Chapter 6: Transcription of Genes PDF
Document Details
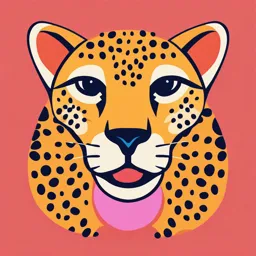
Uploaded by MasterfulElf6815
Tags
Related
- Molecular Genetics: Transcription I PDF
- Chapter 6 How Cells Read the Genome: Molecular Biology of The Cell PDF
- Molecular Biology Instant Notes PDF
- Human Genetics and Molecular Biology Notes PDF
- Molecular Biology Transcription Class 17 Questions - Exam Questions PDF
- Central Dogma Of Molecular Biology PDF
Summary
This chapter explores the process of transcription, a crucial mechanism in molecular biology. It details how genetic information is transcribed from DNA to RNA, primarily focusing on the role of RNA polymerase and its involvement in various types of transcription. The process is examined in the context of both prokaryotic and eukaryotic organisms.
Full Transcript
C H A P T E R S I X Transcription of Genes Genes are Expressed by Making RNA Short Segments of the Chromosome Are Turned into Messages Terminology: Cistrons, Coding Sequences and Open Reading Frames How Is the Beginning of a Gene Recognized? Manufacturing the Message RNA Polymerase K...
C H A P T E R S I X Transcription of Genes Genes are Expressed by Making RNA Short Segments of the Chromosome Are Turned into Messages Terminology: Cistrons, Coding Sequences and Open Reading Frames How Is the Beginning of a Gene Recognized? Manufacturing the Message RNA Polymerase Knows Where to Stop How Does the Cell Know Which Genes to Turn On? What Activates the Activator? Negative Regulation Results from the Action of Repressors Many Regulator Proteins Bind Small Molecules and Change Shape Transcription in Eukaryotes Is More Complex Transcription of rRNA and tRNA in Eukaryotes Transcription of Protein-Encoding Genes in Eukaryotes Upstream Elements Increase the Efficiency of RNA Polymerase II Binding Enhancers Control Transcription at a Distance 132 Genes are Expressed by Making RNA 133 ds DNA Gene of interest STRANDS ARE LOCALLY PULLED APART Template strand Coding strand NEW RNA STRAND IS MADE FIGURE 6.01 Transcription in Its Simplest Form 3' 5' RN The two strands of the DNA to be New m A tr single s and 3' transcribed are separated locally. 5' The top strand serves as a template for building a new RNA molecule. 5' 3' Genes are Expressed by Making RNA DNA “merely” stores genetic For a cell to operate, its genes must be expressed. The word “expressed” means that information. Putting the the gene products, whether proteins or RNA molecules, must be made. The DNA mol- information to use requires ecule that carries the original copy of the genetic information is used to store genetic RNA and (usually) protein. information but is not used as a direct source of instructions to run the cell. Instead, working copies of the genes, made of RNA, are used. The transfer of information from DNA to RNA is known as transcription and RNA molecules are therefore sometimes referred to as transcripts. Genes may be subdivided into two major groups: those whose final product is an RNA molecule (e.g., tRNA, rRNA, assorted regulatory RNAs—see below) and those whose final product is protein. In the latter case, the RNA transcript acts as an intermediary and a further step is needed to convert the information carried Messenger RNA carries the by the RNA to protein. This process is discussed in Ch. 8. The type of RNA molecule information for making that carries genetic information encoding a protein from the genes into the rest of the proteins from the genes to the cell is known as messenger RNA, or mRNA. Since the great majority of genes encode cytoplasm. proteins, we will deal with these genes first. For a gene to be transcribed, the DNA, which is double stranded, must first be pulled apart temporarily, as shown in Figure 6.01. Then, RNA is made by RNA poly- merase. This enzyme binds to the DNA at the start of a gene and opens the double helix. Finally, it manufactures an RNA molecule. The sequence of the RNA message is complementary to the template strand of The DNA double helix must be opened up for RNA the DNA from which it is synthesized. Apart from the replacement of thymine in DNA polymerase to read the with uracil in RNA, this means that the sequence of the new RNA molecule is iden- template strand and make tical to the sequence of the coding strand of DNA; that is, the strand not actually used RNA. as a template during transcription. Note that RNA, like DNA, is synthesized in the 5¢- to 3¢- direction (Fig. 6.02). Other names for the template strand are the non-coding or anti-sense strand; other names for the coding strand are non-template or sense strand. Only one of the strands of DNA is copied in any given transcribed region. [But note coding strand The strand of DNA equivalent in sequence to the messenger RNA (same as plus strand) messenger RNA (mRNA) The molecule that carries genetic information from the genes to the rest of the cell RNA polymerase Enzyme that synthesizes RNA using a DNA template transcription Process by which information from DNA is converted into its RNA equivalent template strand Strand of DNA used as a guide for synthesizing a new strand by complementary base pairing 134 CHAPTER SIX Transcription of Genes Template strand 5' 3' 3' mR RNA NA polymerase 3' 5' 5' DNA Direction of synthesis Coding strand FIGURE 6.02 Naming the Basic Components Involved in Transcription The DNA is shown in its double helical form. After local separation of the strands, the new RNA is synthesized so that it base pairs with one of the DNA strands—the template strand. The other DNA strand is inactive and is called the coding strand. The enzyme RNA polymerase synthesizes single- stranded RNA in the 5¢- to 3¢-direction. The sequence of bases in the RNA is the same as in the coding strand of DNA, except that uracil substitutes in RNA for thymine in DNA. The bases of the mRNA are complementary to those of the template DNA strand; note that uracil base pairs with adenine. that the two different strands of the DNA may each be used as templates in different regions of the chromosome.] Short Segments of the Chromosome Are Turned into Messages Although a chromosome carries hundreds or thousands of genes, only a fraction of these are in use at any given time. In a typical bacterial cell, about 1,000 genes, or about 25% of the total, are expressed under any particular set of growth conditions. Some genes are required for the fundamental operations of the cell and are therefore expressed under most conditions. These are known as housekeeping genes. Other genes vary in expression in response to changes in the environment. During cell growth Each mRNA carries and metabolism, each gene or small group of related genes is used to generate a sepa- information from only a short rate RNA copy when, and if, it is needed. Consequently, each cell contains many dif- stretch of the DNA. ferent RNA molecules, each carrying the information from a short stretch of DNA. In the cells of higher organisms, which have many more genes than do bacteria, the proportion of genes in use in a particular cell at a particular time is much smaller. Different cells of multi-cellular organisms express different selections of genes depend- ing on their specialized roles. For example, in the human