The Microbial World PDF
Document Details
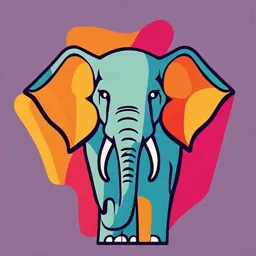
Uploaded by mirehh
Tags
Summary
This textbook excerpt introduces the microbial world, covering topics such as microscopy, the origins of microbiology, microbial cultivation, and molecular biology. It discusses the structure and activities of microbial cells, including prokaryotic and eukaryotic cells, and explores the diversity and functions of microorganisms in various environments. The text also touches on the impact of microorganisms on human society, including their roles in disease, agriculture, industry, and environmental processes.
Full Transcript
The Microbial World 1 I Exploring the Microbial World 38...
The Microbial World 1 I Exploring the Microbial World 38 II Microscopy and the Origins of Microbiology 54 III Microbial Cultivation Expands the Horizon of Microbiology 61 IV Molecular Biology and the Unity and Diversity of Life 67 MICROBIOLOGYNOW Microbiology in Motion The microbial world is strange and fierce. It is teeming with Listeria are soil organisms that sometimes find their way life, ancient, diverse, and constantly changing. Microorgan- into our food. In soils they infect other microbes such as isms are Earth’s life support system, and from our first amoebae. Our cells are similar in many ways to those of breath they influence nearly every moment of our lives. microscopic organisms, and so Listeria finds itself well Microbes are in our water and our food, and we carry them adapted to live within us. This bacterium has the unique on us and in us. Indeed, microbes abound in any natural ability to hijack cellular systems, causing actin to polymerize environment that will support life, including many environ- and propel the cell like a rocket within the host cytoplasm. ments too hostile for higher life forms. The force of this propulsion causes Listeria to penetrate While the microbial world is invisible, we can explore it adjacent cells (image, lower left), spreading the infection. through the science of microbiology. Microbiology evolves at Listeria can also invade host vacuoles (not shown), where it a breathtaking pace. Even the microscope continues to hides and survives. This persistent state can prolong evolve, providing an ever more detailed picture of the infection and promote resistance to antibiotic therapy. microbial world. The image above was made with a fluores- Research on Listeria has provided new insights on the cence microscope that uses lasers, guided by a computer, to biology of this pathogen and an ever-changing view of a map the three-dimensional structure of cells. The image microbial world in motion. shows neighboring human cells with their nuclei stained blue and actin filaments stained green. These cells are Source: Kortebi, M., et al. 2017. Listeria monocytogenes switches infected with the foodborne bacterial pathogen Listeria from dissemination to persistence by adopting a vacuolar lifestyle in monocytogenes, stained red. epithelial cells. PLoS Pathog. 13: e1006734. 37 M01_MADI4790_16_GE_C01.indd 37 08/03/2021 20:46 38 UNIT 1 THE FOUNDATIONS OF MICROBIOLOGY This chapter launches our journey into the microbial world. Here 1 UNIT we will begin to discover what the science of microbiology is all about and what microorganisms are, what they do, and how they can be studied. We also place microbiology in historical context, as a process of scientific discovery driven by simple (yet powerful) experiments and insightful minds. I Exploring the Microbial World T he microbial world consists of microscopic organisms that have defined structures, unique evolutionary histories, and are of enormous importance to the biosphere. D.E. Caldwell Jiri Snaidr 1.1 Microorganisms, Tiny Titans of the Earth M icroorganisms (also called microbes) are life forms too small to be seen by the unaided human eye. These microscopic organisms are diverse in form and function, and they inhabit every (a) (b) environment on Earth that supports life. Many microbes are undif- ferentiated single-celled organisms, but some can form complex structures, and some are even multicellular. Microorganisms typi- cally live in complex microbial communities (Figure 1.1), and their activities are regulated by interactions with each other, with their environments, and with other organisms. The science of microbiol- ogy is all about microorganisms, who they are, how they work, and what they do. Microorganisms were teeming on the land and in the seas for bil- lions of years before the appearance of plants and animals, and their diversity is staggering. Microorganisms represent a major fraction of Earth’s biomass, and their activities are essential to sustaining life. Indeed, the very oxygen (O2 ) we breathe is the result of microbial activities. Plants and animals are immersed in a world of microbes, and their evolution and survival are heavily influenced by microbial (c) activities, by microbial symbioses, and by pathogens—those microbes that cause disease. Microorganisms are woven into the fabric of Figure 1.1 Microbial communities. (a) A bacterial community that developed in the human life as well (Figure 1.2), from infectious diseases, to the food depths of a small Michigan lake, including cells of various phototrophic bacteria. The we eat, the water we drink, the fertility of our soils, the health of our bacteria were visualized using phase-contrast microscopy. (b) A bacterial community in a sewage sludge sample. The sample was stained with a series of dyes, each of animals, and even the fuel we put in automobiles. Microbiology is which stained a specific bacterial group. From Journal of Bacteriology 178 : the study of the dominant form of life on Earth, and the effect that 3496–3500, Fig. 2b. © 1996 American Society for Microbiology. (c) Colorized scanning microbes have on our planet and all of the living things that call electron micrograph of a microbial community scraped from a human tongue. it home. Microbiologists have many tools for studying microorganisms. Microbiology was born of the microscope, and microscopy is foun- of disease and microbial biochemical diversity has relied on the dational to microbiology. Microbiologists have developed an array ability to grow microorganisms in the laboratory. of methods for visualizing microorganisms, and these microscopic The ability to grow microorganisms rapidly under