Drug Biotransformation PDF
Document Details
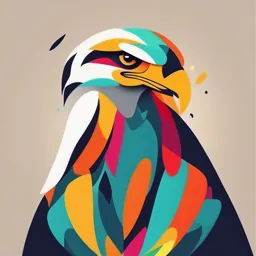
Uploaded by MiraculousMeteor
Creighton University Medical Center
Maria Almira Correia, PhD
Tags
Summary
This document explains drug biotransformation, a crucial process in pharmacology. It details the necessity of this process and its role in drug disposition, using a case study example. The document also discusses the role of different organs like liver and intestines in the process.
Full Transcript
4 C H A P T E R Drug Biotransformation Maria Almira Correia, PhD C ASE STUDY A 40-year-old woman presents to the emergency department...
4 C H A P T E R Drug Biotransformation Maria Almira Correia, PhD C ASE STUDY A 40-year-old woman presents to the emergency department reveal abnormal liver function as indicated by the increased of her local hospital somewhat disoriented, complaining of indices: alkaline phosphatase 302 (41–133),* alanine amino- midsternal chest pain, abdominal pain, shaking, and vomiting transferase (ALT) 351 (7–56),* aspartate aminotransferase for 2 days. She admits to having taken a “handful” of Lorcet (AST) 1045 (0–35),* bilirubin 3.33 mg/dL (0.1–1.2),* and pro- (hydrocodone/acetaminophen, an opioid/nonopioid analgesic thrombin time of 19.8 seconds (11–15).* In addition, plasma combination), Soma (carisoprodol, a centrally acting muscle bicarbonate is reduced, and she has ~45% reduced glomerular relaxant), and Cymbalta (duloxetine HCl, an antidepressant/ filtration rate from the normal value at her age, elevated serum antifibromyalgia agent) 2 days earlier. On physical examina- creatinine and blood urea nitrogen, markedly reduced blood tion, the sclera of her eyes shows yellow discoloration. Labora- glucose of 35 mg/dL, and a plasma acetaminophen concentra- tory analyses of blood drawn within an hour of her admission tion of 75 mcg/mL (10–20).* Her serum titer is significantly positive for hepatitis C virus (HCV). Given these data, how * Normal values are in parentheses. would you proceed with the management of this case? Humans are exposed daily to a wide variety of foreign compounds drugs and other environmental xenobiotics. Renal excretion plays called xenobiotics—substances absorbed across the lungs or skin a pivotal role in terminating the biologic activity of some drugs, or, more commonly, ingested either unintentionally as compounds particularly those that have small molecular volumes or possess present in food and drink or deliberately as drugs for therapeutic polar characteristics, such as functional groups that are fully ion- or “recreational” purposes. Exposure to environmental xenobiotics ized at physiologic pH. However, many drugs do not possess such may be inadvertent and accidental or—when they are present as physicochemical properties. Pharmacologically active organic components of air, water, and food—inescapable. Some xenobiot- molecules tend to be lipophilic and remain unionized or only ics are innocuous, but many can provoke biologic responses. Such partially ionized at physiologic pH; these are readily reabsorbed biologic responses often depend on conversion of the absorbed from the glomerular filtrate in the nephron. Certain lipophilic substance into an active metabolite. The discussion that follows is compounds are often strongly bound to plasma proteins and may applicable to xenobiotics in general (including drugs) and to some not be readily filtered at the glomerulus. Consequently, most extent to endogenous compounds. drugs would have a prolonged duration of action if termination of their action depended solely on renal excretion. WHY IS DRUG BIOTRANSFORMATION An alternative process that can lead to the termination or NECESSARY? alteration of biologic activity is metabolism. In general, lipophilic xenobiotics are transformed to more polar and hence more readily The mammalian drug biotransformation systems are thought to excreted products. The role that metabolism plays in the inactiva- have first evolved from the need to detoxify and eliminate plant tion of lipid-soluble drugs can be quite dramatic. For example, and bacterial bioproducts and toxins, which later extended to lipophilic barbiturates such as thiopental and pentobarbital would 56 CHAPTER 4 Drug Biotransformation 57 have extremely long half-lives if it were not for their metabolic O H conversion to more water-soluble compounds. N C N NH2 (INH) Metabolic products are often less pharmacodynamically active than the parent drug and may even be inactive. However, some bio- Phase II (acetylation) transformation products have enhanced activity or toxic properties. It is noteworthy that the synthesis of endogenous substrates such as O H H O steroid hormones, cholesterol, active vitamin D congeners, and bile N C N N C CH3 (N-acetyl INH) acids involves many pathways catalyzed by enzymes associated with the metabolism of xenobiotics. Finally, drug-metabolizing enzymes Phase I (hydrolysis) have been exploited in the design of pharmacologically inactive prodrugs that are converted to active molecules in the body. O O H N C OH + CH3 C N NH2 (acetylhydrazine) THE ROLE OF BIOTRANSFORMATION IN Acetylation of DRUG DISPOSITION Isonicotinic acid macromolecules (proteins) Most metabolic biotransformations occur at some point between Hepatotoxicity absorption of the drug into the circulation and its renal elimination. A few transformations occur in the intestinal lumen or intestinal FIGURE 4–2 Phase II activation of isoniazid (INH) to a hepatotoxic wall. In general, all of these reactions can be assigned to one of two metabolite. major categories called phase I and phase II reactions (Figure 4–1). Phase I reactions usually convert the parent drug to a more N-acetyl conjugate in a phase II reaction. This conjugate is then polar metabolite by introducing or unmasking a functional group a substrate for a phase I type reaction, namely, hydrolysis to (–OH, –NH2, –SH). Often these metabolites are inactive, although isonicotinic acid (Figure 4–2). Thus, phase II reactions may actually in some instances activity is only modified or even enhanced. precede phase I reactions. If phase I metabolites are sufficiently polar, they may be readily excreted. However, many phase I products are not eliminated rap- idly and undergo a subsequent reaction in which an endogenous WHERE DO DRUG substrate such as glucuronic acid, sulfuric acid, acetic acid, or an BIOTRANSFORMATIONS OCCUR? amino acid combines with the newly incorporated functional group to form a highly polar conjugate. Such conjugation or Although every tissue has some ability to metabolize drugs, the synthetic reactions are the hallmarks of phase II metabolism. A liver is the principal organ of drug metabolism. Other tissues that great variety of drugs undergo these sequential biotransformation display considerable activity include the gastrointestinal tract, the reactions, although in some instances, the parent drug may already lungs, the skin, the kidneys, and the brain. After oral administration, possess a functional group that may form a conjugate directly. For many drugs (eg, isoproterenol, meperidine, pentazocine, morphine) example, the hydrazide moiety of isoniazid is known to form an are absorbed intact from the small intestine and transported first Absorption Metabolism Elimination Phase I Phase II Drug Conjugate Drug metabolite with modified activity Conjugate Drug Inactive drug metabolite Conjugate Drug Lipophilic Hydrophilic FIGURE 4–1 Phase I and phase II reactions, and direct elimination, in drug biodisposition. Phase II reactions may also precede phase I reactions. 