Ch-6: The Second Law of Thermodynamics PDF
Document Details
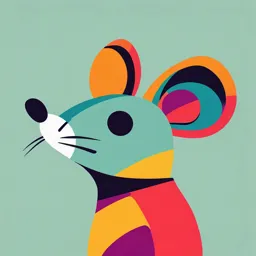
Uploaded by GratefulMossAgate5506
HITEC University
Tags
Summary
These are lecture notes on the second law of thermodynamics, specifically covering heat engines and refrigerators. The notes include details on thermodynamic concepts and provide examples and problems.
Full Transcript
Ch-6: The Second Law of Thermodynamics Book: ▪ Thermodynamics: An Engineering Approach by Yunus A. Cengel, Michael A. Boles, 5th Ed. (Chap 6) ▪ Applied Thermodynamics by TD Eastop and A McConkey, 5th Ed. ▪ Fundamentals of Thermodynamics by Borgnakke and Sontag, 8th Ed. (...
Ch-6: The Second Law of Thermodynamics Book: ▪ Thermodynamics: An Engineering Approach by Yunus A. Cengel, Michael A. Boles, 5th Ed. (Chap 6) ▪ Applied Thermodynamics by TD Eastop and A McConkey, 5th Ed. ▪ Fundamentals of Thermodynamics by Borgnakke and Sontag, 8th Ed. (Ch. 5) Mechanical Engineering Dept. HITEC Univ. 1 THE SECOND LAW OF THERMODYNAMICS Introduction to the Second Law ▪ Hot cup of coffee gets cooled off when exposed to surrounding →Energy lost by coffee = Energy gained by Surroundings →Here, First Law of Thermodynamics is satisfied ▪ HOWEVER, converse is NOT true → i.e. Taking out Heat Energy from Surroundings ≠ Coffee getting hot → Still, First Law of Thermodynamics is satisfied ! Mechanical Engineering Dept. HITEC Univ. 2 THE SECOND LAW OF THERMODYNAMICS Introduction to the Second Law ▪ Heating of a room by Electric heater; by passing Electric Current through the Resistor →Electric Energy supplied to the heater = Energy transferred to the Surroundings ( room air ) →Here, First Law of Thermodynamics is satisfied ▪ HOWEVER, converse is NOT true. →Transferring Heat to the wire ≠ Equivalent amount of Electric Energy generated in wire →Still, First Law of Thermodynamics is satisfied ! Mechanical Engineering Dept. HITEC Univ. 3 THE SECOND LAW OF THERMODYNAMICS Introduction to the Second Law ▪ Paddle Wheel mechanism operated by falling mass ▪ Paddle wheel rotates as mass falls down and stirs the fluid inside the container ▪ Decrease in Potential Energy of the mass = Increase in Internal Energy of the fluid → Here, First Law of Thermodynamics is satisfied. ▪ HOWEVER, converse is NOT true. → Transferring Heat to the Paddle Wheel ≠ Raising the mass. → Still, First Law of Thermodynamics is satisfied ! Gasoline is used as a car drives up a hill, but the fuel in the gasoline tank cannot be restored to its original level when the car coasts down the hill Mechanical Engineering Dept. HITEC Univ. 4 THE SECOND LAW OF THERMODYNAMICS Introduction to the Second Law ▪ From these day – to – day life examples, it can be clearly seen that; Satisfying the First Law of Thermodynamics does not ensure for a Process to occur actually ▪ Processes proceed in certain direction; but may not in Reverse direction ▪ First Law of Thermodynamics has no restriction on the DIRECTION of a Process to occur ▪ This Inadequacy of the First Law of Thermodynamics; to predict whether the Process can occur is solved by introduction of the Second Law of Thermodynamics A process must satisfy both the first and second laws of thermodynamics to proceed Mechanical Engineering Dept. HITEC Univ. 5 THE SECOND LAW OF THERMODYNAMICS Introduction to the Second Law MAJOR USES OF THE SECOND LAW ▪ Second law may be used to identify the Direction of processes ▪ Second law also asserts that energy has Quality as well as Quantity o The First Law is concerned with the Quantity of energy and the transformations of energy from one form to another with no regard to its quality o The Second Law provides the necessary means to determine the Quality as well as the degree of degradation of energy during a process ▪ Second law of Thermodynamics is also used in determining the Theoretical Limits for the performance of commonly used engineering systems → such as Heat Engines and Refrigerators → as well as predicting the degree of completion of Chemical Reactions Mechanical Engineering Dept. HITEC Univ. 6 THE SECOND LAW OF THERMODYNAMICS Thermal Energy Reservoirs Thermal Energy Reservoir : Hypothetical body with relatively very large Thermal Energy Capacity ( mass x Sp. Heat ) that can supply or absorb finite amount of Heat without undergoing change in Temperature