controlled con- techniques are essential to microbiology. The cultivation of micro- ditions makes them highly useful for experiments that probe the organisms is also foundational to microbiology. A microbial culture fundamental processes of life. Most discoveries relating to the is a collection of cells that have been grown in or on a nutrient molecular and biochemical basis of life have been made using medium. A medium (plural, media) is a liquid or solid nutrient microorganisms. The study of molecules and their interactions is mixture that contains all of the nutrients required for a microorgan- essential to defining the workings of microbial cells, and the tools ism to grow. In microbiology, we use the word growth to refer to of molecular biology and biochemistry are foundational to micro- the increase in cell number as a result of cell division. A single micro- biology. Molecular biology has also provided a variety of tools to bial cell placed on a solid nutrient medium can grow and divide study microorganisms without need for their cultivation in the labo- into millions or even billions of cells that form a visible colony ratory. These molecular tools have greatly expanded our knowledge (Figure 1.3). The formation of visible colonies makes it easier to see of microbial ecology and diversity. Finally, the tools of genomics and grow microorganisms. Comprehension of the microbial basis and molecular genetics are also cornerstones of modern M01_MADI4790_16_GE_C01.indd 38 08/03/2021 20:46 CHAPTER 1 The Microbial World 39 1 UNIT Animal Health Human Health Ecosystem Health Water & Waste Agriculture Natural Resources Food Biotechnology Industry Bioenergy Figure 1.2 Microbial applications. Microorganisms have major impacts on the world in which we live. In the chapters that follow we will learn how microorganisms impact our health, the foods we eat, the water we drink, and even the air we breathe. We will learn how microbes can be used to produce valuable products and the many ways in which microorganisms touch our lives. microbiology and allow microbiologists to study the genetic basis the cytoplasm, from the outside. The cytoplasm is an aqueous mix- of life, how genes evolve, and how they regulate the activities of cells. ture of macromolecules (for example proteins, lipids, nucleic In the next section, we explore the basic elements of microbial cell acids, and polysaccharides), small organic molecules (mostly the structure and summarize the major physiological activities that take precursors of macromolecules), various inorganic ions, and ribo- place in all cells, regardless of their structure. somes. All cells also contain ribosomes, which are the structures responsible for protein synthesis. Some cells have a cell wall that Check Your Understanding lends structural strength to a cell. The cell wall is a relatively perme- In what ways are microorganisms important to humans? able structure located outside the cytoplasmic membrane and is a Why are microbial cells useful for understanding the basis much stronger layer than the membrane itself. Cell walls are typi- of life? cally found in plant cells and most microorganisms but are not found in animal cells. What is a microbial colony and how is one formed? There are two fundamental cell types that differ categorically in cellular organization: those having prokaryotic cell structure, and those having eukaryotic cell structure (Figure 1.4). Cells having 1.2 Structure and Activities eukaryotic cell structure are found in a group of organisms called of Microbial Cells the Eukarya. This group includes plants and animals as well as Microbial cells are living compartments that interact with their envi- diverse microbial eukaryotes such as algae, protozoa, and fungi. Mastering Microbiology ronment and with other cells in dynamic ways. We purposely Eukaryotic cells contain an assortment of membrane-enclosed cyto- Art Activity: exclude viruses in most of this discussion because although they plasmic structures called organelles (Figure 1.4b). These include, Figure 1.3 Common resemble cells in many ways, viruses are not cells but instead a spe- most prominently, the DNA-containing nucleus but also mitochon- elements of prokaryotic/ cial category of microorganism. We consider the structure, diversity, dria and chloroplasts, organelles that specialize in supplying the cell eukaryotic cells and activities of viruses in Section 1.4 and in Chapters 5 and 11. with energy, and various other organelles. Prokaryotic cell structure is found within two different groups of Elements of Microbial Structure organisms we know as Bacteria and Archaea. Prokaryotic cells have few internal structures, they lack a nucleus, and they typically lack All cells have much in common and contain many of the same organelles (Figure 1.4a). Bacteria and Archaea appeared long before components (Figure 1.4). All cells have a permeability barrier called the evolution of eukaryotes (Section 1.5). While all Archaea and the cytoplasmic membrane that separates the inside of the cell, M01_MADI4790_16_GE_C01.indd 39 08/03/2021 20:46 40 UNIT 1 THE FOUNDATIONS OF MICROBIOLOGY 0.01 mm (10 om) 90 mm 1 UNIT 2 mm (b) Paul V. Dunlap Paul V. Dunlap (a) (c) Figure 1.3 Microbial cells. (a) Bioluminescent (light-emitting) colonies of the bacterium Photobacterium grown in laboratory culture on a Petri plate. (b) A single colony can contain more than 10 million (107) individual cells. (c) Colorized scanning electron micrograph of cells of Photobacterium. Bacteria have prokaryotic cell structure, these two groups diverged Activities of Microbial Cells very early in the history of life and as a result many of their molecu- To be competitive in nature, a microorganism must survive and lar and genetic characteristics differ at a fundamental level. Indeed, reproduce. Figure 1.5 considers structure and some of the activities we will see later that in many ways Archaea and Eukarya are more that are performed by cells to drive survival and reproduction. All similar to each other than either is to Bacteria. cells show some form of metabolism through which nutrients are acquired from the environment and transformed into new cellular Genes, Genomes, Nucleus, and Nucleoid materials and waste products. During these transformations, energy In addition to a cytoplasmic membrane and ribosomes, all cells also is used