58 SECTION I Basic Principles via the portal system to the liver, where they undergo exten- and fractionation of the cell, they re-form into vesicles called sive metabolism. This process is called the first-pass effect (see microsomes. Microsomes retain most of the morphologic and Chapter 3). Some orally administered drugs (eg, clonazepam, functional characteristics of the intact membranes, including chlorpromazine, cyclosporine) are more extensively metabolized the rough and smooth surface features of the rough (ribosome- in the intestine than in the liver, while others (eg, midazolam) studded) and smooth (no ribosomes) endoplasmic reticulum. undergo significant (~50%) intestinal metabolism. Thus, intesti- Whereas the rough microsomes tend to be dedicated to protein nal metabolism can contribute to the overall first-pass effect, and synthesis, the smooth microsomes are relatively rich in enzymes individuals with compromised liver function may rely increasingly responsible for oxidative drug metabolism. In particular, they on such intestinal metabolism for drug elimination. Compromise contain the important class of enzymes known as the mixed func- of intestinal metabolism of certain drugs (eg, felodipine, cyclo- tion oxidases (MFOs), or monooxygenases. The activity of these sporine A) can also result in significant elevation of their plasma enzymes requires both a reducing agent (nicotinamide adenine levels and clinically relevant drug-drug interactions (DDIs, see dinucleotide phosphate [NADPH]) and molecular oxygen; in a below). First-pass effects may limit the bioavailability of orally typical reaction, one molecule of oxygen is consumed (reduced) administered drugs (eg, lidocaine) so greatly that alternative routes per substrate molecule, with one oxygen atom appearing in the of administration must be used to achieve therapeutically effective product and the other in the form of water. blood levels. Furthermore, the lower gut harbors intestinal micro- In this oxidation-reduction process, two microsomal enzymes organisms that are capable of many biotransformation reactions. play a key role. The first of these is a flavoprotein, NADPH- In addition, drugs may be metabolized by gastric acid (eg, peni- cytochrome P450 oxidoreductase (POR, or CPR). One mole cillin), by digestive enzymes (eg, polypeptides such as insulin), of this enzyme contains 1 mol each of flavin mononucleotide or by enzymes in the wall of the intestine (eg, sympathomimetic (FMN) and flavin adenine dinucleotide (FAD). The second catecholamines). microsomal enzyme is a hemoprotein called cytochrome P450, Although drug biotransformation in vivo can occur by spon- which serves as the terminal oxidase. In fact, the microsomal taneous, noncatalyzed chemical reactions, most transformations membrane harbors multiple forms of this hemoprotein, and are catalyzed by specific cellular enzymes. At the subcellular level, this multiplicity is increased by repeated administration of or these enzymes may be located in the endoplasmic reticulum, exposure to exogenous chemicals (see text that follows). The mitochondria, cytosol, lysosomes, or even the nuclear envelope or name cytochrome P450 (abbreviated as P450 or CYP) is derived plasma membrane. from the spectral properties of this hemoprotein. In its reduced (ferrous) form, it binds carbon monoxide to give a complex that absorbs light maximally at 450 nm. The relative abundance MICROSOMAL MIXED FUNCTION of P450s, compared with that of the reductase in the liver, OXIDASE SYSTEM & PHASE I REACTIONS contributes to making P450 heme reduction a rate-limiting step in hepatic drug oxidations. Many drug-metabolizing enzymes are located in the lipophilic Microsomal drug oxidations require P450, P450 reductase, endoplasmic reticulum membranes of the liver and other tissues. NADPH, and molecular oxygen. A simplified scheme of the +3 When these lamellar membranes are isolated by homogenization oxidative cycle is presented in Figure 4–3. Briefly, oxidized (Fe ) Drug RH Flavoprotein NADP+ (reduced) 1 P450[Fe+3] P450[Fe+3] ROH P450 Reductase RH 2 Flavoprotein e− NADPH (oxidized) CO P450 P450[Fe+3] CO P450[Fe+2] P450[Fe+2] ROH RH RH O2 e− H2O O2 3 4 P450[Fe+2] 2H+ RH FIGURE 4–3 Cytochrome P450 cycle in drug oxidations. e–, electron; RH, parent drug; ROH, oxidized metabolite. CHAPTER 4 Drug Biotransformation 59 P450 combines with a drug substrate to form a binary complex smoke, charcoal-broiled meat, and other organic pyrolysis prod- (step 1). NADPH donates an electron to the flavoprotein P450 ucts, is known to induce CYP1A enzymes and to alter the rates reductase, which in turn reduces the oxidized P450-drug complex of drug metabolism. Other environmental chemicals known (step 2). A second electron is introduced from NADPH via the to induce specific P450s include the polychlorinated biphenyls same P450 reductase, which serves to reduce molecular oxygen (PCBs), which were once used widely in industry as insulating and to form an “activated oxygen”–P450–substrate complex materials and plasticizers, and 2,3,7,8-tetrachlorodibenzo-p- (step 3). This complex in turn transfers activated oxygen to the dioxin (dioxin, TCDD), a trace byproduct of the chemical synthesis drug substrate to form the oxidized product (step 4). of the defoliant 2,4,5-T (see Chapter 56). The potent oxidizing properties of this activated oxygen permit Increased P450 synthesis requires enhanced transcription and oxidation of a large number of substrates. Substrate specificity is translation along with increased synthesis of heme, its prosthetic very low for this enzyme complex. High lipid solubility is the cofactor. A cytoplasmic receptor (termed AhR) for polycyclic only common structural feature of the wide variety of structurally aromatic hydrocarbons (eg, benzo[a]pyrene, dioxin) has been unrelated drugs and chemicals that serve as substrates in this sys- identified. The translocation of the inducer-receptor complex into tem (Table 4–1). However, compared with many other enzymes the nucleus, followed by