female, genes related to the menstrual cycle are largely unique and expressed in a timed sequence to provide functionality to the organism. In addition, gene expression varies with the stage of development. Embryonic genes are often expressed only at certain times. Thus, the control of gene expression is much more complex in higher organisms, although the basic principles are the same. Terminology: Cistrons, Coding Sequences and Open Reading Frames A cistron is a structural gene, which is a coding sequence or segment of DNA encod- ing a polypeptide. It was defined originally as a genetic unit by complementation using the cis/trans test. Nowadays, the terms cistron and structural gene also include DNA sequences that code for RNA molecules that function as RNA without being trans- lated into proteins (e.g., rRNA, tRNA, snRNA, etc.). An open reading frame (ORF) cistron Segment of DNA (or RNA) that encodes a single polypeptide chain housekeeping genes Genes that are switched on all the time because they are needed for essential life functions open reading frame (ORF) Sequence of bases (either in DNA or RNA) that can be translated (at least in theory) to give a protein structural gene Sequence of DNA (or RNA) that codes for a protein or for an untranslated RNA molecule How Is the Beginning of a Gene Recognized? 135 EUKARYOTES PROKARYOTES DNA Promoter Structural gene DNA Promoter Structural genes Operon TRANSCRIPTION FIGURE 6.03 Monocistronic Versus Polycistronic mRNA Monocistronic mRNA Polycistronic mRNA The typical situation in eukaryotes is to have one structural gene produce monocistronic RNA and this, in TRANSLATION turn, be translated into a single protein. In bacteria, it is common to see several structural genes transcribed under the control of a single promoter. The RNA produced is polycistronic and yields several separate proteins. Single protein Several proteins is any sequence of bases (in DNA or RNA) that could, in theory, encode a protein. The ORF is “open” in the sense that it does not contain any stop codons that would interrupt its translation into a polypeptide chain (although, of course, every ORF ends in a stop codon). (Any cistron that encodes a protein must also be an ORF, whereas a cistron that encodes an untranslated RNA is not an ORF.) In eukaryotes, each mRNA In eukaryotes, each gene is transcribed to give a separate mRNA and each mRNA carries only a single gene. In molecule therefore encodes the information for only a single protein and is known as prokaryotes, several genes monocistronic mRNA (Fig. 6.03). In bacteria, clusters of related genes, known as may be carried on the same operons, are often found next to each other on the chromosome and are transcribed mRNA. together to give a single mRNA, which is therefore called polycistronic mRNA. Thus, a single bacterial mRNA molecule may encode several proteins, usually with related functions, such as the enzymes that oversee the successive steps in a metabolic pathway. How Is the Beginning of a Gene Recognized? Transcription will first be described in bacteria because it is simpler. The principles of Before starting transcription, transcription are similar in higher organisms, but the details are more complicated, as RNA polymerase binds to the will be shown below. The major differences between prokaryotes and eukaryotes occur promoter, a recognition sequence in front of the gene. in the initiation and regulation of transcription, rather than in the actual synthesis of RNA. In front of each gene is a region of regulatory DNA that is not itself transcribed. This contains the promoter, the sequence to which RNA polymerase binds (Fig. 6.04), together with other sequences involved in the control of gene expression. This stretch of DNA in front of a gene (i.e., at the 5¢-end) is often referred to as the upstream region. Note also that the first base of the mRNA of a protein-encoding gene is not the first base of the protein coding sequence. Between these two points there is a short stretch known as the 5¢-untranslated region, or 5¢-UTR, meaning it will not be trans- lated to form protein (Fig. 6.04). At the far end of the mRNA there is another short 5¢-untranslated region (5¢-UTR) Region of an mRNA between the 5¢-end and the translation start site monocistronic mRNA mRNA carrying the information of a single cistron, that is a coding sequence for only a single protein operon A cluster of prokaryotic genes that are transcribed together to give a single mRNA (i.e. polycistronic mRNA) polycistronic mRNA mRNA carrying the information of multiple cistrons, that is coding sequences for several proteins promoter Region of DNA in front of a gene that binds RNA polymerase and so promotes gene expression upstream region Region of DNA in front (i.e. beyond the 5¢-end) of a structural gene; its bases are numbered negatively counting backwards from the start of transcription 136 CHAPTER SIX Transcription of Genes Upstream region Downstream region DNA Promoter Gene -35 -10 +1 5'UTR mRNA 3'UTR Start and end of protein coding sequence Start of transcript End of transcript FIGURE 6.04 Upstream and Downstream Regions Genes and their regulatory regions are divided into upstream and downstream portions. The upstream portion contains the promoter. The downstream region begins with the information for the 5¢-untranslated component, then the structural gene. The messenger RNA begins with the 5¢- untranslated region (5¢-UTR), then the coding sequence for the protein. Transcription begins by definition at the first base after the promoter. The upstream region, including the promoter, is given negative numbers counting backward from the beginning of transcription. region, beyond the end of the protein coding sequence, that is not translated. This is the 3¢-untranslated region, or 3¢-UTR. Bacterial RNA polymerase consists of two major components, the core enzyme The sigma subunit of bacterial (itself made of four subunits) and the sigma subunit. The core enzyme is responsible RNA polymerase recognizes for RNA synthesis whereas the sigma subunit is largely responsible for recognizing the promoter. The core enzyme the promoter. The sigma subunit, recognizes two special sequences of bases in the makes RNA. promoter region of the coding (non-template) strand of the DNA (Fig. 6.05). These are known as the -10 sequence and the -35 sequence because they are found by count- ing backward approximately 10 and 35 bases, respectively, from the first base that is transcribed into mRNA. [Previously, the -10 sequence was known as the Pribnow box, after its discoverer. This name is rarely used nowadays.] The consensus sequence for the -10 sequence is TATAA and the consensus sequence at -35 is TTGACA. (Consensus sequences are found by comparing many Strong promoters usually have sequences and taking the average.) Although a few highly expressed genes do have sequences close to consensus. the exact consensus sequences in their promoters, the -10 and -35 region sequences are rarely perfect. However, as long as they are wrong by only up to three or four bases, the sigma subunit will still recognize them. The strength of a promoter depends partly on how closely it matches the ideal consensus sequence. Strong promoters are highly