to support synthesis of new structures. Production of these possess a DNA genome. The genome is the full set of genes in a cell. new structures culminates in the division of the cell to form two A gene is a segment of DNA that encodes a protein or an RNA cells. Microbial growth results from successive rounds of cell molecule. The genome is the living blueprint of an organism; the division. characteristics, activities, and very survival of a cell are governed by Genes contain information that is used by the cell to perform the its genome. work of metabolism. Genes are decoded to form proteins that regu- The genomes of prokaryotic cells and eukaryotic cells are orga- late cellular processes. Enzymes, those proteins that have catalytic nized into structures called chromosomes. In eukaryotic cells, DNA activity, carry out reactions that supply energy and perform biosyn- is present as several linear molecules (each one formed into its own thesis within the cell. Enzymes and other proteins are synthesized chromosome) within the membrane-enclosed nucleus. By contrast, during gene expression in the sequential processes of transcription the genomes of Bacteria and Archaea are typically closed circular and translation. Transcription is the process by which the informa- chromosomes (though some prokaryotic cells have linear chromo- tion encoded in DNA sequences is copied into an RNA molecule, somes). The chromosome aggregates within the prokaryotic cell to and translation is the process whereby the information in an RNA form the nucleoid, a mass that is visible in the electron microscope molecule is used by a ribosome to synthesize a protein (Chapter 6). (Figure 1.4a) but which is not enclosed by a membrane. Most pro- Gene expression and enzyme activity in a microbial cell are coordi- karyotic cells have only a single chromosome, but many also contain nated and highly regulated to ensure that the cell remains optimally one or more small circles of DNA distinct from that of the chromo- tuned to its surroundings. Ultimately, microbial growth requires some, called plasmids (Figure 1.4a). Plasmids typically contain replication of the genome through the process of DNA replication, genes that are not essential but often confer some special property followed by cell division. All cells carry out the processes of tran- on the cell (such as a unique metabolism, or antibiotic resistance). scription, translation, and DNA replication. The genomes of Bacteria and Archaea are typically small and com- Microorganisms have the ability to sense and respond to pact, and most contain between 500 and 10,000 genes encoded by changes in their local environment. Many microbial cells are capa- Mastering Microbiology 0.5 to 10 million base pairs of DNA. Eukaryotic cells typically have ble of motility, typically by self-propulsion (Figure 1.5). Motility Art Activity: Figure 1.4 much larger and much less streamlined genomes than prokaryotic allows cells to relocate in response to environmental conditions. Basic properties cells. A human cell, for example, contains approximately 3 billion Some microbial cells undergo differentiation, which may result of microbial cells base pairs, which encode about 20,000–25,000 genes. M01_MADI4790_16_GE_C01.indd 40 08/03/2021 20:46 CHAPTER 1 The Microbial World 41 Cell wall 1 UNIT Cytoplasmic membrane John Bozzola and Nucleoid M.T. Madigan Cytoplasm Bacteria Plasmid Ribosomes H. König and K.O. Stetter (a) Prokaryotic cell Archaea Cell wall Cytoplasmic membrane Mitochondrion Nuclear membrane Nucleus Ribosomes Endoplasmic reticulum Cytoplasm S.F. Conti and T.D. Brock Golgi complex Eukarya (b) Eukaryotic cell Figure 1.4 Microbial cell structure. (a) (Left) Diagram of a prokaryotic cell. (Right) Electron micrograph of Heliobacterium modesticaldum (Bacteria, cell is about 1 mm in diameter) and Thermoproteus neutrophilus (Archaea, cell is about 0.5 mm in diameter). (b) (Left) Diagram of a eukaryotic cell. (Right) Electron micrograph of a cell of Saccharomyces cerevisiae (Eukarya, cell is about 8 mm in diameter). In terms of relative scale, the bacterial cell in a is about the same size as the mitochondria of Saccharomyces in b. in the formation of modified cells specialized for growth, disper- Figure 1.5 occur in all cells. Metabolism, growth, and evolution, sal, or survival. Cells respond to chemical signals in their environ- however, are universal and will be major areas of emphasis ment, including those produced by other cells of either the same throughout this text. or different species, and these signals often trigger new cellular We now move on to consider the diversity of cell shapes and sizes activities. Microbial cells thus exhibit intercellular communica- found in the microbial world. tion; that is, they are “aware” of their neighbors and can respond accordingly. Many prokaryotic cells can also exchange genes with Check Your Understanding neighboring cells, regardless of their species, in the process of What structures are universal to all type of cells? horizontal gene transfer. What processes are universal to all types of cells? Evolution (Figure 1.5) results when genes in a population of What structures can be used to distinguish between cells change in sequence and frequency over time, leading to prokaryotic cells and eukaryotic cells? descent with modification. The evolution of microorganisms can be very rapid relative to the evolution of plants and animals. For example, the indiscriminate use of antibiotics in human and vet- erinary medicine has selected for the proliferation of antibiotic 1.3 Cell Size and Morphology resistance in pathogenic bacteria. The rapid pace of microbial evo- Microscopic examination of microorganisms immediately reveals lution can be attributed in part to the ability of microorganisms their morphology, which is defined by cell size and shape. A variety to grow very quickly and to acquire new genes though the process of cell shapes pervade the microbial world, and although micro- of horizontal gene transfer. Not all of the processes depicted in scopic by their very nature, microbial cells come in a variety of sizes. M01_MADI4790_16_GE_C01.indd 41 08/03/2021 20:46 42 UNIT 1 THE FOUNDATIONS OF MICROBIOLOGY Properties of all cells: 1 UNIT Structure Metabolism Growth Evolution All cells have a cytoplasmic All cells use information encoded Information from DNA is