ligand-induced dimerization with Arnt, including phase II enzymes, P450s are remarkably sluggish a closely related nuclear protein, leads to subsequent activation of catalysts, and their drug biotransformation reactions are slow. regulatory elements of CYP1A genes, resulting in their induction. This is also the mechanism of CYP1A induction by crucifer- ous vegetables, and the proton pump inhibitor, omeprazole. A HUMAN LIVER P450 ENZYMES pregnane X receptor (PXR), a member of the steroid-retinoid- thyroid hormone receptor family, has recently been shown to Gene arrays combined with immunoblotting analyses of micro- mediate CYP3A induction by various chemicals (dexamethasone, somal preparations, as well as the use of relatively selective rifampin, mifepristone, phenobarbital, atorvastatin, and hyper- functional markers and selective P450 inhibitors, have identified forin, a constituent of St. John’s wort) in the liver and intestinal numerous P450 isoforms (CYP: 1A2, 2A6, 2B6, 2C8, 2C9, 2C18, mucosa. A similar receptor, the constitutive androstane receptor 2C19, 2D6, 2E1, 3A4, 3A5, 4A11, and 7) in the human liver. (CAR), has been identified for the relatively large and structur- Of these, CYP1A2, CYP2A6, CYP2B6, CYP2C9, CYP2D6, ally diverse phenobarbital class of inducers of CYP2B6, CYP2C9, CYP2E1, and CYP3A4 appear to be the most important forms, and CYP3A4. Peroxisome proliferator receptor α (PPAR-α) is yet accounting for approximately 15%, 4%, 1%, 20%, 5%, 10%, and another nuclear receptor highly expressed in liver and kidneys, 30%, respectively, of the total human liver P450 content. Together, which uses lipid-lowering drugs (eg, fenofibrate and gemfibrozil) they are responsible for catalyzing the bulk of the hepatic drug and as ligands. Consistent with its major role in the regulation of fatty xenobiotic metabolism (Table 4–2, Figure 4–4). acid metabolism, PPAR-α mediates the induction of CYP4A It is noteworthy that CYP3A4 alone is responsible for the enzymes, responsible for the metabolism of fatty acids such as metabolism of over 50% of the prescription drugs metabolized by arachidonic acid and its physiologically relevant derivatives. It is the liver. The involvement of individual P450s in the metabolism noteworthy that on binding of its particular ligand, PXR, CAR, of a given drug may be screened in vitro by means of selective and PPAR-α each forms heterodimers with another nuclear functional markers, selective chemical P450 inhibitors, and P450 receptor, the retinoid X-receptor (RXR). This heterodimer in turn antibodies. In vivo, such screening may be accomplished by means binds to response elements within the promoter regions of specific of relatively selective noninvasive markers, which include breath P450 genes to induce gene expression. tests or urinary analyses of specific metabolites after administra- P450 enzymes may also be induced by substrate stabilization, tion of a P450-selective substrate probe. eg, decreased degradation, as is the case with troleandomycin- or clotrimazole-mediated induction of CYP3A enzymes, the ethanol- Enzyme Induction mediated induction of CYP2E1, and the isosafrole-mediated Some of the chemically dissimilar P450 substrate drugs, on induction of CYP1A2. repeated administration, induce P450 expression by enhancing the rate of its synthesis or reducing its rate of degradation (Table 4–2). Enzyme Inhibition Induction results in accelerated substrate metabolism and usually Certain drug substrates inhibit cytochrome P450 enzyme activity in a decrease in the pharmacologic action of the inducer and also (Table 4–2). Imidazole-containing drugs such as cimetidine and of co-administered drugs. However, in the case of drugs metaboli- ketoconazole bind tightly to the P450 heme iron and effectively cally transformed to reactive metabolites, enzyme induction may reduce the metabolism of endogenous substrates (eg, testosterone) exacerbate metabolite-mediated toxicity. or other co-administered drugs through competitive inhibition. Various substrates induce P450 isoforms having different Macrolide antibiotics such as troleandomycin, erythromycin, and molecular masses and exhibiting different substrate specificities erythromycin derivatives are metabolized, apparently by CYP3A, and immunochemical and spectral characteristics. to metabolites that complex the cytochrome P450 heme iron Environmental chemicals and pollutants are also capable of and render it catalytically inactive. Another compound that acts inducing P450 enzymes. Exposure to benzo[a]pyrene and other through this mechanism is the inhibitor proadifen (SKF-525-A, polycyclic aromatic hydrocarbons, which are present in tobacco used in research), which binds tightly to the heme iron and 60 SECTION I Basic Principles TABLE 4–1 Phase I reactions. Reaction Class Structural Change Drug Substrates Oxidations Cytochrome P450-dependent oxidations: Aromatic hydroxylations R R Acetanilide, propranolol, phenobarbital, pheny- toin, phenylbutazone, amphetamine, warfarin, 17α-ethinyl estradiol, naphthalene, benzpyrene R OH O Aliphatic hydroxylations RCH2CH3 RCH2CH2OH Amobarbital, pentobarbital, secobarbital, RCH2CH3 RCHCH3 chlorpropamide, ibuprofen, meprobamate, glutethimide, phenylbutazone, digitoxin OH Epoxidation H O H Aldrin RCH CHR R C C R Oxidative dealkylation N-Dealkylation RNHCH3 RNH2 + CH2O Morphine, ethylmorphine, benzphetamine, aminopyrine, caffeine, theophylline O-Dealkylation ROCH3 ROH + CH2O Codeine, p-nitroanisole S-Dealkylation RSCH3 RSH + CH2O 6-Methylthiopurine, methitural N-Oxidation Primary amines RNH2 RNHOH Aniline, chlorphentermine Secondary amines R1 R1 2-Acetylaminofluorene, acetaminophen NH N OH R2 R2 Tertiary amines R1 R1 Nicotine, methaqualone R2 N R2 N→O R3 R3 S-Oxidation R1 R1 Thioridazine, cimetidine, chlorpromazine S S O R2 R2 Deamination OH Amphetamine, diazepam RCHCH3 R C CH3 R CCH3 + NH3 NH2 NH2 O Desulfuration R1 R1 Thiopental C S C O R2 R2 (continued ) CHAPTER 4 Drug Biotransformation 61 TABLE 4–1 Phase I reactions. (Continued) Reaction Class Structural Change Drug Substrates Cytochrome P450- dependent oxidations: (continued) R1 R1 Parathion P S P O R2 R2 Dechlorination CCl4 [CCl3 ] CHCl3 Carbon tetrachloride Cytochrome P450-independent oxidations: Flavin monooxygenase + Chlorpromazine, amitriptyline, H (Ziegler’s enzyme) R3N R3N+→ O– R3N+OH benzphetamine RCH2N CH2R RCH2 N CH2R Desipramine, nortriptyline H OH RCH N CH2R O– N N N Methimazole, propylthiouracil SH SOH SO2H N N N Amine oxidases RCH2NH2 RCHO + NH3 Phenylethylamine, epinephrine Dehydrogenations RCH2OH RCHO Ethanol Reductions Azo reductions Prontosil, tartrazine RN NR1 RNH NHR1 RNH2 + R1NH2 Nitro reductions RNO2 RNO RNHOH RNH2 Nitrobenzene, chloramphenicol, clonazepam, dantrolene Carbonyl reductions RCR RCHR Metyrapone, methadone, naloxone O OH Hydrolyses Esters R1COOR2 