can diffuse through the plasticized polymer matrix at a higher rate depending on the plasticizer concentration. Exercise # 8: Give two examples of plasticizers and their use in drug delivery Molecular weight of polymers A typical polymer batch contains chains with different lengths (MW) and MW distribution. A very narrow molecular weight distribution is desired for a polymer that is intended to be mechanically strong. Since chains are different, a polymer cannot be identified as a molecule with a specific MW polymer MW is expressed as the average MW of all chains Polymer MW can be expressed in different ways: – Number-average MW (Mn): related to number of chains – Weight-average MW (Mw): related to chain size – Viscosity-average MW Mn and Mw are most common Molecular weight of polymers If all polymer chains are similar in size, then Mn = Mw. If chains are of different sizes, then Mn ≠ Mw. Polydispersity (PD) indicates how far the weight average can distance itself from the number average. PD is given by the ratio Mw/Mn (≥ 1) A PD value ≈ 1 means the polymer system is close to monodispersed and all polymer chains are almost similar in size. A PD value > 1 indicates that the polymer system is polydispersed and chains are different in size. Molecular weight of polymers If you have two batches of the same polymer with the following composition: Where Ni is the no. of polymer chains having a mass of Mi Molecular weight of polymers Both batches have the same Mn of 25,000 g/mol. Both batches have different Mw of 30,000 g/mol for batch 1 and 70,000 g/mol for batch 2. Polydispersity of 2.8 versus 1.2 indicates that the batch 2 contains very different chains. Should you, as a pharmaceutical scientist, claim that the two batches are similar and you can use them interchangeably within your formulation? If both polymer batches are soluble in water, they will show different solubility behavior. The shorter chains will dissolve faster in water than longer chains. Drug release from these batches will be different due to different PD values. Explain? Mechanical properties Depending on the polymer’s structure, MW, and intermolecular forces, polymers respond differently to an applied stress. Examples of important polymer mechanical properties: – Stretching (tensile strength) – Compression (compressive strength) – Bending (flexural strength) – Sudden stress (impact strength) – Dynamic loading (fatigue) A flexible polymer can perform better under stretching whereas a rigid polymer is better under compression. Mechanical properties A polymer is subjected to a load (stress) and its deformation (strain) is monitored to measure its strength. For elastic materials (metals and ceramics), the stress and strain correlation is linear up to breaking point. Elastic materials show high stress and very low elongation (strain) at their breaking point. E.g. fibers and highly cross-linked polymers display elastic behavior. The increase in the intermolecular forces within a cross-linked polymers, resulting in steep slope of the stress/strain line. The steeper the slope, the higher the modulus. – Modulus = stiffness and both refer to polymer strength Mechanical properties Some polymeric materials do not display a breaking point. Instead, they yield at certain stresses and continue to deform under lower stresses before they finally break apart (e.g. tough plastics). Energy is needed to break the polymer apart. The area under the stress/strain curve measures the energy requires to break the polymer apart (toughness of polymer). The larger the area is, the tougher the polymer. Mechanical properties Viscoelastic properties Polymers are classified as viscoelastic materials Viscoelastic materials are neither a pure solid nor a pure fluid Viscoelastic materials have the ability to store energy (display elastic behavior) and to dissipate it (display viscous behavior) E.g. Poly (vinyl chloride) has Tg of about 100°C. This means, it behaves like a solid at temperatures Tg Characterized by elasticity Characterized by viscosity Classical extremes Viscoelastic properties Viscoelasticity in polymers can be evaluated by: 1. Creep test: the polymer is first loaded with a certain weight (stress) and its deformation (strain) is then monitored over time. Explain the below figure. Viscoelastic properties 2. Stress relaxation test: the polymer is first deformed to a certain extent, and then its stress relaxation is monitored with time. Molecular weight and polymer properties Mechanical properties of a polymer generally increase with an increase in molecular weight As molecular weight increases, polymer chains are more likely entangled into each other at certain molecular weights, resulting in poor polymer flow Varieties of polymers-Rubbers Silicone is a very inert rubber with almost no affinity to any material Silicone rubber is an excellent candidate for implants in biomedical applications Rubbers are not very strong in their raw form but they have a potential to be cross-linked and cured at high pressure and temperature. Tg of the rubbery polymers (elastomers) are below the room temperature. Exercise # 8: Implants in biomedical applications, short discussion Plastics Tg