Chance mutations in DNA cause membrane, cytoplasm, a genome in DNA to make RNA and protein. converted into proteins, which new cells to have new properties, made of DNA, and ribosomes. All cells take up nutrients, do work. Proteins are used to thereby promoting evolution. transform them, conserve energy, convert nutrients from the Phylogenetic trees built from and expel wastes. environment into new cells. DNA sequences capture Cytoplasmic evolutionary relationships DNA membrane 1. Catabolism (transforming between species. molecules to produce energy and building blocks) 2. Anabolism (synthesizing Distinct macromolecules) species Ribosomes Cell & Cytoplasm Distinct Ancestral species Environment cell Properties of some cells: Differentiation Communication Motility Horizontal gene transfer Some cells can form new cell Cells interact with each other Some cells are capable of Cells can exchange genes by structures such as a spore. by chemical messengers. self-propulsion. several mechanisms. Flagellum DNA Spore Donor cell Recipient cell Figure 1.5 The properties of microbial cells. While cells are tremendously diverse in form and function, certain properties are shared by all cells. Cell shape can be useful for distinguishing different microbial cells with the square of its size. This relationship means that, as cell size and often has ecological significance. Moreover, the very small size increases, it becomes advantageous to have cellular structures that of most microbial cells has a profound effect on their ecology and facilitate transport and compartmentalize cellular activities as dictates many aspects of their biology. We begin by considering cell seen in eukaryotic cells. In contrast, since diffusion is rapid at size and then consider cell shape. small spatial scales, high metabolic rates can be maintained in small prokaryotic cells without a need for complex cellular The Small World structures. A micrometer (mm or micron) is one-millionth of a meter in It is possible, though unusual, for prokaryotic cells to be visible length. The unaided human eye has difficulty resolving objects that to the human eye; the largest are more than 600 mm (0.6 mm) long. are less than 100 mm in diameter, but this is the scale of the micro- To achieve this size, these bacteria must have traits that allow them bial world. Most prokaryotic cells are small, ranging between 0.5 to overcome diffusional limitation. The bacterium Epulopiscium and 10 mm in length, but prokaryotic cells can vary widely in size. fishelsoni (Figure 1.6a; Figure 1.9), which is found in the gut of the For example, the smallest prokaryotic cells are about 0.2 mm in surgeonfish, can be more than 75 mm wide and 600 mm long diameter and the largest can be more than 600 mm long (Table 1.1). (Table 1.1). One of the traits that allows this bacterium to get so In contrast, most eukaryotic cells are larger on average than pro- large is that it can have more than 10,000 copies of its genome dis- karyotic cells, being between 5 and 100 mm in length, but eukary- tributed throughout its cytoplasm, thereby preventing diffusional otic cells can vary widely in size too. For example, the smallest limitation between the genome and any region of the cytoplasm. eukaryotic microorganism known is about 0.8 mm in diameter Cells of the largest known bacterium, the sulfur-oxidizing chemoli- and the largest eukaryotic cells can be many centimeters in length thotroph Thiomargarita (Figure 1.6b, Table 1.1), are even larger than (Section 1.4). those of Epulopiscium, about 750 mm in diameter. Thiomargarita Cell size is influenced fundamentally by cell structure. Eukary- achieves this enormous size by having a large vacuole that fills the otic cells, owing to their complex intracellular structure and center of the cell. Hence, the cytoplasm of Thiomargarita occurs as a organelles (Figure 1.4), can actively transport molecules and mac- thin layer squeezed between the cytoplasmic membrane and this romolecules within the cytoplasm. Prokaryotic cells, in contrast, central vacuole. In this way, the cytoplasm is never more than 1 mm rely on diffusion for transport through the cytoplasm and this from the membrane. In addition, Thiomargarita, like Epulopiscium, limits their size. While diffusion is very fast at small distances, the also has many copies of its genome, which are distributed through- rate of diffusion increases as the square of the distance traveled. out its cytoplasm. Hence, the metabolic rate in a prokaryotic cell varies inversely M01_MADI4790_16_GE_C01.indd 42 08/03/2021 20:46 CHAPTER 1 The Microbial World 4 3 1 UNIT TABLE 1.1 Cell size and volume of some cells of Bacteria, from the largest to the smallest Volumes compared Organism Characteristics Morphology Size a (mm3) Cell volume (mm3) to E. coli Thiomargarita namibiensis Sulfur chemolithotroph Cocci in chains 750 200,000,000 100,000,000* Epulopiscium fishelsoni a Chemoorganotroph Rods with tapered ends 80 * 600 3,000,000 1,500,000* a Beggiatoa species Sulfur chemolithotroph Filaments 50 * 160 1,000,000 500,000* Achromatium oxaliferum Sulfur chemolithotroph Cocci 35 * 95 80,000 40,000* Lyngbya majuscula Cyanobacterium Filaments 8 * 80 40,000 20,000* Thiovulum majus Sulfur chemolithotroph Cocci 18 3,000 1,500* Staphylothermus marinus a Hyperthermophile Cocci in irregular clusters 15 1,800 900* Magnetobacterium bavaricum Magnetotactic bacterium Rods 2 * 10 30 15* Escherichia coli Chemoorganotroph Rods 1 * 2 2 1* a Pelagibacter ubique Marine chemoorganotroph Rods 0.2 * 0.5 0.014 0.007* Ultra-small bacteria a Uncultured, from groundwater Variable 60.2 0.009 0.0045* b Mycoplasma pneumoniae Pathogenic bacterium Pleomorphic 0.2 0.005 0.0025* a Where only one number is given, this is the diameter of spherical cells. The values given are for the largest cell size observed in each species. For example, for T. namibiensis, an average cell is only about 200 mm in diameter. But on occasion, giant cells of 750 mm are observed. Likewise, an average cell of S. marinus is about 1 mm in diameter. The species of Beggiatoa here is unclear, and E. fishelsoni, M. bavaricum, and P. ubique are not formally recognized names in taxonomy. For more on ultra-small bacteria, see Explore the Microbial World “Tiny Cells.” b Mycoplasma is a bacterium that lacks a cell wall and can thus take on many shapes (pleomorphic means “many shapes”). Source: Data obtained from Schulz, H.N., and B.B. Jørgensen. 