R1COOH + R2OH Procaine, succinylcholine, aspirin, clofibrate, methylphenidate Amides RCONHR1 RCOOH + R1NH2 Procainamide, lidocaine, indomethacin quasi-irreversibly inactivates the enzyme, thereby inhibiting the steroids (ethinyl estradiol, norethindrone, and spironolactone); metabolism of potential substrates. fluroxene; allobarbital; the analgesic sedatives allylisopropylacetylurea, Some substrates irreversibly inhibit P450s via covalent interac- diethylpentenamide, and ethchlorvynol; carbon disulfide; grape- tion of a metabolically generated reactive intermediate that may fruit furanocoumarins; selegiline; phencyclidine; ticlopidine and react with the P450 apoprotein or heme moiety or even cause the clopidogrel; ritonavir; and propylthiouracil. On the other hand, heme to fragment and irreversibly modify the apoprotein. The the barbiturate secobarbital is found to inactivate CYP2B1 by antibiotic chloramphenicol is metabolized by CYP2B1 to a spe- modification of both its heme and protein moieties. Other meta- cies that modifies the P450 protein and thus also inactivates bolically activated drugs whose P450 inactivation mechanism is the enzyme. A growing list of such suicide inhibitors—inactivators not fully elucidated are mifepristone, troglitazone, raloxifene, and that attack the heme or the protein moiety—includes certain tamoxifen. 62 SECTION I Basic Principles TABLE 4–2 Human liver P450s (CYPs), and some of the drugs metabolized (substrates), inducers, and selective inhibitors. Note: Some P450 substrates can be potent competitive inhibitors and/or mechanism-based inactivators. CYP Substrates Inducers Inhibitors 1A2 Acetaminophen, alosetron, antipyrine, caffeine, clomip- Charcoal-broiled foods, Artemisinin, atazanavir, cimetidine, ramine, clozapine, duloxetine, flutamide, frovatriptan, cruciferous vegetables, ciprofloxacin, enoxacin, ethinyl estradiol, melatonin, mexiletine, mirtazapine, olanzapine, phenacetin, grilled meat, lansoprazole, fluvoxamine, furafylline, galangin, ramelteon, rasagiline, ropinirole, tacrine, tamoxifen, theoph- omeprazole, primidone, mexiletene, tacrine, thiabendazole, ylline, tizanidine, triamterene, warfarin, zolmitriptan rifampin, smoking zileuton 2A6 Coumarin, dexmedetomidine, tobacco nitrosamines, nicotine Efavirenz, rifampin, Clotrimazole, isoniazid, ketoconazole, (to cotinine and 2′-hydroxynicotine) phenobarbital letrozole, menthofuran, methimazole, methoxsalen, miconazole, tranylcypromine 2B6 Artemisinin, bupropion, clopidogrel, cyclophosphamide, Carbamazepine, cyclophos- Amiodarone, amlodipine, clopidogrel, efavirenz, ifosfamide, irinotecan, ketamine, S-mephobarbital, phamide, fosphenytoin, clotrimazole, desipramine, disulfiram, S-mephenytoin (N-demethylation to nirvanol), methadone, nevirapine, phenobarbital, doxorubicin, ethinyl estradiol, fluoxetine, nevirapine, promethazine, propofol, selegiline, sertraline, primidone, rifampin fluvoxamine, isoflurane, ketoconazole, ticlopidine mestranol, methimazole, nefazodone, nelfinavir, orphenadrine, paroxetine, phencyclidine, sertraline, thiotepa, ticlopidine 2C8 Amiodarone, cabazitaxel, carbamazepine, chloroquine, Rifampin, barbiturates Deferasirox, gemfibrozil, lapatinib, diclofenac, ibuprofen, paclitaxel, all-trans-retinoic acid, montelukast, pioglitazone, quercetin, repaglinide, rosiglitazone, treprostinil rosiglitazone, trimethoprim 2C9 Alosetron, bosentan, celecoxib, chlorpropamide, diclofenac, Aminoglutethimide, Amiodarone, clopidogrel, delavirdine, dronabinol, flurbiprofen, fluvastatin, glimepiride, glipizide, barbiturates, bosentan, disulfiram, doxifluridine, efavirenz, glyburide, hexobarbital, ibuprofen, indomethacin, irbesartan, carbamazepine, phenytoin, fluconazole, fluvoxamine, fluorouracil, losartan, meloxicam, montelukast, naproxen, nateglinide, primidone, rifabutin, imatinib, leflunomide, metronidazole, phenobarbital, phenytoin, piroxicam, rosiglitazone, rifampin, rifapentine, miconazole, phenytoin, sulfamethoxa- rosuvastatin, sulfamethoxazole, sulfaphenazole, ticrynafen, St. John’s wort zole, sulfaphenazole, sulfinpyrazone, tolbutamide, torsemide, trimethadione, valsartan, S-warfarin tienilic acid, valproic acid, voriconazole 2C18 Tolbutamide, phenytoin Phenobarbital 2C19 Aripiprazole, carisoprodol, citalopram, clomipramine, clopi- Aminoglutethimide, N3-Benzylnirvanol, N3-benzylpheno- dogrel, clozapine, desipramine, diazepam, diphenhydramine, artemisinin, barbiturates, barbital, chloramphenicol, cimetidine, doxepin, escitalopram, fluoxetine, imipramine, lansoprazole, carbamazepine, phenytoin, clopidogrel, delavirdine, efavirenz, S-mephenytoin, methadone, moclobemide, naproxen, primidone, rifampin, esomeprazole, felbamate, fluconazole, nelfinavir, nirvanol, olanzapine, omeprazole, pantoprazole, rifapentine, St. John’s wort fluoxetine, fluvoxamine, isoniazid, phenobarbital, phenytoin, proguanil, propranolol, rabeprazole, moclobemide, modafinil, nootkatone, sertraline, thalidomide, voriconazole, R-warfarin omeprazole, ticlopidine, voriconazole 2D6 Amitriptyline, atomoxetine, bufuralol, bupranolol, carvedilol, Unknown Bupropion, cinacalcet, chloroquine, chlorpheniramine, chlorpromazine, clomipramine, clozapine, diphenhydramine, fluoxetine, codeine, debrisoquine, desipramine, dextromethorphan, haloperidol, imatinib, paroxetine, dihydrocodeine, encainide, flecainide, fluoxetine, fluvox- propafenone, propoxyphene, quinidine, amine, guanoxan, haloperidol, hydrocodone, imipramine, terbinafine, thioridazine maprotiline, 4–methoxy-amphetamine, metoclopramide, metoprolol, mexiletine, nebivolol, nortriptyline, oxycodone, palonosetron, paroxetine, perhexiline, perphenazine, phen- formin, propafenone, propoxyphene, propranolol, risperi- done, selegiline (deprenyl), sparteine, tamoxifen, thioridazine, timolol, tolterodine, tricyclic antidepressants, tramadol, trazodone, venlafaxine 2E1 Acetaminophen, chlorzoxazone, dacarbazine, enflurane, Ethanol, isoniazid Amitriptyline, chlorpromazine, cimetidine, ethanol (a minor pathway), halothane, isoflurane, isoniazid, clomethiazole, clotrimazole, clozapine, sevoflurane, theophylline, trimethadione disulfiram, diethylthiocarbamate, diallyl sulfide, econazole, methimazole, methoxsalen, 4-methylpyrazole, miconazole, modafinil, ritonavir, selegiline, sildenafil, sulconazole, ticlopidine, tioconazole (continued) CHAPTER 4 Drug Biotransformation 63 TABLE 4–2 Human liver P450s (CYPs), and some of the drugs metabolized (substrates), inducers, and selective inhibitors. Note: Some P450 substrates can be potent competitive inhibitors and/or mechanism-based inactivators. (Continued) CYP Substrates Inducers Inhibitors 1 3A4 Acetaminophen, alfentanil, alfuzosin, almotriptan, alprazolam, Aminoglutethimide, Amprenavir, azamulin, boceprevir, amiodarone, amlodipine, aprepitant, astemizole, atazanavir, avasimibe, barbiturates, clarithromycin, conivaptan, diltiazem, atorvastatin, bepridil, bexarotene, bosentan, bromocriptine, carbamazepine, efavirenz, erythromycin, fluconazole, grapefruit