of plastics is generally above the room temperature, opposed to elastomers. Plastics are manufactured by injection molding, extrusion, and thermoforming that require the plastic to be in its molten state. Plastics Polymers such as polyethylene, polypropylene, and polystyrene have only carbon in their backbone. Plastics used in engineering applications are required to be resistant to impact, weather, and solvent. These are heterogeneous plastics, which have N, Si, and O in their backbone. Polyesters, polyamides, and polyacetals are engineering plastics with very high intermolecular forces and hence high melting point. Exercise # 9: Chemical structures of Polyesters, polyamides, and polyacetals Fibers Polymers for fibrous products are: required to have a crystalline structure with a very sharp melting point need to be meltable and spinnable Examples of fiber-forming materials are cellulose acetate, rayon, polypropylene, nylon, polyester, polyamide, and polyacrylonitrile. Adhesives Polymers for adhesive applications should have adhesiveness properties Adhesive forces (interaction with a second material) should be in balance with cohesive forces (interaction with itself) Adhesive and cohesive forces increase with the molecular weight of the polymer as molecular interactions increase between the same or different molecules due to increased surface area The adhesive polymer intended for a nonpolar adherent should be nonpolar and for very polar adherent should be very polar (“like dissolves like”) Exercise # 10: what dosage forms need adhesive polymers Coatings Coatings are used for protection purposes Coating polymer is exposed to a second environment such as air, oxygen, water, stomach fluid, intestinal fluid, and solvents (e.g. poly (vinyl acetate), acrylate esters, ethyl cellulose. Exercise # 11: what dosage forms need coating polymers Polymers as rheology modifiers Polymer chains are in a coiled conformation at rest and in extended conformation once they are loaded. When increased viscosity of the solution is desirable, the goal is to increase the chain end-to-end distance under a given load. In dissolution and swelling of a polymer, the load originates from the interaction of a polymer and a solvent. Polymers as rheology modifiers Longer end-to-end distances are obtained if the polymer chains are longer and have more interaction with the solvent. In more hydrophilic polymer, water is better as a solvent and in more lipophilic polymer, dissolution or swelling is better in organic medium. Rheological behavior (viscosity of polymers) is characterized by the volume occupied by the polymer chains. Gums are hydrophilic and high molecular weight polymers are the best candidates for increasing the viscosity of the aqueous solutions or dispersions. Polymers as rheology modifiers Hydrogels Hydrogels are made of hydrophilic polymers that are able to swell rapidly when placed in excess water and retain large volumes of water in their structures Hydrogels are cross-linked either by chemical bonds or by cohesion forces such as ionic interaction, hydrogen bonding, or hydrophobic interactions Hydrogels behave like an elastic solid which can return to their original conformation even after a long-term loading If a polymer structure is nonionic, the major driving force of swelling within the hydrogel is polymer–solvent interactions If a polymer structure is ionic, the driving forces of swelling within the hydrogel are osmotic and electrostatic forces Swelling forces in hydrogels Exercise # 12: discuss the driving force of Carbopol® used for hydrogel. Chemical gels Are covalently cross-linked gels Will not dissolve in water or other organic solvent unless the covalent cross-links are broken apart. Two different approaches can be used to form chemical gels Cross-linking through double bond is energetically favored as less energy is required to break a double bond than to react the functional groups Physical gels Hydrogen bonding, hydrophobic interaction, and complexation are three major tools in preparing a physical gel A hydrogen bond occurs in poly(vinyl alcohol, PVA) of MW > 100,000 g/mol, which is water insoluble. To dissolve PVA, the hydrogen bonds need to be broken by heating the solution up to 80-90°C. Hydrophobic interactions are the driving force for the folding of thermoresponsive hydrogels (methylcellulose, hydroxypropyl methylcellulose, or PEO/PPO/PEO triblock copolymers). These polymers dissolve in cold water and form viscous solutions. Once the solution temperature increases up to a certain point, these solutions become thicker by forming a gel. Physical gels PVA Pluronic Physical gels Complexation may happen between two oppositely charged groups of different polymer structures or via metal ions E.g. alginic acid with negatively charged groups and chitosan with positively charged groups can form a complex gel in water The solubility of the complex is dependent on the pH of the dissolution medium and the pKa of the polymers Alginic acid with negatively charged carboxyl groups can form insoluble complexes with divalent and trivalent ions such as calcium, aluminum, and iron. These complexes are reversible and pH-dependent.