2001. Annu. Rev. Microbiol. 55: 105–137, and Luef, B., et al. 2015. Nat. Commun. doi:10.1038/ncomms7372. At the opposite end of the spectrum from these large prokaryotic Surface-to-Volume Ratios, Growth Rates, and Evolution cells are very small prokaryotic cells. Exactly how small a cell can be For a cell, there are advantages to being small. Small cells have more is not precisely known. However, cells 0.2 mm in diameter exist (see surface area relative to cell volume and thus have a higher surface- Explore the Microbial World, “Tiny Cells”), and the lower limit is to-volume ratio than larger cells. To understand this principle, con- probably only a bit smaller than this. Ultimately, the lower limit to sider a spherical cell. The volume of a sphere is a function of the cell size is likely a function of the amount of space needed to house cube of its radius (V = 43pr3 ), whereas its surface area is a function the essential biochemical components—proteins, nucleic acids, of the square of the radius (S = 4πr2 ). Therefore, the S/V ratio of a ribosomes and so on (Section 1.2)—that all cells need to survive coccus is 3/r (Figure 1.7). As cell size increases, its S/V ratio decreases. and reproduce. Epulopiscium cell Esther R. Angert, Harvard University Paramecium cell Heide Schulz-Vogt (a) (b) Figure 1.6 Two very large Bacteria. (a) Epulopiscium fishelsoni. The rod-shaped cell is about 600 mm (0.6 mm) long and 75 mm wide and is shown with four cells of the protist Paramecium (a microbial eukaryote), each of which is about 150 mm long. (b) Thiomargarita namibiensis, a large sulfur chemolithotroph and currently the largest known of all prokaryotic cells. Cell widths vary from 400 to 750 mm. M01_MADI4790_16_GE_C01.indd 43 08/03/2021 20:46 44 UNIT 1 THE FOUNDATIONS OF MICROBIOLOGY r = 1 ,m of nutrients will support the synthesis of more small cells than large 1 UNIT r = 1 om Surface area (4rr2 ) = 12.6 om 2 cells. We will see that cell morphology is also often predicated on the effect of cell shape on S/V ratio. For example, cell shapes that Volume ( 3 rr3 ) = 4.2 om 3 4 increase the overall membrane area of the cell, such as those having long thin appendages or invaginations, allow bacteria to increase Surface their S/V ratio for a given mass of cytoplasm. We will see that pro- = 3/r = 3 Volume karyotic cell morphology is remarkably diverse and different cell shapes can convey different benefits upon the cell. r = 2 ,m r = 2 om Major Morphologies of Prokaryotic Cells Surface area = 50.3 om 2 Common morphologies of prokaryotic cells are shown in Figure 1.8. Volume = 33.5 om 3 A cell that is spherical or ovoid in morphology is called a coccus (plural, cocci). A cylindrically shaped cell is called a rod or a bacillus Surface (plural, bacilli). A spiral-shaped cell is called a spirillum (plural, spi- = 3/r = 1.5 Volume rilla). A cell that is slightly curved and comma-shaped is called a vibrio. A spirochete is a special kind of organism ( ▶ Section 15.17) that has a spiral shape but which differs from spirilla because the cells of spirochetes are flexible, whereas cells of spirilla are rigid. Figure 1.7 Surface area and volume relationships in cells. As a cell increases in size, its S/V ratio decreases. Some bacteria are irregular in shape. Appendages, such as stalks and hyphae, are used by some cells for attachment or to increase surface area. In addition, asymmetrical cell division such as budding can To illustrate this, consider the S/V ratio for some of the cells of dif- result in irregular and asymmetrical cell shapes. ferent sizes listed in Table 1.1: Pelagibacter ubique, 22; Escherichia coli, Cell division has a major impact on morphology because cells 4.5; and E. fishelsoni (Figure 1.6a), 0.05. The S/V of a rod-shaped that remain attached to each other can form distinctive shapes. For organism can be estimated as if it were a cylinder; hence, the S/V of instance, some cocci occur in pairs (diplococci), some form long the cell will decrease as its radius increases. chains (streptococci), others occur in three-dimensional cubes (tet- The S/V ratio of a cell controls many of its properties, including rads or sarcinae), and still others occur in grapelike clusters (staphy- how fast it grows (its growth rate) and shape. Cellular growth rate lococci). Filamentous bacteria are long, thin, rod-shaped bacteria depends in part on the rate at which cells exchange nutrients and that divide terminally and then form long filaments composed of waste products with their environment. As cell size decreases, the many cells attached end to end. S/V ratio of the cell increases, and this means that small cells can The cell morphologies described here are representative but cer- exchange nutrients and wastes more rapidly (per unit cell volume) tainly not exhaustive; many variations of these morphologies are than can large cells. As a result, free-living cells that are smaller tend known. For example, there can be fat rods, thin rods, short rods, and to be more efficient than those that are larger, and any given mass long rods, rods that occur as single cells, as pairs of cells, or rods that Norbert Pfennig Norbert Pfennig Norbert Pfennig Coccus Rod Spirillum (cocci) (rods) (spirilla) E. Canale-Parola Norbert Pfennig Stalk Hypha T.D. Brock Spirochete Budding and Filamentous appendaged Figure 1.8 Cell morphologies. Beside each drawing is a phase-contrast photomicrograph of cells showing that morphology. Coccus (cell diameter in photomicrograph, 1.5 mm); rod (1 mm); spirillum (1 mm); spirochete (0.25 mm); budding (1.2 mm); filamentous (0.8 mm). All photomicrographs are of species of Bacteria. Not all of these morphologies are known among the Archaea, but cocci, rods, and spirilla are common. M01_MADI4790_16_GE_C01.indd 44 08/03/2021 20:46 CHAPTER 1 The Microbial World 45 Explore the Microbial World UNIT Tiny Cells Viruses are very small microbes and range in Electron cryotomography, a microscopic diameter from as small as 20 nm to almost technique in which a specimen is examined 750 nm. Although no cells exist that are as at extremely cold temperatures without fixa- small as most viruses, the recent discovery of tion (chemical treatment that can alter a cell’s ultra-small bacterial cells 1,2 has pushed the morphology, see Section 1.10), showed the lower