expressed and are often close to consensus. Promoters further away from the consensus sequence will be expressed only weakly (in the absence of other factors— but see below). In practice, consensus sequences for regulatory sites on DNA such as promoters will vary from one group of organisms to another. Thus, the -10 and -35 consensus sequences given above are for Escherichia coli and related bacteria. Both the consen- sus sequences and the proteins that recognize them will diverge in more distantly Promoter sequences vary in different organisms. related organisms. This is of practical importance when genes from one organism are expressed in another as a result of biotechnological manipulations. Consequently, it is -10 region Region of bacterial promoter 10 bases back from the start of transcription that is recognized by RNA polymerase 3¢-untranslated region (3¢-UTR) Sequence at the 3¢-end of mRNA, downstream of the final stop codon, that is not translated into protein -35 region Region of bacterial promoter 35 bases back from the start of transcription that is recognized by RNA polymerase core enzyme Bacterial RNA polymerase without the sigma (recognition) subunit Pribnow box Another name for the -10 region of the bacterial promoter sigma subunit Subunit of bacterial RNA polymerase that recognizes and binds to the promoter sequence Manufacturing the Message 137 FIGURE 6.05 Sigma Core Recognizes the -10 and enzyme -35 Sequences Coding Sigma Sigma strand The sigma protein binds to both the of TTGACA TATAAT Gene DNA -10 and -35 sequences of the -35 -10 +1 promoter, thereby establishing a constant position with respect to the Promoter region start of transcription. FIGURE 6.06 Elongation of Template strand nscription bubbl the mRNA Tra e The beginning of RNA synthesis is 3' T G A TGC RNA A CU A CG polymerase shown. The DNA strands have separated at the transcription bubble. Synthesis of six bases of Sequence of Direction of synthesis RNA complementary to those of the growing RNA 5' DNA template strand occurs while chain RNA polymerase remains at the promoter site. often helpful to supply consensus promoters (or other regulatory sequences) that work well in the host organism to achieve high expression of a cloned gene from a foreign source. The use of “expression vectors” to optimize gene expression during cloning is discussed in more detail in Ch. 22. Manufacturing the Message Once the sigma subunit has bound to a promoter, the RNA polymerase core enzyme opens up the DNA double helix locally to form the transcription bubble. Note that the RNA polymerase opens up the -10 sequence, TATAAT, consists of AT base pairs and this assists in the melting of the DNA to form the transcription bubble. DNA into single strands.After the DNA helix has been opened, a single strand of RNA is generated using one of the DNA strands as a template for matching up the bases. Once the RNA polymerase has bound to the DNA and initiated a new strand of RNA, the sigma subunit is no longer needed and often (though not always) detaches from the DNA, leaving behind the core enzyme. The RNA polymerase actually remains at the promoter until the new strand is eight or nine bases long. At this point, sigma leaves and the core enzyme is free to move forward and elongate the mRNA (Fig. 6.06). The first transcribed base of the mRNA is normally an A (as in Fig. 6.06). This special A is usually flanked by two pyrimidines, most often giving the sequence CAT. Sometimes the first transcribed base is a G, but almost never a pyrimidine. Synthesis of mRNA is from 5¢ to 3¢ and proceeds at about 40 nucleotides per second. This is The core enzyme moves much slower than DNA replication (~1,000 bp/sec), but roughly equivalent to the rate ahead, manufacturing RNA of polypeptide synthesis (15 amino acids per second). and leaving sigma behind at The core enzyme of RNA polymerase consists of four subunits, two a plus b and the promoter. b¢ (Fig. 6.07). The b and b¢ subunits comprise the catalytic site of the enzyme. The a subunit is required partly for assembly and partly for recognizing promoters. RNA polymerase has a deep groove through the middle that can accommodate about 16 bp of DNA in the case of bacteria and about 25 bp in the case of eukaryotes such as yeast, whose RNA polymerase is larger. A thinner groove, roughly at right angles to the first, may hold the newly constructed strand of RNA. transcription bubble Region where DNA double helix is temporarily opened up so allowing transcription to occur 138 CHAPTER SIX Transcription of Genes A GENE SUBUNIT FUNCTION NAME -assembles enzyme 2 a subunits rpoA -recognizes promoter (40 kD each) -binds some activators } b subunit rpoB (155 kD) enzymatically C promotes RNA synthesis b' subunit rpoC (160 kD) RNA groove s subunit recognizes promoter rpoD A (32-90 kD) RN B Direction of synthesis DNA Unwinding Rewinding DNA DNA DNA DNA groove coding strand DNA template strand A RN Binding site Catalytic site FIGURE 6.07 Structure of RNA Polymerase A) Bacterial RNA polymerase has four types of subunits and three functional specificities. B) Topography of functions performed by RNA polymerase. C) The structure of yeast RNA polymerase reveals a groove that may be used by DNA as it moves relative to the polymerase and a potential groove for the newly formed RNA. The negative supercoiling of the chromosome promotes opening of DNA during transcription. As RNA polymerase moves along the DNA, it winds the DNA more tightly ahead of itself, creating positive supercoils. It also leaves partly unwound DNA behind, which generates negative supercoils. To restore normal levels of supercoiling, DNA gyrase inserts negative supercoils ahead of RNA polymerase and topoisomerase I removes negative supercoils behind RNA polymerase (see Ch. 4). RNA Polymerase Knows Where to Stop Just as there is a recognition site at the front of each gene, so there is a special termi- nator sequence at the end. The terminator is in the template strand of DNA and con- The end of a gene is marked sists of two inverted repeats separated by half a dozen bases, and followed by a run by a terminator sequence that of A’s. The sequence of the mRNA will be the same as the non-template strand of forms a hairpin structure in the DNA except for the substitution of U for T. Thus the string of A’s in the DNA tem- RNA. plate strand gives rise to a run of U’s at the 3¢-end of the mRNA (Fig. 6.08). Note that in the DNA, the two inverted repeat sequences are on opposite strands. Although researchers often talk as if the mRNA has inverted “repeats,” its second “repeat” is actually the complement of the first. Because of this, such inverted repeats on the terminator DNA sequence at end of a gene that tells RNA polymerase to stop transcribing RNA Polymerase Knows Where to Stop 139 A DNA: Inverted Coding strand N N N N - TA G C G G C C AT C - N N N N N N N N N - G AT G G C C G C TA - T T T T T T T Template strand N N N N - AT C G C C G G TA G - N N N N N N N N N - C TA C C G G C G AT- A A A A A A A (N = any base) Repeats TRANSCRIPTION Messenger RNA NNNN-UAGCGGCCAUC-NNNNNNNNN-GAUGGCCGCUA-UUUUUUU B G U U Loop U A C C A A C G U A A U C G C G Stem: G C (base pairing of inverted repeats) G C C G G C Terminator A U U A