budesonide, buspirone, carbamazepine, cisapride, clarithro- glucocorticoids, nevirapine, juice (furanocoumarins), indinavir, mycin, clonazepam, clopidogrel, cocaine, colchicine, conivap- pioglitazone, phenytoin, itraconazole, ketoconazole, lopinavir, tan, cortisol, cyclosporine, dapsone, darunavir, dasatinib, primidone, rifampin, rifa- mibefradil, nefazodone, nelfinavir, delavirdine, dexamethasone, diazepam, dihydroergotamine, pentine, St. John’s wort posaconazole, ritonavir, saquinavir, dihydropyridines, diltiazem, disopyramide, doxorubicin, telaprevir, telithromycin, troleandomycin, droperidol, dutasteride, ebastine, efavirenz, eletriptan, eplere- verapamil, voriconazole none, ergotamine, erlotinib, erythromycin, estazolam, eszopi- clone, ethinyl estradiol, ethosuximide, etoposide, everolimus, exemestane, felodipine, fentanyl, finasteride, flurazepam, fluticasone, fosamprenavir, galantamine, gefitinib, gestodene, granisetron, halofantrine, ifosfamide, imatinib, indinavir, irinotecan, isradipine, itraconazole, ixabepilone, lapatinib, lidocaine, loperamide, lopinavir, loratadine, lovastatin, mac- rolides, maraviroc, mefloquine, methadone, methylpred- nisolone, miconazole, midazolam, mifepristone, modafinil, nefazodone, nevirapine, nicardipine, nifedipine, nimodipine, nisoldipine, paclitaxel, paricalcitol, pimozide, pioglitazone, praziquantel, prednisolone, prednisone, progesterone, que- tiapine, quinacrine, quinidine, quinine, ranolazine, rapamycin, repaglinide, rifabutin, ritonavir, saquinavir, sibutramine, sildenafil, simvastatin, sirolimus, solifenacin, spironolactone, sufentanil, sulfamethoxazole, sunitinib, tacrolimus, tadalafil, tamoxifen, tamsulosin, teniposide, terfenadine, testoster- one, tetrahydrocannabinol, tiagabine, tinidazole, tipranavir, tolvaptan, topiramate, triazolam, troleandomycin, vardenafil, verapamil, vinblastine, vincristine, ziprasidone, zolpidem, zonisamide, zopiclone 1 CYP3A5 has similar substrate and inhibitor profiles but, except for a few drugs, is generally less active than CYP3A4. PHASE II REACTIONS (NATs), encoded by NAT1 and NAT2 genes, which utilize acetyl- CoA as the endogenous cofactor. Parent drugs or their phase I metabolites that contain suitable S-Adenosyl-l-methionine (SAMe; AdoMet)-mediated O-, N-, chemical groups often undergo coupling or conjugation reac- and S-methylation of drugs and xenobiotics by methyltransfer- tions with an endogenous substance to yield drug conjugates ases (MTs) also occurs. Finally, endobiotic, drug, and xenobiotic (Table 4–3). In general, conjugates are polar molecules that are epoxides generated via P450-catalyzed oxidations can also be readily excreted and often inactive. Conjugate formation involves hydrolyzed by microsomal or cytosolic epoxide hydrolases (EHs). high-energy intermediates and specific transfer enzymes. Such Conjugation of an activated drug such as the S-CoA derivative of enzymes (transferases) may be located in microsomes or in benzoic acid, with an endogenous substrate, such as glycine, also the cytosol. Of these, uridine 5′-diphosphate (UDP)-glucuronosyl occurs. Because the endogenous substrates originate in the diet, transferases (UGTs) are the most dominant enzymes (Figure 4–4). nutrition plays a critical role in the regulation of drug conjugations. These microsomal enzymes catalyze the coupling of an activated Phase II reactions are relatively faster than P450-catalyzed endogenous substance (such as the UDP derivative of glucuronic reactions, thus effectively accelerating drug biotransformation. acid) with a drug (or endogenous compound such as bilirubin, the Drug conjugations were once believed to represent terminal end product of heme metabolism). Nineteen UGT genes (UGTA1 inactivation events and as such have been viewed as “true detoxifi- and UGT2) encode UGT proteins involved in the metabolism cation” reactions. However, this concept must be modified, because of drugs and xenobiotics. Similarly, 11 human sulfotransferases it is now known that certain conjugation reactions (acyl glucuroni- (SULTs) catalyze the sulfation of substrates using 3′-phospho- dation of nonsteroidal anti-inflammatory drugs, O-sulfation of adenosine 5′-phosphosulfate (PAPS) as the endogenous sulfate N-hydroxyacetylaminofluorene, and N-acetylation of isoniazid) donor. Cytosolic and microsomal glutathione (GSH) transferases may lead to the formation of reactive species responsible for the (GSTs) are also engaged in the metabolism of drugs and xenobiot- toxicity of the drugs. Furthermore, sulfation is known to activate ics, and in that of leukotrienes and prostaglandins, respectively. the orally active prodrug minoxidil into a very efficacious vasodila- Chemicals containing an aromatic amine or a hydrazine moiety tor, and morphine-6-glucuronide is more potent than morphine (eg, isoniazid) are substrates of cytosolic N-acetyltransferases itself. 64 SECTION I Basic Principles A CYP1A1/2 Esterases Epoxide METABOLISM OF DRUGS TO TOXIC CYP1B1 Others hydrolase PRODUCTS CYP2A6 DPYD CYP2B6 Metabolism of drugs and other foreign chemicals may not always CYP2C8/9 be an innocuous biochemical event leading to detoxification and CYP2C19 elimination of the compound. Indeed, as previously noted, several compounds have been shown to be metabolically transformed to reactive intermediates that are toxic to various organs. Such CYP2D6 CYP3A4/5 toxic reactions may not be apparent at low levels of exposure to CYP2E1 parent compounds when alternative detoxification mechanisms are not yet overwhelmed or compromised and when the avail- TPMT NATs B GSTs ability of endogenous detoxifying cosubstrates (GSH, glucuronic Others acid, sulfate) is not limited. However, when these resources are exhausted, the toxic pathway may prevail, resulting in overt organ toxicity or carcinogenesis. The number of specific examples of such drug-induced toxicity is expanding rapidly. An example is acetaminophen (APAP; paracetamol)-induced hepatotoxicity (Figure 4–5). Acetaminophen, an analgesic antipyretic drug, is SULTs UGTs quite safe in therapeutic doses (1.2 g/d for an adult). It normally undergoes glucuronidation and sulfation to the corresponding FIGURE 4–4 Relative contributions of various cytochrome P450 conjugates, which together make up 95% of the total excreted isoforms (A) and different phase II pathways (B) to metabolism of metabolites. The alternative P450-dependent GSH conjugation drugs in clinical use. Many drugs are metabolized by two or more pathway accounts for the remaining 5%. When acetaminophen of these pathways. Note that two pathways, CYP3A4/5 and UGT, are intake far exceeds therapeutic doses, the glucuronidation