limits of cell size to what microbiolo- groundwater ultramicrobacteria to consist pri- gists feel must be very close to the minimal marily of oval-shaped cells about 0.2 mm in value. And, because microbiologists today can diameter (Figure 2). The volume of these cells deduce amazing amounts of information was calculated to be about 1/200 that of a about cells in nature without culturing them, cell of the bacterium Escherichia coli (see the lack of laboratory cultures of these tiny Table 1.1) such that more than 200 of the cells has been only a minor impediment to small cells could fit into one E. coli cell! Each understanding their biology in detail. of the tiny cells contained about 50 ribo- Microbiologists collected groundwater, somes, which is also about 1/100 of the num- which travels through Earth’s deep subsurface, ber present in a slowly growing (100-min from a Colorado (USA) aquifer (Figure 1) and generation time) cell of E. coli. The very small passed it through a membrane filter whose size of the groundwater ultramicrobacteria gives them an enormous surface- to-volume ratio, and it is hypothe- sized that this advantage benefits Figure 2 A tiny bacterial cell from anoxic ground‑ them in extracting resources water that passed through a filter with 0.2@mm pores. The cell is not quite 0.2 mm in diameter. from their nutrient-deficient habitat. Despite the fact that the tiny microbes lets them "get away" with a minimal groundwater bacteria have yet to genomic complement because any essential be cultured in the laboratory, molecules they are unable to biosynthesize much is already known about are supplied by the host. them because their small Although we do not yet know exactly how genomes—less than 1 megabase small a microbial cell can be, microbiologists (Mb) in size—were obtained and are closing in on this number from environ- analyzed. 2 From a phylogenetic mental analyses such as the Colorado ground- perspective, the different species water study. From the same samples that detected were distantly related to yielded ultra-small Bacteria in this study, major phyla of Bacteria known ultra-small Archaea were also detected and from environmental analyses of found to contain small and highly reduced diverse environments but which genomes. 2 have thus far defied laboratory cul- It is thus likely that a large diversity of ture. Further analyses showed very small prokaryotic cells occurs in nature, that genes encoding the enzymes and from the continued study of these tiny for several core metabolic path- cells, more precise values for both the lower ways widely distributed among limits to cell size and the minimal genomic Figure 1 Sampling the anoxic groundwater aquifer microorganisms were absent from requirements for life should emerge. More- that parallels the Colorado River near Rifle, Colorado. the genomes of the groundwater ultramicro- over, theoretical considerations of cell size bacteria. This suggests a metabolically mini- have shown that DNA and proteins dominate pores were only 0.2 mm in diameter. The liquid malist lifestyle for these tiny cells and a the volume of very small cells and that the that passed through the filter was then sub- survival strategy of cross-feeding essential theoretical lower limit to cell size agrees jected to microbiological analyses. Surprisingly, nutrients with neighboring species in their closely with the smallest bacteria observed in since filters with 0.2-mm pores have been microbial community. nature thus far. 3 used for decades to remove bacterial cells A strategy of obtaining nutrients from 1 Luef, B., et al. 2015. Nat. Commun. doi:10.1038/ncomms7372. from solutions to generate “sterile solutions,” other organisms is one widely used in the 2 Castelle, C.J., et al. 2015. Curr. Biol. 25: 1–12. 3 Kempes, C.P., et al. 2016. ISME J. 10: 2145–2157. prokaryotic cells were present in the ground- microbial world. As we will see later in this water filtrate. In fact, a diverse array of Bacte- book, many disease-causing (pathogenic or ria were present in the filtrate, revealing that parasitic) bacteria have very small genomes the groundwater was inhabited by a microbial that are missing many key genes otherwise community of tiny cells 1 that microbiologists necessary for a free-living lifestyle. However, have come to call ultramicrobacteria. the pathogenic or parasitic way of life of these 45 M01_MADI4790_16_GE_C01.indd 45 08/03/2021 20:46 46 UNIT 1 THE FOUNDATIONS OF MICROBIOLOGY form into filaments. As we will see, there are even square bacteria, Bacteria 1 UNIT hexagon-shaped bacteria, and star-shaped bacteria! Cell morpholo- Bacteria have a prokaryotic cell structure (Figure 1.4a). Bacteria are gies thus form a continuum, with some shapes, such as rods and often thought of as undifferentiated single cells with a length that cocci, being very common, whereas others, such as spiral, budding, ranges from 0.5 to 10 mm. While bacteria that fit this description are and filamentous shapes, are less common. common, the Bacteria are actually tremendously diverse in appear- ance, size, and function (Figure 1.9). Although most bacteria are unicel- Check Your Understanding lular, some bacteria can differentiate to form multiple cell types and What properties of the cell change as it gets smaller? others are even multicellular (for example, Magnetoglobus, Figure 1.9). Why is it that eukaryotic cells are typically larger than Among the Bacteria, 30 major phylogenetic lineages (called prokaryotic cells? phyla) have at least one species that has been grown in culture, What traits have allowed the bacteria Epulopiscium and though many more phyla exist which remain largely uncharacter- Thiomargarita to have such large cells? ized. Some of these phyla contain thousands of described species while others contain only a few. More than 90% of cultivated bac- teria belong to one of only four phyla: Actinobacteria, Firmicutes, 1.4 An Introduction to Microbial Life Proteobacteria, and Bacteroidetes. The analyses of environmental DNA sequences provide evidence for the existence of at least 80 As we have seen, microorganisms vary dramatically in size, shape, bacterial phyla (Section 1.15). and structure. In this section we will learn more about different evo- lutionary (phylogenetic) lineages of cells. All cells fall into one of three major groups: Bacteria, Archaea, or