e.g. ocean, lake, atmosphere, two-phase system, Bodies with relatively large thermal industrial furnace, etc. masses can be modeled as thermal energy reservoirs A source supplies energy in the form of heat, and a → Reservoir that Supplies Energy in the form of Heat is known as SOURCE sink absorbs it → Reservoir that Absorbs Energy in the form of Heat is known as SINK ▪ Any physical body whose Thermal Energy Capacity is large relative to the amount of energy it supplies or absorbs can be modeled as Reservoir → E.g. Heat Dissipation from a TV set in the Room Mechanical Air Dept. HITEC Univ. Engineering 7 THE SECOND LAW OF THERMODYNAMICS HEAT ENGINES ▪ Mechanical Work done by the shaft is first converted to the internal energy of the water → may then leave the water as heat ▪ Any attempt to reverse this process will fail → transferring heat to the water does not cause the shaft to rotate → From such examples, it can be concluded that, 1. Work can be converted to Heat. 2. BUT, Converting Heat to Work requires Special Devices → These devices are known as Heat Engines Mechanical Engineering Dept. HITEC Univ. 8 THE SECOND LAW OF THERMODYNAMICS HEAT ENGINES Mechanical Engineering Dept. HITEC Univ. 9 THE SECOND LAW OF THERMODYNAMICS HEAT ENGINES Heat Engines are devices that convert Heat to Work: 1. They receive heat from a high-temperature source (solar energy, oil furnace, nuclear reactor, etc.) 2. convert part of this heat to work → usually in the form of a rotating shaft) 3. reject the remaining waste heat to a low-temperature sink (the atmosphere, rivers, etc.) 4. They operate on a cycle Heat Engines and other Cyclic Devices usually involve a Fluid to and from which heat is transferred while undergoing a cycle → called the Working Fluid Heat Engines are generally Work – Producing devices, e.g. Gas Turbines, I.C. Engines, Steam Power Plants, etc. Mechanical Engineering Dept. HITEC Univ. 10 THE SECOND LAW OF THERMODYNAMICS HEAT ENGINES Steam Power Plant Qin = Amount of heat supplied to steam in boiler from a high-temperature source (furnace) Qout = Amount of heat rejected from steam in condenser to a low temperature sink (the atmosphere, a river, etc.) Wout = Amount of work delivered by steam as it expands in turbine Win = Amount of work required to compress water to boiler pressure net work output of this power plant: A portion of the work output of a heat engine is consumed internally to Mechanical Engineering Dept. HITEC Univ. 11 maintain continuous operation THE SECOND LAW OF THERMODYNAMICS HEAT ENGINES Steam Power Plant ▪ four components of the steam power plant involve Mass Flow In and Out, and therefore they should be treated as Open Systems ▪ These components, together with the connecting pipes always contain the Same Fluid ▪ No mass enters or leaves this Combination System, → indicated by the Shaded Area ▪ For a closed system undergoing a cycle, change in internal energy ∆U is zero, and therefore the Net Work Output of the system is also equal to the Net Heat Transfer to the system Mechanical Engineering Dept. HITEC Univ. 12 THE SECOND LAW OF THERMODYNAMICS HEAT ENGINES Thermal Efficiency ▪ηth: Fraction of the heat input that is converted to net work output is a measure of the performance of a heat engine OR ▪ Cyclic devices of practical interest such as Heat Engines, Refrigerators, and Heat Pumps operate between a high-temperature medium (or reservoir) at temperature TH and a low-temperature medium (or reservoir) at temperature TL Some heat engines perform better than others (convert more of the heat Mechanical Engineering Dept. HITEC Univ. 13 they receive to work) THE SECOND LAW OF THERMODYNAMICS HEAT ENGINES Thermal Efficiency– contd-- ▪ To bring uniformity to the treatment of Heat Engines, Refrigerators, and Heat Pumps QH = magnitude of heat transfer between the cyclic device and the high temperature medium at temperature TH QL = magnitude of heat transfer between the cyclic device and the low temperature medium at temperature TL ⇒ ⇒ Mechanical Engineering Dept. HITEC Univ. 14 THE SECOND LAW OF THERMODYNAMICS HEAT ENGINES Thermal Efficiency– contd-- ▪ Thermal Efficiencies of work-producing devices are relatively low ▪ Ordinary spark-ignition automobile engines have a thermal efficiency of about 25 percent ▪ ηth is as high as 40 percent for Diesel Engines and Large