and involved in the metabolism of more than 75% of drugs in use. DPYD, sulfation pathways are saturated, and the P450-dependent path- dihydropyrimidine dehydrogenase; GST, glutathione-S-transferase; NAT, N-acetyltransferase; SULT, sulfotransferase; TPMT, thiopurine way becomes increasingly important. Little or no hepatotoxic- methyltransferase; UGT, UDP-glucuronosyltransferase. (Reproduced, ity results as long as hepatic GSH is available for conjugation. with permission, from Brunton LL, Chabner BA, Knollman BC: Goodman & Gilman’s However, with time, hepatic GSH is depleted faster than it can The Pharmacological Basis of Therapeutics, 12th ed. McGraw-Hill, 2011. Copyright © be regenerated, and a reactive, toxic metabolite accumulates. The McGraw-Hill Companies, Inc.) In the absence of intracellular nucleophiles such as GSH, this TABLE 4–3 Phase II reactions. Type of Endogenous Transferase Conjugation Reactant (Location) Types of Substrates Examples Glucuronidation UDP glucuronic acid UDP glucuronosyl- Phenols, alcohols, carboxylic Nitrophenol, morphine, acetaminophen, (UDPGA) transferase acids, hydroxylamines, diazepam, N-hydroxydapsone, sulfathia- (microsomes) sulfonamides zole, meprobamate, digitoxin, digoxin Acetylation Acetyl-CoA N-Acetyltransferase Amines Sulfonamides, isoniazid, clonazepam, (cytosol) dapsone, mescaline Glutathione Glutathione (GSH) GSH-S-transferase Epoxides, arene oxides, nitro Acetaminophen, ethacrynic acid, conjugation (cytosol, microsomes) groups, hydroxylamines bromobenzene Glycine Glycine Acyl-CoA glycinetrans Acyl-CoA derivatives of Salicylic acid, benzoic acid, nicotinic conjugation ferase (mitochondria) carboxylic acids acid, cinnamic acid, cholic acid, deoxycholic acid Sulfation Phosphoadenosyl Sulfotransferase Phenols, alcohols, aromatic Estrone, aniline, phenol, phosphosulfate (cytosol) amines 3- hydroxycoumarin, acetaminophen, (PAPS) methyldopa Methylation S-Adenosylmethionine Transmethylases Catecholamines, phenols, Dopamine, epinephrine, pyridine, (SAM) (cytosol) amines histamine, thiouracil Water Water Epoxide hydrolase Arene oxides, cis-disubsti- Benzopyrene 7,8-epoxide, conjugation (microsomes) tuted and monosubstituted styrene 1,2-oxide, carbamazepine oxiranes epoxide (cytosol) Alkene oxides, fatty acid Leukotriene A4 epoxides CHAPTER 4 Drug Biotransformation 65 Acetaminophen CLINICAL RELEVANCE OF DRUG NHCOCH3 METABOLISM COOH The dose and frequency of administration required to achieve O Glucuronidation Sulfation effective therapeutic blood and tissue levels vary in different O OH UDP OH patients because of individual differences in drug distribution and O ADP SO3H CYP2E1 CYP3A4 rates of drug metabolism and elimination. These differences are OH determined by genetic factors as well as nongenetic variables, such OH HONCOCH3 as commensal gut microbiota, age, sex, liver size, liver function, NHCOCH3 NHCOCH3 circadian rhythm, body temperature, and nutritional and environ- mental factors such as concomitant exposure to inducers or inhibi- tors of drug metabolism. The discussion that follows summarizes COOH OH the most important of these variables. O OH O O SO3H Reactive Toxic OH Intermediates NCOCH3 Nontoxic sulfate Individual Differences OH Individual differences in metabolic rate depend on the nature of the Nontoxic + drug itself. Thus, within the same population, steady-state plasma glucuronide levels may reflect a 30-fold variation in the metabolism of one drug O and only a twofold variation in the metabolism of another. GSH-Conjugation + Nucleophillic +GSH Cell Macromolecules (Protein-SH) NHCOCH3 NHCOCH3 Genetic Factors Genetic factors that influence enzyme levels account for some of these differences, giving rise to “genetic polymorphisms” in drug SG S-Protein metabolism (see also Chapter 5). The first examples of drugs found OH OH to be subject to genetic polymorphisms were the muscle relaxant succinylcholine, the antituberculosis drug isoniazid, and the anti- coagulant warfarin. A true genetic polymorphism is defined as the NHCOCH3 occurrence of a variant allele of a gene at a population frequency of LIVER CELL DEATH ≥ 1%, resulting in altered expression or functional activity of the gene product, or both. Well-defined and clinically relevant genetic SCH2CHNHCOCH3 polymorphisms in both phase I and phase II drug-metabolizing OH COOH enzymes exist that result in altered efficacy of drug therapy or Mercapturic Acid adverse drug reactions (ADRs). The latter frequently necessitate Conjugate dose adjustment (Table 4–4), a consideration particularly crucial for drugs with low therapeutic indices. FIGURE 4–5 Metabolism of acetaminophen (top center) to hepatotoxic metabolites. GSH, glutathione; SG, glutathione moiety. A. Phase I Enzyme Polymorphisms Genetically determined defects in the phase I oxidative metabolism of several drugs have been reported (Table 4–4; see also Chapter 5). reactive metabolite (N-acetylbenzoiminoquinone) not only reacts These defects are often transmitted as autosomal recessive traits and with nucleophilic groups of cellular proteins resulting in direct may be expressed at any one of the multiple metabolic transforma- hepatocellular damage, but also participates in redox cycling, tions that a chemical might undergo. Human liver P450s 3A4, thereby generating reactive O2 species (ROS) and consequent 2C9, 2D6, 2C19, 1A2, and 2B6 are responsible for about 75% of oxidative stress that greatly enhance acetaminophen-induced all clinically relevant phase I drug metabolism (Figure 4–4), and hepatotoxicity. thus for about 60% of all physiologic drug biotransformation and The chemical and toxicologic characterization of the elec- elimination. Thus, genetic polymorphisms of these enzymes, by sig- trophilic nature of the reactive acetaminophen metabolite has nificantly influencing phase I drug metabolism, can alter their phar- led to the development of effective antidotes—cysteamine and macokinetics and the magnitude or the duration of drug response N-acetylcysteine (NAC; Acetadote; Mucomyst). Administration and associated events. of N-acetylcysteine (the safer of the two) within 8–16 hours Three P450 genetic polymorphisms have been particularly after acetaminophen overdosage has been shown to protect vic- well characterized, affording some insight into possible under- tims from fulminant hepatotoxicity and death (see Chapter 58). lying molecular mechanisms, and are clinically noteworthy, Administration of GSH is not effective because it does not cross as they require therapeutic dosage adjustment. The first is the cell membranes readily. debrisoquin-sparteine oxidation type of polymorphism, which 66 SECTION I Basic Principles TABLE 4–4 Some examples of genetic polymorphisms in phase I and phase II drug metabolism. Enzyme Involved Defect Genotype Drug and Therapeutic Use