Messenger RNA - - - A U U A U U U U U U U - - - 3' end FIGURE 6.08 The Terminator Sequence is Transcribed into RNA A) The signal for RNA polymerase to stop is shown in both the DNA and the RNA transcribed from it. The terminator consists of an inverted repeat separated by approximately 10 bases from a run of U’s. B) The complementary bases form the stem of the hairpin, with the intervening bases forming the loop. same strand of an RNA molecule can pair up to generate a stem and loop or “hairpin” structure (Fig. 6.08). Once the RNA polymerase reaches the stem and loop, it pauses. Long RNA mol- ecules contain many possible hairpin structures that cause RNA polymerase to slow down or stop briefly, depending on the size of the hairpin. This provides an opportu- nity for termination, but if there is no string of U’s, the RNA polymerase will start off again. However, a string of U’s paired with a string of A’s in the template strand of DNA is a very weak structure, and the RNA and DNA fall apart while the RNA poly- merase is idling (Fig. 6.09). Pausing varies in length, but is around 60 seconds for a typical terminator. Termination may actually occur at several possible positions in the middle or end of the run of U’s. In other words, the RNA polymerase “stutters” and the precise location of termination may vary slightly between different molecules of the same mRNA. Once the DNA and RNA have separated at the terminator structure, the RNA polymerase falls off and departs to find another gene (Fig. 6.09). Two classes of terminators exist. Rho-dependent terminators need Rho (r) protein to separate the RNA polymerase from the DNA. Rho-independent, or “intrinsic,” ter- minators do not need Rho or any other factor to cause termination. Most terminators in E. coli do not need Rho. In contrast, Rho-dependent terminators are relatively frequent in bacteriophages. A sub-class of terminators Rho protein is a specialized helicase that uses energy from ATP to unwind a require a recognition protein, known as Rho, to function. DNA/RNA hybrid double helix. It consists of a hexamer of six identical subunits that recognizes and binds to a sequence of 50 to 90 bases located upstream of the termi- Rho (r) protein Protein factor needed for successful termination at certain transcriptional terminators Rho-dependent terminator Transcriptional terminator that depends on Rho protein Rho-independent terminator Transcriptional terminator that does not need Rho protein 140 CHAPTER SIX Transcription of Genes mRNA AAAAA DNA template strand UUUUU RNA polymerase Hairpin DISASSEMBLY AAAAA RNA FIGURE 6.09 Termination polymerase of Messenger RNA When the mRNA reaches the hairpin of the terminator it pauses; when it reaches the AAAAA UUUUU sequence it falls off the template strand along with the newly synthesized RNA. nator in the mRNA. The Rho hexamer does not form a closed ring, but instead is split open and resembles a lock washer in structure. The RNA sequence for Rho binding is poorly defined but is high in C and low in G. Rho can only bind to the growing mRNA chain once the RNA polymerase has synthesized the C-rich/G-poor recognition region and moved on. Rho moves along the RNA transcript and catches up with the RNA polymerase at the terminator stem and loop structure where the RNA polymerase pauses (Fig. 6.10). Rho then unwinds the DNA/RNA helix in the transcription bubble and separates the two strands. How Does the Cell Know Which Genes to Turn On? Some genes, known as housekeeping genes, are switched on all the time; i.e., they are expressed “constitutively.” In bacteria, these often have both their -10 and -35 region promoter sequences very close or identical to consensus. Consequently, they are always recognized by the sigma subunit of RNA polymerase and are expressed under all con- Housekeeping genes are ditions. Other constitutive promoters are further from consensus and expressed less switched on all the time. strongly. Nonetheless, if only relatively low amounts of the gene product are needed, this is acceptable. Genes that are only needed under certain conditions sometimes have poor re- cognition sequences in the -10 and -35 regions of their promoters. In such cases, the Some genes need activator promoter is not recognized by sigma unless another accessory protein is there to help proteins to switch them on. (Fig. 6.11). These accessory proteins are known as gene activator proteins and are dif- ferent for different genes. Each activator protein may stimulate the transcription of one or more genes. A group of genes that are all recognized by the same activator protein will be expressed together under similar conditions, even if the genes are at different places on the DNA. Higher organisms have many genes that are expressed differently activator protein Protein that switches a gene on constitutive gene Gene that is expressed all the time What Activates the Activator? 141 FIGURE 6.10 Termination by Rho Rho first binds to the growing messenger RNA. When the RNA polymerase pauses at the termination site, Rho catches up and untwists the newly formed mRNA strand from the DNA. Subsequently, the mRNA and RNA polymerase fall off the DNA and Rho detaches from the mRNA. in different tissues. As a result, eukaryotic genes are often controlled by multiple acti- vator proteins, more specifically known as transcription factors (see below). What Activates the Activator? Long ago, the Greek philosopher Plato pondered the political version of this question: “Who will guard the guardians?” In living cells, especially in more complex higher transcription factor Protein that regulates gene expression by binding to DNA in the control region of the gene 142 CHAPTER SIX Transcription of Genes Activator protein DNA Gene to be transcribed -35 -10 +1 FIGURE 6.11 Gene Activator Proteins The activator protein first binds to Activator the promoter region of the gene. protein Once bound, the activator protein RNA polymerase facilitates the binding of the RNA DNA Gene to be transcribed polymerase. Gene transcription then commences. -35 -10 +1 FIGURE 6.12 MalT Changes Shape upon Empty Binding Maltose MalT Maltose protein The MalT protein has a binding site complementary in shape to the sugar maltose. In step 1, MalT binds to maltose, which causes MalT to change shape. In step 2, STEP 1 the new conformation of MalT protein allows it to bind to DNA at a specific sequence found only in certain promoters. The gene thus Occupied activated is involved in the MalT metabolism of maltose. protein STEP 2 MalT activator protein Genes for use DNA Promoter of maltose -35 -10 +1 organisms, there may indeed be a series of regulators, each regulating the next. What is the initial event? The cell must respond to some outside influence or must be influ- enced by other internal processes. The regulation of gene expression will be consid- ered in more detail in Chapters 9 and 10. This chapter will be limited to a discussion of the basic mechanisms needed for a promoter to be functional. As a simple example of an activator, consider the use of maltose by Escherichia Activator proteins often change coli. Maltose is a sugar made originally from the starch in malt and many other sources. shape in response to small It can be