Eukarya. These three major Archaea cell lineages are called domains, and all known cellular organisms Like Bacteria, Archaea also have a prokaryotic cell structure belong to one of these three domains. In addition, while much of (Figure 1.4a). The domain Archaea consists of five described phyla: our focus in this chapter is on cellular forms of life, not all microbes Euryarchaeota, Crenarchaeota, Thaumarchaeota, Nanoarchaeota, and form cells. In this section, we will also consider viruses, which are a Korarchaeota. Archaea have historically been associated with extreme group of microorganisms that lack a cellular structure. All known environments; the first isolates came from hot, salty, or acidic sites. microorganisms can be classified into one of these four groups. But not all Archaea are extremophiles. Archaea are indeed common in 100 nm 1 om 10 om 100 om 1 x 10–7m 1 x 10–6m 1 x 10–5m 1 x 10–4m Nucleus Nucleus Circovirus Pandoravirus Mycoplasma Nanoflagellates Yeast Paramecium Tobacco mosaic Influenza A virus virus Diatoms Flagella T4 bacteriophage Ignicoccus Giganthauma Nanoarchaeum Haloquadratum Methanosarcina Magnetoglobus Epulopiscium Cell envelope Escherichia coli Spirochaetes Cytoplasm Proteins Pelagibacter Flexibacter Cyanobacteria Ribosome Nucleoid >0.2 nm Electron microscopy >0.2 om Light microscopy >100 om Visible Figure 1.9 Microorganisms vary greatly in size and 50 mm * 12 mm), yeast (Saccharomyces, 5 mm), (0.4 mm * 0.15 mm), and Mycoplasma (0.2 mm); shape. The smallest known microbe is the circovirus and nanoflagellates (Cafeteria, 2 mm); Bacteria: Archaea: Giganthauma (10-mm-diameter multicel‑ (20 nm) and the largest shown here is the bacterium Epulopiscium (700 mm * 80 mm), cyanobacteria lular filament), Ignicoccus (6 mm), Nanoarchaeum Epulopiscium (700 mm), which represents a 35,000-fold (Oscillatoria, 10-mm-diameter multicellular filaments), (0.4 mm), Haloquadratum (2 mm), Methanosarcina difference in length! Certain protozoa can be even Magnetoglobus (multicellular aggregate, 20 mm (2 mm per cell in packet); and viruses: Pandoravirus larger than Epulopiscium ( 72 mm long) and are visible diameter), Spirochaetes (2–10 mm * 0.25 mm), (1 mm * 0.4 mm), T4 bacteriophage (200 nm * 90 nm), to the unaided eye. Included in the figure are Eukarya: Flexibacter (5–100 mm * 0.5 mm filaments), Influenza A virus (100 nm), Tobacco mosaic virus Paramecium (300 mm * 85 mm), diatoms (Navicula, Escherichia coli (2 mm * 0.5 mm), Pelagibacter (300 nm * 20 nm), Circovirus (20 nm). M01_MADI4790_16_GE_C01.indd 46 08/03/2021 20:46 CHAPTER 1 The Microbial World 47 the most extreme environments that support life, such as those associ- Although they are not cells, viruses are as diverse as the cells they 1 UNIT ated with volcanic systems, and species of Archaea hold many of the infect, and different viruses are known to infect cells from all three records that define the chemical and physical limits of life as we know domains of life. Viruses are often classified on the basis of their struc- them. However, in addition to these, Archaea are found widely in ture, genome composition, and host specificity. Viruses that infect nature in nonextreme environments. For example, methane-produc- bacteria are called bacteriophages (or phages, for short). Bacteriophages ing Archaea (methanogens) are common in wetlands and in the guts have been used as model systems to explore many aspects of viral biol- of animals (including humans) and have a major impact on the ogy. While most viruses are considerably smaller than bacterial cells greenhouse gas composition of our atmosphere. In addition, species (Figure 1.9), there are also unusually large viruses such as the Pandora- of Thaumarchaeota inhabit soils and oceans worldwide and are impor- viruses, which can be more than 1 micrometer long and have a tant contributors to the global nitrogen cycle. genome that contains as many as 2500 genes, larger than that of many Archaea are also notable in that this domain lacks any known dis- bacteria! We will learn much more about viruses in Chapters 5 and 11. ease-causing (pathogenic or parasitic) species of plants or animals. Most described species of Archaea fall within the phyla Crenarchaeota Check Your Understanding and Euryarchaeota while only a handful of species have been described How are viruses different from Bacteria, Archaea, and Eukarya? for the Nanoarchaeota, Korarchaeota, and Thaumarchaeota. Analysis of What four bacterial phyla contain the largest number of well- environmental DNA sequences indicate more than 12 archaeal phyla characterized species? likely exist. We discuss Archaea in detail in Chapter 17. What phylum of Archaea is common worldwide in soils and in the oceans? Eukarya Plants, animals, and fungi are the most well-known groups of Eukarya. These groups are phylogenetically relatively young com- Mammals Humans pared with Bacteria and Archaea, originating during an evolutionary Vascular burst called the Cambrian explosion, which began about 600 million plants years ago. The first eukaryotes, however, were unicellular microbes. Origin of Earth Microbial eukaryotes, which include diverse algae and protozoa, Animals (4.6 bya) Present may have first appeared as early as 2 billion years ago, well before the origin of plants, animals, and fungi (Section 1.5). The major Earth lineages of Eukarya are traditionally called kingdoms instead of phyla. ste 1 rile There are at least six kingdoms of Eukarya, and this diverse domain 4 bya Bacteria contains microorganisms as well as the plants and animals. bya and Archaea Microbial eukaryotes vary dramatically in size, shape, and physi- Oxygen ology (Figure 1.9). Among the smallest are the nanoflagellates, present Phototrophic which are microbial predators that can be as small as 2 mm long. bacteria cr M In addition, Ostreococcus, a genus of green algae that contains spe- ob ly i ial l on cies whose cells are only 0.8 mm in diameter, are smaller than if e f o r m s 2 3 Oxygen many bacteria. The largest single-celled organisms are eukaryotes, bya bya absent but they are hardly microbial. Xenophyophores are amoeba-like, Eukarya single-celled organisms that live exclusively in the deep oceans and Cyanobacteria Transition to can be up to 10 centimeters in length. In addition, plasmodial slime an oxygenated molds consisting of a single cytoplasmic compartment can be up