Clinical Consequences1 CYP1A2 N-Demethylation EM Caffeine (CNS stimulant) Reduced CNS stimulation due to increased gene inducibility and thus increased metabolism/clearance in cigarette smokers and frequent ingesters of omeprazole. N-Demethylation PM Caffeine (CNS stimulant) Enhanced CNS stimulation. CYP2A6 Oxidation PM Nicotine Nicotine toxicity. Lesser craving for frequent (cholinoceptorstimulant) cigarette smoking. Oxidation EM Nicotine Increased nicotine metabolism. Greater (cholinoceptorstimulant) craving for frequent cigarette smoking. Oxidation PM Coumarin (anticoagulant) Increased risk of bleeding. Oxidation EM Coumarin (anticoagulant) Increased clearance. Greater risk of thrombosis. CYP2B6 Oxidation, PM Cyclophosphamide, ifosfamide Reduced clearance. Increased risk of ADRs. N-Dechloroethylation (anti-cancer) Oxidation PM Efavirenz, nevirapine (anti-HIV) Reduced clearance. Increased risk of ADRs. CYP2C8 Hydroxylation PM Repaglinide, rosiglitazone, Reduced clearance. Increased risk of ADRs. pioglitazone (antidiabetic) Hydroxylation PM Paclitaxel (anti-cancer) Reduced clearance. Increased risk of ADRs (myelosuppression). N-Deethylation/ PM Amodiaquine, chloroquine Reduced clearance. Increased risk of ADRs. N-Dealkylation (antimalarial) N-Deethylation PM Amiodarone (antiarrhythmic) Reduced clearance. Increased risk of ADRs. CYP2C9 Hydroxylation PM Celecoxib, diclofenac, flurbipro- Reduced clearance. Increased risk of ADRs. fen, S-ibuprofen (NSAIDs) Hydroxylation PM S-Warfarin, S-acenocoumarol Enhanced bleeding risk. Clinically highly (anticoagulants) relevant. Dose adjustment required. Hydroxylation PM Tolbutamide (antidiabetic) Cardiotoxicity. Hydroxylation PM Phenytoin (antiepileptic) Nystagmus, diplopia, and ataxia. CYP2C19 N-Demethylation PM Amitriptyline, clomipramine Reduced clearance. Increased risk of ADRs. (antidepressants) Dose adjustment required. Oxidation PM Moclobemide (MAOI) N-Demethylation PM Citalopram (SSRI) Increased risk of gastrointestinal side effects. O-Demethylation PM Omeprazole (PPI) Increased therapeutic efficacy. Hydroxylation PM Mephenytoin (antiepileptic) Overdose toxicity. N-Demethylation EM Escitalopram (antidepressants) Increased gene transcription resulting in increased activity and thus reduced therapeutic efficacy. O-Demethylation EM Omeprazole (PPI) Reduced therapeutic efficacy. Hydroxylation EM Tamoxifen (anti-cancer) Increased metabolic activation, increased therapeutic efficacy; reduced risk of relapse. Dose adjustment required. Oxidative cyclization EM Chlorproguanil (antimalarial) Increased metabolic activation, increased therapeutic efficacy. Dose adjustment required. Oxidation EM Clopidogrel (antiplatelet) Increased metabolic activation, increased therapeutic efficacy. Dose adjustment required. CYP2D6 Oxidation PM Bufuralol (β-adrenoceptor Exacerbation of β blockade, nausea. blocker) O-Demethylation PM Codeine (analgesic) Reduced metabolic activation to morphine and thus reduced analgesia. Oxidation PM Debrisoquin (antihypertensive) Orthostatic hypotension. (continued) CHAPTER 4 Drug Biotransformation 67 TABLE 4–4 Some examples of genetic polymorphisms in phase I and phase II drug metabolism. (Continued) Enzyme Involved Defect Genotype Drug and Therapeutic Use Clinical Consequences1 N-Demethylation PM Nortriptyline (antidepressant) Reduced clearance. Increased risk of ADRs. Oxidation PM Sparteine Oxytocic symptoms. O-Demethylation PM Dextromethorphan Reduced clearance. Increased risk of ADRs. (antitussive) O-Demethylation PM Tramadol (analgesic) Increased risk of seizures. Hydroxylation PM Tamoxifen (anti-cancer) Reduced metabolic activation to the therapeutically active endoxifen and thus reduced therapeutic efficacy. O-Demethylation UM Codeine (analgesic) Increased metabolic activation to morphine and thus increased risk of respiratory depression. N-Demethylation UM Nortriptyline (antidepressant) Reduced therapeutic efficacy due to increased clearance. O-Demethylation UM Tramadol (analgesic) Reduced therapeutic efficacy due to increased clearance. CYP3A4 PM? All drugs metabolized by this Reduced clearance. Dose adjustment may be enzyme would be potentially required to avoid drug-drug interactions. affected CYP3A5 PM? Saquinavir, and other CYP3A Usually less catalytically active than CYP3A4. substrates A higher frequency of a functional CYP3A5*1 allele is seen in Africans than in Caucasians; the latter most often carry the defective CYP3A5*3 allele. This may significantly affect therapeutics of CYP3A substrates in CYP3A5*1 or CYP3A5*3 homozygous individuals. ALDH Aldehyde PM Ethanol (recreational drug) Facial flushing, hypotension, tachycardia, dehydrogenation nausea, vomiting. BCHE Ester hydrolysis PM Succinylcholine (muscle Prolonged apnea. relaxant) Mivacurium (neuromuscular Prolonged muscle paralysis. blocker) Cocaine (CNS stimulant) Increased blood pressure, tachycardia, ventricular arrhythmias. GST GSH-conjugation PM Acetaminophen (analgesic), Impaired GSH conjugation due to gene Busulfan (anti-cancer) deletion. NAT2 N-Acetylation PM Hydralazine (antihypertensive) Lupus erythematosus-like syndrome. N-Acetylation PM Isoniazid (antitubercular) Peripheral neuropathy. TPMT S-Methylation PM 6-Thiopurines (anti-cancer) Myelotoxicity. UGT1A1 Glucuronidation PM Bilirubin (heme metabolite) Hyperbilirubinemia. Irinotecan (anti-cancer) Reduced clearance. Dose adjustment may be required to avoid toxicity (GI dysfunction, immunosuppression). 1 Observed or predictable. ADR, adverse drug reaction; EM, extensive metabolizer; PM, poor metabolizer; UM, ultrarapid metabolizer. apparently occurs in 3–10% of Caucasians and is inherited as an correlates with a higher risk of relapse in patients with breast autosomal recessive trait. In affected individuals, the CYP2D6- cancer treated with tamoxifen, an anticancer drug that relies on its dependent oxidations of debrisoquin and other drugs (Table 4–2; CYP2D6-dependent metabolic activation to endoxifen for its effi- Figure 4–6) are impaired. These defects in oxidative drug metabo- cacy. More recently, however, another polymorphic genotype has lism are probably co-inherited. The precise molecular basis for the been reported that results in ultrarapid metabolism of relevant defect appears to be faulty expression of the P450 protein due to drugs due to the presence of CYP2D6 allelic variants with up to either defective mRNA splicing or protein folding, resulting in 13 gene copies in tandem. This ultrarapid metabolizer (UM) gen- little or no isoform-catalyzed drug metabolism and thereby con- otype is most common in Ethiopians and Saudi Arabians, popula- ferring a poor metabolizer (PM) phenotype. This PM phenotype tions that display it in up to one third of individuals. As a result, 68 SECTION I Basic Principles 25 to nirvanol, which accumulates in