used by E. coli to satisfy all of its needs for energy and organic material. An molecules. Only one activator protein, MalT, detects maltose and binds to it (Fig. 6.12). This causes the MalT conformation binds to DNA. protein to change shape, exposing its DNA-binding site. The original “empty” form of MalT cannot bind to DNA. The active form (MalT + maltose) binds to a specific sequence of DNA found only in the promoter region of genes needed for growth on maltose. The presence of MalT helps RNA polymerase bind to the promoter and transcribe the genes. The small molecule, in this case maltose, which causes gene Negative Regulation Results from the Action of Repressors 143 Empty Lacl in operator site DNA Gene -35 -10 -5 +1 +21 Operator Promoter Inducer molecules FIGURE 6.13 Principle of Negative Regulation by a Repressor The LacI protein is bound to the Lacl operator site, within the promoter region of a gene that affects lactose metabolism. The inducer binds to RNA LacI, changing its conformation and polymerase causing its release from the DNA. Gene DNA The RNA polymerase is then free to transcribe the gene. -35 -10 -5 +1 +21 expression is known as the inducer. The result of this is that the genes intended for using maltose are only induced when this particular sugar is available. The same general principle applies to most nutrients, although the details of the regulation may vary from case to case. Negative Regulation Results from the Action of Repressors Genes may be controlled by positive or negative regulation. In positive regulation, an activator protein binds to the DNA only when the gene is to be turned on. In nega- Repressors are proteins that tive regulation, a repressor protein binds to the DNA and insures that the gene is switch genes off. turned off. Only when the repressor is removed from the DNA can the gene be tran- scribed. The site where a repressor binds is called the operator sequence. Like activa- tor proteins, repressor proteins alternate between DNA-binding and nonbinding forms. In this case, binding of the inducer to the repressor causes it to change from its DNA-binding form to the nonbinding form. Historically, negative regulators were discovered before activators.The best known example is the lactose repressor, the LacI protein (Fig. 6.13). Lactose is another sugar, found in milk, on which bacteria such as E. coli can grow. When no lactose is available, the LacI protein binds to its operator sequence, which overlaps part of the promoter and the front part of the coding region for the genes for using lactose. When lactose is present, the LacI protein changes shape and is released from the DNA and the lactose genes are induced. Overall, the result is the same as for maltose: when lactose is available, the genes for using it are switched on and when there is no lactose, the genes are turned off. inducer Small signal molecule that binds to a regulatory protein and thereby causes a gene to be switched on LacI protein Repressor that controls the lac operon negative regulation Regulatory mode in which a repressor keeps a gene switched off until it is removed operator Site on DNA to which a repressor protein binds positive regulation Control by an activator that promotes gene expression when it binds repressor Regulatory protein that prevents a gene from being transcribed 144 CHAPTER SIX Transcription of Genes Signal Signal binding site molecule NON-BINDING FORM DNA binding site FIGURE 6.14 Allosteric Protein Binds a Signal Molecule and Changes Shape The two subunits shown have a signal-binding site and a DNA- DNA BINDING binding site. When the signal FORM molecule binds to the subunits, they pair and change conformation They DNA are then able to bind to DNA. recognition site The detailed mechanism by which repressors prevent transcription varies consid- erably and is often unknown. The repressor sometimes blocks the binding of RNA polymerase to the promoter, simply by getting in the way (steric hindrance). An example is the well-studied CI repressor of bacteriophage lambda. Sometimes the repressor may bind further downstream, inside the structural gene. In this case, RNA polymerase can still bind to the promoter but is prevented from moving forward and transcribing the gene. Sometimes, even though their binding sites in the DNA sequence overlap, the RNA polymerase and the repressor both bind the DNA simultaneously. [Remember that the DNA double helix is 3-dimensional and that two proteins may therefore bind to the same linear segment, if they occupy separate locations around its surface.] Indeed, an example of this is the LacI repressor. In this case the RNA polymerase actually binds more tightly in the presence of repressor, but is locked in place and cannot open the DNA to initiate transcription. Many Regulator Proteins Bind Small Molecules and Change Shape Whether a regulator protein is an activator or a repressor, it needs a signal of some sort. Small molecules may control One of the most common ways to do this is by using some small molecule that fits into gene expression by binding to regulatory proteins. a binding site on the regulatory protein (Fig. 6.14). This is called the signal molecule. In the case of using a nutrient for growth, an obvious and common choice is the nutri- ent molecule itself. [In prokaryotes the DNA binding protein often binds the signal molecule directly. In eukaryotes, where the DNA is inside the nucleus, things are often more complex, and multiple proteins are involved. The signal molecule is often bound by proteins in the cell membrane or cytoplasm and the signal is then transmitted to the nucleus. The DNA binding protein itself normally stays in the nucleus and upon receiv- ing the signal, is converted to its DNA-binding form by phosphorylation.] When a regulator protein binds its signal molecule, it changes shape (Fig. 6.14). Regulator proteins have two alternative forms, the DNA-binding form and the non- binding form. Binding or loss of the signal molecule causes the larger protein to flip- flop between its two alternative shapes. Proteins that change in activity by changing signal molecule Small molecule that exerts a regulatory effect by binding to a regulatory protein Transcription in Eukaryotes Is More Complex 145 FIGURE 6.15 Regulator Binds at an Inverted Repeat—Principle At sites where regulator proteins bind there is often an inverted repeat with both DNA strands participating as shown. If the subunits of regulator protein are identical, they each recognize one of the inverted repeats and pair so that the same regions of each subunit face each other. shape in this manner are called allosteric proteins. Examples include some enzymes, transport proteins and regulators. Allosteric proteins have multiple subunits that change shape in concert (Fig. 6.14). Usually there is an even number of subunits, most often two or four. All of the subunits bind the signal molecule and then they all change shape together. Since there is an even number of protein subunits, the recognition site on the DNA Recognition sites on DNA are for regulator proteins is often duplicated. In this case, the recognition site is usually an often inverted repeats. Separate subunits of the inverted repeat, often