Gas- turbine Plants and as high as 60 percent for large Combined Gas- steam Power Plants ▪ Even with the most efficient heat engines available today, almost One-half of the energy supplied ends up in the rivers, lakes, or the atmosphere as waste or useless energy Mechanical Engineering Dept. HITEC Univ. 15 THE SECOND LAW OF THERMODYNAMICS HEAT ENGINES Can we save Qout? ▪ In a steam power plant, the Condenser is the device where large quantities of Waste Heat is rejected to rivers, lakes, or the atmosphere ▪ Can we not just take the condenser out of the plant and save all that waste energy? ▪ The answer is, unfortunately, a firm NO for the simple reason that without a heat rejection process in a condenser, the cycle cannot be completed ▪ 100 kJ of heat is transferred to the gas in the cylinder from a source at 100°C, causing it to expand and to raise the loaded piston until the piston reaches the upper stops ▪ Work done on the load during this expansion process is equal to the increase in its Potential Energy, say 15 kJ ▪ Even under ideal conditions (weightless piston, no friction, no heat losses, and quasi-equilibrium expansion), amount of heat supplied to the gas is greater than the work done → part of the Heat Supplied is used to raise the Temperature of the gas Mechanical Engineering Dept. HITEC Univ. 16 THE SECOND LAW OF THERMODYNAMICS HEAT ENGINES Can we save Qout? ▪ Is it possible to transfer the 85 kJ of excess heat at 90°C back to the reservoir at 100°C for later use? ▪ Answer is again No → Heat is always transferred from a high temperature medium to a low-temperature one ▪ system must be brought into contact with a low- temperature reservoir, (at e.g. 20°C) → gas can return to its initial state by rejecting its 85 kJ of excess energy as heat to this reservoir ▪ This energy cannot be recycled → called Waste Energy ⇒ Every Heat Engine must waste some energy by transferring it to a low-temperature reservoir in order to complete the Cycle Mechanical Engineering Dept. HITEC Univ. 17 THE SECOND LAW OF THERMODYNAMICS HEAT ENGINES ▪ It is impossible to make a water wheel that converts all the energy of the inlet water into output shaft work ▪ There must be an outflow of water from the wheel, and this outflow must have some energy ▪ if this idea is extended to a Steam Engine (or any type of Heat Engine) by replacing the word Water by Heat → it is easy to conclude that when Heat at a certain ENERGY LEVEL (temperature) enters a work-producing heat engine, it must also exit the engine at a lower Mechanical Energy Engineering Dept. HITECLevel Univ. (temperature). 18 THE SECOND LAW OF THERMODYNAMICS Example HEAT ENGINES A coal-burning steam power plant produces a net power of 300 MW with an overall thermal efficiency of 32 percent. The actual air–fuel ratio in the furnace is calculated to be 12 kg air/kg fuel. The heating value of the coal is 28,000 kJ/kg. Determine: (a) the amount of coal consumed during a 24-hr period and (b) the rate of air flowing through the furnace ⇒ Mechanical Engineering Dept. HITEC Univ. 19 THE SECOND LAW OF THERMODYNAMICS Example HEAT ENGINES A coal-burning steam power plant produces a net power of 300 MW with an overall thermal efficiency of 32 percent. The actual air–fuel ratio in the furnace is calculated to be 12 kg air/kg fuel. The heating value of the coal is 28,000 kJ/kg. Determine: (a) the amount of coal consumed during a 24-hr period and (b) the rate of air flowing through the furnace Mechanical Engineering Dept. HITEC Univ. 20 THE SECOND LAW OF THERMODYNAMICS HEAT ENGINES The Second Law of Thermodynamics: Kelvin–Planck Statement It is impossible for any device that operates on a Cycle to receive heat from a single reservoir and produce a net amount of work → II Law applies only for a Cycle -- not for a process!! → As during an Isothermal Process the system can exchange heat with a single reservoir and yet deliver work ▪ No heat engine can have a thermal efficiency of 100 percent → OR as for a power plant to operate, the working fluid must exchange heat with the environment as well as the furnace ▪ Impossibility of having a 100% Efficient Heat Engine is not due to Friction or Other Dissipative Effects A heat engine that violates the Kelvin– → It is a limitation that applies to both the Idealized and the Actual Planck statement of the second