much higher concentrations. Thus, PMs of mephenytoin show signs of profound sedation 20 and ataxia after doses of the drug that are well tolerated by normal metabolizers. Two defective CYP2C19 variant alleles Relative frequency (CYP2C19*2 and CYP2C19*3), the latter predominant in Asians, 15 are largely responsible for the PM genotype. The molecular bases include splicing defects resulting in a truncated, nonfunctional 10 protein. CYP2C19 is responsible for the metabolism of various clinically relevant drugs (Table 4–4). Thus, it is clinically impor- URM EM PM tant to recognize that the safety of each of these drugs may be 5 severely reduced in persons with the PM phenotype. On the other hand, the PM phenotype can notably increase the therapeutic 0 efficacy of omeprazole, a proton-pump inhibitor, in gastric ulcer –2.0 –1.0 0 1.0 2.0 and gastroesophageal reflux diseases (see Chapter 5 for additional Log10 metabolic ratio discussion of the CYP2C19 polymorphism). Another CYP2C19 variant allele (CYP2C19*17) exists that is FIGURE 4–6 Genetic polymorphism in debrisoquin associated with increased transcription and thus higher CYP2C19 4-hydroxylation by CYP2D6 in a Caucasian population. The semilog frequency distribution histogram of the metabolic ratio (MR; defined expression and even higher functional activity than that of the as percent of dose excreted as unchanged debrisoquin divided by wild type CYP2C19-carrying EMs. Individuals carrying this the percent of dose excreted as 4-hydroxydebrisoquin metabolite) in CYP2C19*17 allele exhibit higher metabolic activation of pro- the 8-hour urine collected after oral ingestion of 12.8 mg debrisoquin drugs such as the breast cancer drug tamoxifen, the antimalarial sulfate (equivalent to 10 mg free debrisoquin base). Individuals with chlorproguanil, and the antiplatelet drug clopidogrel. The former MR values >12.6 were phenotyped as poor metabolizers (PM, red event is associated with a lower risk of breast cancer relapse, and bars), and those with MR values 0.2 were designated as the latter event with an increased risk of bleeding. Carriers of the extensive metabolizers (EM, blue bars). Those with MR values CYP2C19*17 allele are also known to enhance the metabolism 300 mcg/mL, less toxic and more water-soluble APAP-glucuronide and respectively (Chapter 3). Given that at 48 hours after inges- APAP-sulfate, both of which are eliminated in the urine tion (ie, 24 half-lives later), the patient’s APAP blood level (Figure 4–5). Five percent of parent APAP is converted by is 75 mcg/mL, it is obvious that her initial APAP levels phase I P450 enzymes into a reactive toxic product that is were dangerously above the toxic range, and thus upon ED conjugated by GSH, excreted in the urine, and thus detoxi- admission, her liver function tests are consistent with fied. However, APAP’s safety may be greatly compromised ongoing liver failure. She should be given N-acetylcysteine, in mixed drug overdoses, ie, when ingested with other drugs the APAP-specific antidote (Acetadote, Mucomyst; see such as hydrocodone, duloxetine, and carisoprodol, which Chapter 58) and continuous intravenous glucose infusion to compete with APAP for phase II-dependent elimination or provide the precursor (glucose) for generating the UDPGA for cellular cofactors (GSH, UDPGA, PAPS) involved in cofactor required for APAP glucuronidation, as well as the these processes. Accordingly, more APAP is diverted into fluid to induce urine output and accelerate APAP-metabolite its hepatotoxic reactive metabolite pathway, resulting in elimination. 5 C H A P T E R Pharmacogenomics Jennifer E. Hibma, PharmD, & Kathleen M. Giacomini, PhD C ASE STUDY A 35-year-old male with newly diagnosed human immu- experienced visible yellow discoloration of the skin and eyes. nodeficiency virus (HIV) infection was prescribed an anti- Blood samples were drawn, and grade 4 hyperbilirubinemia retroviral regimen, which included the protease inhibitor was documented. When atazanavir was discontinued and atazanavir 300 mg to be taken by mouth once daily, along the antiretroviral regimen was modified to include lopinavir, with ritonavir, a pharmacokinetic enhancer, and two nucleo- the plasma levels of bilirubin returned to the normal range, side analog antiretroviral agents. Liver function and renal and skin and eye color were cleared. Could a UGT1A1*28 function were normal. After 1 year of treatment, the patient polymorphism have led to the adverse effects? Pharmacogenomics, the study of genetic factors that underlie the past decade has ushered in “precision medicine,” also known variation in drug response, is a modern term for pharmacoge- as “stratified or personalized medicine,” in which genetic netics. Pharmacogenomics implies a recognition that more than information is used to guide drug and dosing selection for sub- one genetic variant may contribute to variation in drug response. groups of patients or individual patients in medical practice. The Historically, the field began with observations of severe adverse Clinical Pharmacogenetics Implementation Consortium (CPIC) drug reactions in certain individuals, who were found to harbor published a series of guidelines for using genetic information in genetic variants in drug-metabolizing enzymes. As a scientific selecting medications and in dosing. These highly informative field, pharmacogenomics has advanced rapidly since the sequenc- guidelines are being used by practitioners in prescribing drugs to ing of the human genome. In the last decade, powerful genome- more effectively treat patients. In this chapter, we begin with a case wide association (GWA) studies, in which hundreds of thousands study and then describe genetic variants that are determinants of of genetic variants across the genome are tested for association drug response. Where appropriate, CPIC recommendations are with drug response, led to the discovery of many other important included to provide information on how to use genetic variant polymorphisms that underlie variation in both therapeutic and data appropriately in therapeutic medicine. adverse drug response. In addition to polymorphisms in genes The description in this chapter of DNA sequence variations that encode drug-metabolizing enzymes, it is now known that in germline DNA involves a number of terms that describe the polymorphisms in genes that encode transporters, human leu- nature of the variations and their locations within the genome. kocyte antigen (HLA) loci, cytokines, and various other proteins A glossary of commonly used terms is presented in the Glossary are also predictive of variation in therapeutic and adverse drug Table. Some of the more common and important variations are responses. In addition to the new discoveries that have been made, described in the text that follows. 74