referred to as a palindrome. This is because the subunits of the regulator protein each bind regulator protein bind to each other head to head rather than head to tail (Fig. 6.15). one of the repeat sequences. Consequently, the two protein molecules are pointing in opposite directions. Because they have identical binding sites for DNA, they recognize the same sequence of bases but in opposite directions on the two strands of the DNA. The two half-sites are usually separated by a spacer region of several bases, whose identity is free to vary. The two half-sequences of such recognition sites are not always exact matches. One regulator protein subunit binds to the recognition sequence on the template strand of the DNA double helix, and its partner binds to the same sequence but on the non-template strand of the DNA pointing in the opposite direction. This is simpler in practice than it sounds, precisely because the DNA molecule is helical. Although the two recognition sequences are on different strands of DNA, they end up on the same face of the DNA molecule due to its helical twisting (Fig. 6.15). An example of a palindromic recognition site is the lac operator sequence which is bound by the LacI repressor (a tetramer). This sequence runs from -6 to +28 rela- tive to the start of transcription. It is not exactly symmetrical. The two half sequences are: TGTGTGgAATTGTgA and, running in the opposite sense on the other strand, TGTGTGaAATTGTtA (capital letters indicate matching bases). The two half-sites are separated by five base pairs. The left hand half of this site binds the LacI protein more strongly than the right hand side. A stronger operator sequence could be gener- ated by changing the right hand half-site to exactly match the left. Transcription in Eukaryotes Is More Complex Since typical eukaryotic cells have 10 times as many genes as do bacteria, the whole process of transcription and its regulation is more complex. For a start, eukaryotes have Eukaryotes have three RNA three different RNA polymerases, unlike bacteria which have just one. The three RNA polymerases that specialize in polymerases transcribe different categories of nuclear genes. In addition, mitochondria which type of genes they and chloroplasts have their own RNA polymerases, which resemble the bacterial transcribe. enzyme. allosteric protein Protein that changes shape when it binds a small molecule 146 CHAPTER SIX Transcription of Genes Non-transcribed space gene for gene for gene for DNA 45S RNA 45S RNA 45S RNA TRANSCRIPTION FIGURE 6.16 Clusters of Ribosomal RNA Genes 45S RNA 45S RNA 45S RNA The genes for rRNA are located at multiple sites along the DNA. A single transcribed unit of DNA PROCESSING yields an initial RNA molecule of 45S. The 45S RNA is processed to yield the final 18S and 28S subunits of rRNA. 18S rRNA 28S rRNA RNA polymerase I transcribes the genes for the two large ribosomal RNA mole- cules and RNA polymerase III transcribes the genes for tRNA, 5S rRNA and a few other small RNA molecules. RNA polymerase II transcribes most eukaryotic genes that encode proteins and as a result is subject to the most complex regulation. Since ribosomal RNA and transfer RNA are needed all the time by all types of cells, RNA polymerases I and III operate constitutively in most cell types. Many transcription factors are A variety of proteins, known as transcription factors are also needed for the correct involved in controlling gene functioning of RNA polymerases. Transcription factors may be divided into general expression in eukaryotes. transcription factors and specific transcription factors. General transcription factors are needed for the transcription of all genes transcribed by a particular RNA polymerase, and are typically designated TFI, TFII, TFIII followed by individual letters. The I, II, and III refer to the corresponding RNA polymerase (see below). Specific transcrip- tion factors are needed for transcription of particular specific gene(s) under specific circumstances. [Proteins such as the sigma subunit of bacterial RNA polymerase may also be regarded as transcription factors, however, this terminology is usually only used for eukaryotes.] Transcription of rRNA and tRNA in Eukaryotes The genes for the two large ribosomal RNAs are present in multiple copies, from seven in E. coli to several hundred in higher eukaryotes (Fig. 6.16). In bacteria, the copies are dispersed, but in eukaryotes they form clusters of tandem repeats. In humans, there are clusters of rRNA genes on five separate chromosomes. The 18S and the 28S rRNA are transcribed together as a single large RNA (45S RNA) that is cleaved to Eukaryotes contain many release the two separate ribosomal RNA molecules. Between these transcription units copies of the genes for are non-transcribed spacer regions. In eukaryotic cells, the rRNA genes have their own ribosomal RNA. These are RNA polymerase to transcribe them, RNA polymerase I. found in clusters and are Synthesis of rRNA is localized to a special zone of the nucleus known as the nucle- transcribed by RNA olus. Here the rRNA precursor is both transcribed and processed into 18S and 28S polymerase I. rRNA. These rRNA molecules then bind proteins, giving ribonucleo-protein particles. This yields a dense granular region when seen under the microscope (Fig. 6.17). The segments of chromosomes associated with the nucleolus were named “nucleolar organ- izers.” It is now known that these correspond to the clusters of rRNA genes. nucleolar organizer Chromosomal region associated with the nucleolus; actually a cluster of rRNA genes RNA polymerase I Eukaryotic RNA polymerase that transcribes the genes for the large ribosomal RNAs RNA polymerase II Eukaryotic RNA polymerase that transcribes the genes encoding proteins RNA polymerase III Eukaryotic RNA polymerase that transcribes the genes for 5S ribosomal RNA and transfer RNA transcription factor Protein that regulates gene expression by binding to DNA in the control region of the gene Transcription of rRNA and tRNA in Eukaryotes 147 (a) FIGURE 6.17 Ribosomal RNA is Made in the Nucleolus A) Electron micrograph of a thin- sectioned nucleolus from a mouse cell fixed in situ. Black arrows indicate peri-nucleolar condensed chromatin and the asterisk shows dense fibrillar components (d) clumping around fibrillar centers (f). (b) Granular regions (g) of newly made ribonucleoproteins are also marked. Image provided by Ulrich Scheer, University of Würzburg. B) Spread Christmas tree structure (4 microns long) from a mouse cell is shown at the same magnification as (A). Bar represents 0.5 micron. From: Raska I., Oldies but goldies: searching for Christmas trees within the nucleolar architecture. Trends in Cell Biology TRENDS in Cell Biology 13 (2003) 517–525. Although most promoters are AT rich, presumably because the weaker base pairs help in opening up the DNA, the promoter for RNA polymerase I is unusual in con- taining many GC pairs. There are two GC-rich regions, the core promoter and the upstream control element, that are 80 to 90 percent identical in sequence (Fig. 6.18). Both are recognized by protein UBF1 (Upstream Binding Factor 1), a single polypep- tide. After UBF1 has bound, another protein, selectivity factor