atmosphere to 30 cm in diameter. In Chapter 18 we consider microbial eukary- (a) otes in detail. Bacteria LUCA Bacteria and Archaea Viruses diverge ~3.8 bya Viruses are not found on the tree of life, and for a variety of reasons, Archaea it can be argued that they are not truly alive. Although viruses can Eukarya diverge from replicate—a hallmark of cells—viruses are obligate parasites that can Archaea ~2.0 bya only replicate within the cytoplasm of a host cell. Viruses are not Eukarya cells, and they lack the cytoplasmic membrane, cytoplasm, and ribo- (b) somes found in all forms of cellular life. Viruses do not carry out metabolic processes; instead, they take over the metabolic systems Figure 1.10 A summary of life on Earth through time and origin of the cellular domains. (a) At its origin, Earth was sterile and anoxic. Cellular life, in the form of of infected cells and turn them into vessels for producing more Bacteria and Archaea, was present on Earth by 3.8 billion years ago (bya). The viruses. Unlike cells, which all have genomes composed of double- evolution of phototrophic bacteria called Cyanobacteria caused Earth’s atmosphere stranded DNA, viruses have genomes composed of DNA or RNA that to become oxygenated over time. While the first evidence for oxygen in Earth’s can be either double- or single-stranded. Viral genomes are often atmosphere appears 2.4 bya, current levels of atmospheric O2 were not achieved quite small, with the smallest having only three genes. The small until 500–800 million years ago. (b) The three domains of cellular organisms are size of most viral genomes means that no genes are conserved Bacteria, Archaea, and Eukarya. Bacteria and Archaea appeared first and Eukarya among all viruses, or between all viruses and all cells. evolved later, diverging from the Archaea. LUCA, last universal common ancestor. M01_MADI4790_16_GE_C01.indd 47 08/03/2021 20:46 48 UNIT 1 THE FOUNDATIONS OF MICROBIOLOGY 1 1.5 Microorganisms and the Biosphere Earth (Figure 1.10a) shows that 80% of life’s history was exclusively UNIT microbial, and thus in many ways, Earth can be considered a micro- Microbes are the oldest form of life on Earth, and they have evolved bial planet. to perform critical functions that sustain the biosphere. In this sec- As evolutionary events unfolded, three major lineages of micro- tion we will learn how microbes have changed our planet and how bial cells—the Bacteria, the Archaea, and the Eukarya (Figure 1.10b)— they continue to do so. were distinguished. All cellular organisms share certain characteristics (Figure 1.5) and as a result, certain genes are found in all cells. For A Brief History of Life on Earth example, approximately 60 genes are universally present in cells of Earth is about 4.6 billion years old, and microbial cells first appeared all three domains. Examination of these genes reveals that all three between 3.8 and 4.3 billion years ago (Figure 1.10). During the first domains have descended from a common ancestor, the last universal 2 billion years of Earth’s existence, its atmosphere was anoxic (O2 common ancestor (LUCA, Figure 1.10b). Over enormous periods of was absent), and only nitrogen (N2 ), carbon dioxide (CO2), and a time, microorganisms derived from these three domains have few other gases were present. Only microorganisms capable of evolved to fill every habitable environment on Earth. anaerobic metabolism (that is, metabolisms that do not require O2) could survive under these conditions. Microbial Abundance and Activity in the Biosphere The evolution of phototrophic microorganisms—organisms that Microorganisms are present everywhere on Earth that will support harvest energy from sunlight—occurred within 1 billion years of the life. They constitute a major fraction of global biomass and are key formation of Earth (Figure 1.10a). The first phototrophs were anoxy- reservoirs of nutrients essential for life. There are an estimated genic (non-oxygen-producing), such as the purple sulfur bacteria 2 * 1030 microbial cells on Earth. To put this number in context, and green sulfur bacteria we know today (Figure 1.11). Cyanobacte- the universe in all its vast extent is estimated to contain merely ria—oxygen-producing (oxygenic) phototrophs (Figure 1.11f )— 7 * 1022 stars. The total amount of carbon present in all microbial evolved nearly a billion years later (Figure 1.10a) and began the slow cells is a significant fraction of Earth’s biomass (Figure 1.12). More- process of oxygenating Earth’s atmosphere. These early phototrophs over, the total amount of nitrogen and phosphorus (essential nutri- lived in structures called microbial mats, which are still found on ents for life) within microbial cells is almost four times that in all Earth today (Figure 1.11a–c). After the oxygenation of Earth’s atmo- plant and animal cells combined. Microbes also represent a major sphere, multicellular life forms eventually evolved, culminating in fraction of the total DNA in the biosphere (about 31%), and their the plants and animals we know today. But plants and animals have genetic diversity far exceeds that of plants and animals. only existed for about half a billion years. The timeline of life on Daniel H. Buckley Daniel H. Buckley Daniel H. Buckley (a) (b) (c) Daniel H. Buckley Norbert Pfennig Norbert Pfennig (d) (e) (f) Figure 1.11 Phototrophic microorganisms. The earli- due to the presence of photopigments. Cyanobacteria imaged by bright-field and phase-contrast microscopy. est phototrophs lived in microbial mats. (a) Microbial form the green layer nearest the surface, purple sulfur Purple and green sulfur bacteria are anoxygenic mats in the Great Sippewissett Marsh, a salt marsh in bacteria form the purple and yellow layers below, and phototrophs that appeared on Earth long before Massachusetts, USA. (b) Mats develop a cohesive green sulfur bacteria form the bottommost green layer. oxygenic phototrophs (that is, Cyanobacteria) evolved structure that forms at the sediment surface. (c) A The scale on the knife is in cm. (d) Purple sulfur bacte- (see Figure 1.10a). slice through the mat shows colored layers that form ria, (e) green sulfur bacteria, and (f) cyanobacteria M01_MADI4790_16_GE_C01.indd 48 08/03/2021 20:46 CHAPTER 1 The Microbial World 49 Element Major cellular sources zones” cause massive mortality of fish and shellfish in coastal oceans 1