SL1, binds next to it. SL1 consists of four polypeptides, one of which, TBP (TATA Binding Protein), is also required for RNA polymerases II and III (see below). Once UBF1 and SL1 are in place, RNA polymerase I can bind. It is uncertain how the binding of UBF1 and SL1 at the upstream control element helps initiation in the case of RNA polymerase I. However, in similar cases, the DNA is known to bend around, bringing the upstream element into direct contact with the promoter region. RNA polymerase III is responsible for making 5S rRNA and transfer RNA. It also makes some small nuclear RNAs, while other snRNAs are transcribed by RNA polymerase II (see below). The promoters for 5S rRNA and tRNA are unique and RNA polymerase III transcribes somewhat bizarre in being internal to the genes. Transcription of these genes requires genes for small non-coding the binding of either of two proteins known as TFIIIA and TFIIIC to a region over RNAs, in particular tRNA and 5S rRNA. 50 bp downstream from the start site (Fig. 6.19). Once these have bound, they enable TFIIIB to bind to the region around the start of transcription. TFIIIB consists of three polypeptides, including TBP, and positions RNA polymerase III correctly at the start site. As the promoters for RNA polymerase I and RNA polymerase III illustrate, recog- nition factor sites may be upstream or downstream from the start of transcription. However, in both cases, a positioning factor (SL1 or TFIIIB, respectively) is required to make sure that the polymerase starts transcribing at the correct place. These posi- tioning factors thus play a similar role to that of the sigma factor in bacteria. 148 CHAPTER SIX Transcription of Genes FIGURE 6.18 RNA Polymerase I Transcribes rRNA Genes The promoter for RNA polymerase I has an upstream control element and a core promoter, the latter rich in GC sequences. The UBF1 protein recognizes and binds to both the upstream control element and the core promoter. Subsequently, SL1 binds to the DNA in association with UBF1. Finally, RNA polymerase I binds and transcription commences. How this binding pattern facilitates transcription of rRNA is not known. FIGURE 6.19 Internal Promoter for RNA Polymerase III The gene for 5S rRNA is transcribed using a promoter located within the gene itself. The recognition sites are downstream of the start site. TFIIIC (or TFIIIA) binds to both sites and this induces TFIIIB to bind to the promoter near the start site. Only after TFIIIB binds can RNA polymerase III bind. Transcription of Protein-Encoding Genes in Eukaryotes RNA polymerase II transcribes RNA polymerase II transcribes most eukaryotic genes that encode proteins. Recogni- genes that code for proteins. tion of the promoter and initiation of transcription by RNA polymerase II requires a number of general transcription factors. In addition, since many protein-encoding genes vary markedly in expression, a variety of specific transcription factors are needed for expression of certain genes under particular circumstances. For example, in a multi- Transcription of Protein-Encoding Genes in Eukaryotes 149 ENHANCER REGION PROMOTER REGION GENE Transcription Transcription factors factors ~ 100 bp ~ 200 bp FIGURE 6.20 Promoter and Enhancer Although one RNA polymerase is used to transcribe most protein encoding genes, specificity is controlled by transcription factors and their recognition sequences. The promoter region is close to the start site and usually binds several transcription factors. In addition, extra transcription factors bind to regions known as enhancers. These may be far upstream of the promoter, as shown, or may be located downstream. Binding of the transcription factors to their recognition sequences influences polymerase activity and gene expression. cellular organism, different cell types produce different types of proteins. Thus, red blood cells produce hemoglobin, whereas white blood cells make antibodies. Further, protein production often varies during development. Fetal hemoglobin is different from the adult version. The assorted transcription factors bind to and recognize specific sequences on the DNA. These DNA sequences are of two major classes, those comprising the promoter Some transcription factors bind itself and a variety of enhancer sequences (Fig. 6.20). The general transcription factors to the promoter region, others for RNA polymerase II (TFII factors) bind to the promoter region. However, although to distant enhancer sequences. some of the specific transcription factors also bind to the promoter region, others bind to the enhancer. In eukaryotes, many protein-encoding genes are interrupted by introns. These are removed at the RNA stage. Consequently, transcription of DNA to give RNA does not yield messenger RNA directly. The RNA that results from transcription is known as the primary transcript and must be processed as described in Chapter 12 to give mRNA. The present discussion will therefore be limited to the transcription of genes by RNA polymerase II to give the primary transcript. Promoters for RNA polymerase II consist of three regions, the initiator box, the TATA box and a variety of upstream elements (see below). The initiator box is a The TATA box is the critical sequence found at the site where transcription starts. The first transcribed base of the sequence that allows RNA mRNA is usually A with a pyrimidine on each side, as in bacteria. The consensus is polymerase II to recognize the weak: YYCAYYYYY (where Y is any pyrimidine). About 25 base pairs upstream from promoter. this is the TATA box, an AT-rich sequence, which is recognized by the same factor TBP (TATA binding protein or TATA box factor) that is needed for binding of RNA poly- merases I and III. TBP is unusual in binding in the minor groove of DNA. (Almost all DNA-binding proteins bind in the major groove). On both sides of the TATA box are GC-rich regions (Fig. 6.21). TBP is found in three different protein complexes, depending on whether RNA polymerase I, II or III is involved. In the present case, TBP forms part of a transcrip- tion factor complex known as TFIID that is needed to recognize promoters specific for RNA polymerase II. Several other TFII complexes are also needed for RNA poly- merase II function. TFIIA and TFIIB bind next. Then at last RNA polymerase II itself enhancer Regulatory sequence outside, and often far away from, the promoter region that binds transcription factors initiator box Sequence at the start of transcription of a eukaryotic gene primary transcript RNA molecule produced by transcription before it has been processed in any way TATA binding protein (TBP) Transcription factor that recognizes the TATA box TATA box Binding site for a transcription factor that guides RNA polymerase II to the promoter in eukaryotes TATA box factor Another name for TATA binding protein upstream element DNA sequence upstream of the TATA box in eukaryotic promoters that is recognized by specific proteins 150 CHAPTER SIX Transcription of Genes Upstream Upstream TATA Initiator Gene DNA element element box box Promoter 0 Start of transcription FIGURE 6.21 Eukaryotic Promoter Components—Initiator and TATA Boxes The promoter for RNA polymerase II has an initiator box at the start site and a TATA box slightly upstream of this. Further upstream there are normally several upstream elements (two are shown here). Startpoint FIGURE 6.22 Binding of RNA Polymerase II to Promoter Starting with TFIID, which contains TATA binding protein, the components of the TFII complex bind one after another. Finally TFIIF helps RNA polymerase II to bind to Start of the DNA. transcription arrives, accompanied by TFIIF which probably helps RNA polymerase bind (Fig. 6.22). At this point RNA polymerase II can initiate synthesis of RNA. However, it is not yet free to move away from the promoter. Release of RNA polymerase II from the promoter and elongation of the RNA requires three more TFII complexes, TFIIE, TFIIH and TFIIJ. In particular, TFIIH must phosphorylate the tail of RNA polymerase before it can move (Fig. 6.23). The tail, or CTD (carboxy-terminal domain), consists of a seven-amino acid sequence (Tyr Ser Pro Thr Ser Pro Ser) repeated approximately 50 times. This may be phosphory- lated on the serine or threonine residues. All of the TFII complexes except for TFIIH are left behind as RNA polymerase moves forward. Like bacterial RNA polymerase, the eukaryotic RNA polymerases all have mul- tiple subunits. RNA polymerase II has more than 10 subunits and shares three of these CTD (carboxy-terminal domain) Repetitive region at the C-terminus of RNA polymerase II that may be phosphorylated Upstream Elements Increase the Efficiency of RNA Polymerase II Binding 151 Startpoint BINDING OF TFIIE TFIIE, TFIIH, TFII J FIGURE 6.23 RNA Polymerase II Moves Forward from the TFIIH PHOSPHORYLATES SPH Promoter THE TAIL OF RNA Pol II Before RNA polymerase II can move forward, the binding of other factors must occur. One of these, TFIIH, phosphorylates the tail of RNA polymerase II. The tail changes position with respect to the body of RNA polymerase II. The other factors leave and RNA polymerase moves along the DNA and begins the process of Phosphate transcription. groups with RNA polymerases I and III. The largest subunit of RNA polymerase II is related to the b¢ subunit of bacterial RNA polymerase and possesses the CTD tail. In addi- tion, the assorted TFII complexes each consist of several polypeptide chains. Thus the initiation complex for RNA polymerase II includes over 20 polypeptides. Upstream Elements Increase the Efficiency of RNA Polymerase II Binding RNA polymerase II can bind and initiate transcription at minimal promoter con- sisting of just an initiator and TATA boxes. However, this is extremely inefficient Upstream elements close to the promoter bind a range of unless upstream elements are also present. There are many different upstream ele- specific transcription factors. ments. They are typically five to ten base pairs long and located from 50 to 200 bases upstream of the start site. There may be more than one upstream element in a given promoter and the same upstream element may be found at different places in differ- ent promoters. The TFII proteins are general transcription factors because they are always required. In contrast, specific transcription factors affect only certain genes and are involved in regulating gene expression in response to a variety of signals (Fig. 6.24). The upstream elements are the recognition sites for specific transcription factors. These usually make contact with the transcription apparatus via TFIID, TFIIB or TFIIA, not by directly touching RNA polymerase II itself. Most commonly, binding is to TFIID. Binding of the specific transcription factors helps assembly of the transcription appa- ratus and therefore increases the frequency of initiation. 152 CHAPTER SIX Transcription of Genes 0 Start site FIGURE 6.24 Upstream Elements Facilitate Transcription The upstream elements make contact with one domain of an activator protein. The activator also binds to the transcription apparatus near the start site. TABLE 6.01 General Transcription Factors Associated with RNA Polymerase II TBP binds to TATA box, part of TFIID TFIID includes TBP, recognizes Pol II specific promoter TFIIA binds upstream of TATA box; required for binding of RNA Pol II to promoter TFIIB binds downstream of TATA box; required for binding of RNA Pol II to promoter TFIIF accompanies RNA Pol II as it binds to promoter TFIIE required for promoter clearance and elongation TFIIH phosphorylates the tail of RNA Pol II, retained by polymerase during elongation TFIIJ required for promoter clearance and elongation Common upstream elements include the GC box, CAAT box, AP1 element and Octamer element. The GC box (GGGCGG) is often present in multiple copies. Despite being nonsymmetrical, the GC box works in either orientation and is recog- nized by the SP1 factor. Some upstream elements are recognized by more than one protein. In these cases, different transcription factors are often present in different tissues. For example, the Oct-1 and Oct-2 proteins both recognize the Octamer In eukaryotes most genes are element. Oct-1 is found in all tissues but Oct-2 only appears in immune cells, where it subject to positive control. helps activate genes encoding antibodies. Repressors are rare and The specific transcription factors that bind to the upstream elements and usually behave differently to enhancers are thus gene activator proteins. Repressors are rare in eukaryotes. those in bacteria. Furthermore, when found, they do not bind to the DNA directly and block the binding of the RNA polymerase. Instead, they bind to some component of the growing transcription apparatus and block further assembly. Enhancers Control Transcription at a Distance Enhancers are sequences that are involved in gene regulation, especially during devel- opment or in different cell types. Enhancers do exactly what their name indicates— they enhance the initiation of transcription as a result of binding specific transcription factors. Enhancers often consist of a cluster of recognition sites and therefore bind Enhancer sequences are several proteins. Some recognition sites (e.g., Octamer and AP1) are found in both located far away from the enhancers and as upstream elements in promoters. genes they control. Although enhancers are sometimes close to the genes they control, more often they are found a considerable distance, perhaps thousands of base pairs away, not even Enhancers Control Transcription at a Distance 153 FIGURE 6.25 Looping Model for Enhancer The enhancer shown here is located downstream of the start site. To enhance transcription, the enhancer first binds several transcription factors. Subsequently, the DNA forms a loop allowing the enhancer to make contact with the transcription apparatus via the bound transcription factors. associated with the gene. Enhancers may be located either upstream or downstream from the promoter and the position may vary from case to case. In addition, enhancers function equally well in either orientation. Experiments in which enhancers have been moved have shown that an enhancer will increase transcription from any promoter within its neighborhood. These properties imply that the enhancer must make contact with the transcription apparatus. When an enhancer switches a gene on, the DNA between it and the promoter loops out as shown in Figure 6.25.