law. heat engines Mechanical Engineering Dept. HITEC Univ. 21 THE SECOND LAW OF THERMODYNAMICS Refrigerators and Heat Pumps ▪ Transfer of heat from a low-temperature medium to a high-temperature one requires special devices called Refrigerators ▪ Refrigerators, like heat engines, are Cyclic Devices ▪ Working fluid used in the refrigeration cycle is called a Refrigerant ▪ most frequently used refrigeration cycle is the vapor- compression refrigeration cycle In a household refrigerator, the freezer compartment where heat is absorbed by the refrigerant serves as the EVAPORATOR, and coils behind the refrigerator where heat is dissipated to the kitchen air serve as the Condenser ▪ Air conditioners are refrigerators whose refrigerated space is a room or a building instead of the food compartment ▪COP of a Refrigerator decreases with decreasing refrigeration temperature Mechanical Engineering Dept. HITEC Univ. 22 → it is not economical to refrigerate to a lower temperature than needed THE SECOND LAW OF THERMODYNAMICS Refrigerators and Heat Pumps Refrigeration Cycle on Pv-diagram Mechanical Engineering Dept. HITEC Univ. 23 THE SECOND LAW OF THERMODYNAMICS Refrigerators and Heat Pumps Coefficient of Performance ▪ Efficiency of a refrigerator is expressed in terms of the coefficient of performance (COP) ▪ Objective of a Refrigerator is to remove heat (QL) from the refrigerated space Based on Conservation of energy principle for a cyclic device ⇒ value of COPR can be greater than Unity ⇒ amount of heat removed from the refrigerated space can be greater The objective of a refrigerator is to Mechanical Engineering Dept. HITEC Univ. 24 than the amount of work input remove QL from the cooled space THE SECOND LAW OF THERMODYNAMICS Refrigerators and Heat Pumps Heat Pumps ▪ Device that transfers heat from a low-temp medium to a high-temp one ▪ Heat Pump absorbs heat from a low-temp source, such as cold outside air in winter, and supplying this heat to the high-temp medium such as a House Objective of a heat pump is to ⇒ supply heat QH The work supplied into the warmer to a heat pump is space used to extract ⇒ energy from the cold outdoors and for fixed values of QL and QH carry it into the warm indoors Mechanical Engineering Dept. HITEC Univ. 25 THE SECOND LAW OF THERMODYNAMICS Refrigerators and Heat Pumps Problem Refrigerant-134a enters the condenser of a residential heat pump at 800 kPa and 35°C at a rate of 0.018 kg/s and leaves at 800 kPa as a saturated liquid. If the compressor consumes 1.2 kW of power, determine: (a) the COP of the heat pump and (b) the rate of heat absorption from the outside air. Mechanical Engineering Dept. HITEC Univ. 26 THE SECOND LAW OF THERMODYNAMICS Problem Refrigerators and Heat Pumps Refrigerant-134a enters the condenser of a residential heat pump at 800 kPa and 35°C at a rate of 0.018 kg/s and leaves at 800 kPa as a saturated liquid. If the compressor consumes 1.2 kW of power, determine: (a) the COP of the heat pump and (b) the rate of heat absorption from the outside air. Mechanical Engineering Dept. HITEC Univ. 27 THE SECOND LAW OF THERMODYNAMICS Refrigerators and Heat Pumps Mechanical Engineering Dept. HITEC Univ. 28 THE SECOND LAW OF THERMODYNAMICS Refrigerators and Heat Pumps The Second Law of Thermodynamics: Clausius Statement It is impossible to construct a device that operates in a cycle and produces no effect other than the transfer of heat from a Lower-temperature Body to a Higher-temperature Body ▪ It states that a refrigerator cannot operate unless its compressor is driven by an external power source, such as an Electric Motor ▪ This way, the net effect on the Surroundings involves the consumption of some energy in the form of work, in addition to the transfer of heat from a colder body to a warmer one ▪ To date, no experiment has been conducted that contradicts the second law, and this should be taken as sufficient proof of its validity Refrigerator that violates the Clausius Mechanical Engineering Dept. HITEC Univ. 29 statement of the second law THE SECOND LAW OF THERMODYNAMICS Equivalence of the Two Statements ▪ If a heat engine violates Kelvin–Planck statement i.e., ηth =100 % and QH = W ▪ W is then supplied to a refrigerator that removes heat in the amount of QL from low-temperature reservoir and rejects heat in the amount of QL + QH to the high- temperature reservoir ▪ During this process, high temperature reservoir receives a net amount of heat QL (difference between QL + QH and QH) ▪ combination of these two devices can be viewed as a Refrigerator → which transfers heat QL from a cooler body to a warmer one without requiring any input from outside → a violation of the Clausius Statement ⇒ Kelvin–Planck and the Clausius statements are Equivalent in their consequences ⇒ Any device that violates Kelvin statement also Mechanical Engineering Dept. HITEC Univ. 30 violates the Clausius statement, and vice versa THE SECOND LAW OF THERMODYNAMICS Perpetual-motion Machines ▪ Perpetual-motion machine: Any device that violates the First or the Second Law ▪ A device that violates the first law (by creating energy) → Perpetual-motion Machine of the first kind (PMM1) ▪ A device that violates the second law → Perpetual-motion Machine of the second kind (PMM2) ▪ Despite numerous attempts, no perpetual-motion machine is known to have work → Part of the electricity generated by the plant is to be used to power the resistors as well as the pump → rest of the electric energy is to be supplied to the electric network as the net work output system enclosed by the shaded area is continuously supplying energy to the outside at a rate of without receiving any energy → system is creating energy at a rate of → A clear violation of First Law A perpetual-motion machine that Mechanical Engineering Dept. HITEC Univ. 31 violates the first law (PMM1) THE SECOND LAW OF THERMODYNAMICS Perpetual-motion Machines ▪ An inventor suggests getting rid of the wasteful component “Condenser” and sending the steam to the pump as soon as it leaves the turbine → All the heat transferred to the steam in the boiler will be converted to work → Power Plant will have a theoretical efficiency of 100 percent ▪ It’s a PMM2 → it works on a Cycle and does a net amount of work while exchanging heat with a single reservoir (the furnace) only → It satisfies the First Law but violates the Second Law, and therefore it will not work Mechanical Engineering Dept. HITEC Univ. 32 THE SECOND LAW OF THERMODYNAMICS REVERSIBLE AND IRREVERSIBLE PROCESSES ▪ Reversible process: A process that can be reversed without leaving any trace on the surroundings → both the System and the Surroundings are returned to their initial states at the end of the reverse process → possible only if the net heat and net work exchange between the system and the surroundings is zero for the combined (original and reverse) process ▪ Irreversible process: A process that is not reversible → if the system and all parts of its surroundings cannot be exactly restored to their respective initial states after the process has occurred o All the processes occurring in nature are Irreversible o Why are we interested in reversible processes? → (1) they are easy to analyze and → (2) they serve as idealized models (theoretical limits) to which actual processes can be compared o Some processes are more irreversible than others o We try to approximate reversible processes Mechanical Engineering Dept. HITEC Univ. 33 THE SECOND LAW OF THERMODYNAMICS REVERSIBLE AND IRREVERSIBLE PROCESSES → as friction is eliminated, the states of both the pendulum and its surroundings would be completely restored at the end of each period of motion → A system consisting of a gas adiabatically compressed and expanded in a frictionless piston–cylinder assembly is Reversible o work done on the gas during the compression would equal the work done by the gas during the expansion o The more closely we approximate a reversible process, the more work delivered by a work-producing device or the less work required by a work- consuming device o Concept of reversible processes leads to the definition of the Second Law Efficiency for actual processes: → degree of approximation to the corresponding reversible processes Mechanical Engineering Dept. HITEC Univ. 34 THE SECOND LAW OF THERMODYNAMICS REVERSIBLE AND IRREVERSIBLE PROCESSES Irreversibilities ▪ Factors that cause a process to be irreversible are called Irreversibilities ▪ They include: friction, unrestrained expansion, mixing of two fluids, heat transfer across a finite temperature difference, electric resistance, inelastic deformation of solids, and chemical reactions ▪ The presence of any of these effects renders a process Irreversible Friction: Energy supplied as work is eventually converted to heat during friction and is transferred to the bodies in contact, as evidenced by a temperature rise at the interface →When direction of motion is reversed, the bodies are restored to their original position, but the interface does not cool, and heat is not converted back to work →Since the system (the moving bodies) and the surroundings cannot be returned to their original states, this process is irreversible Friction renders a process irreversible Mechanical Engineering Dept. HITEC Univ. 35 THE SECOND LAW OF THERMODYNAMICS REVERSIBLE AND IRREVERSIBLE PROCESSES Irreversibilities –contd-- ▪ The transformation of work into molecular internal energy → Dissipation of Energy ▪ Dissipation of Energy → Transition of Macroscopic Motion into Chaotic Molecular Motion, the reverse of which is not possible without violating Second Law Mechanical Engineering Dept. HITEC Univ. 36 THE SECOND LAW OF THERMODYNAMICS REVERSIBLE AND IRREVERSIBLE PROCESSES Irreversibilities –contd-- Unrestrained Expansion of a gas is an Irreversible Process: → only way to restore the system to its original state is to compress it to its initial volume, while transferring heat from the gas until it reaches its initial temperature → amount of heat transferred from the gas equals the amount of work done on the gas by the surroundings → Restoration of the surroundings involves conversion of this heat completely to work, which would violate the second law → surroundings are not restored to their initial state, indicating that the unrestrained expansion was an irreversible process → flow through a restriction like a Valve or Throttle is also an example of Unrestrained Expansion Mechanical Engineering Dept. HITEC Univ. 37 THE SECOND LAW OF THERMODYNAMICS REVERSIBLE AND IRREVERSIBLE PROCESSES Irreversibilities –contd-- Heat Transfer Through a Finite Temperature Difference ▪ Heat is transferred from the warmer room air to the cooler soda ▪ only way this process can be reversed and the soda restored to its original temperature is to provide Refrigeration → which requires some work input ▪ Internal Energy of the surroundings will increase by an amount equal in magnitude to the work supplied to the refrigerator ▪ restoration of the surroundings to the initial state can be done only by converting this excess internal energy completely to work, which is impossible to do without violating the Second Law → Heat transfer can occur only when there is a Temperature Difference between a system and its surroundings → it is Physically Impossible to have a Reversible Heat Transfer Process → heat transfer process becomes less and less irreversible as ΔT between the two bodies approaches zero Mechanical Engineering Dept. HITEC Univ. 38 THE SECOND LAW OF THERMODYNAMICS REVERSIBLE AND IRREVERSIBLE PROCESSES Internally and Externally Reversible Processes ▪ Internally Reversible Process: If no irreversibilities occur within the boundaries of the system during the process, E.g. Water Condensing at 100 oC in a copper tube and exposed to 20 oC Ambient ▪ Externally Reversible: If no irreversibilities occur outside the system boundaries ▪ Totally Reversible Process: It involves no irreversibilities within the system or its surroundings o A Totally Reversible Process involves no heat transfer through a finite temperature difference, no non- quasi-equilibrium changes, and no friction or other dissipative effects constant-pressure (thus constant temperature) phase- change process Mechanical Engineering Dept. HITEC Univ. 39 THE SECOND LAW OF THERMODYNAMICS THE CARNOT CYCLE ▪ If the efficiency of all heat engines is less than 100%, what is the Most Efficient Cycle we can have? ▪ Efficiency of a heat-engine cycle greatly depends on how the individual processes that make up the cycle are executed ▪ Net Work, thus the Cycle Efficiency, can be maximized by using processes that require the least amount of work and deliver the most i.e., → by using Reversible Processes ▪ Assume a Heat Engine, which operates between the given high temperature and low-temperature reservoirs, does so in a cycle in which every process is reversible → This is the Most Efficient Cycle that can operate between two constant-temperature reservoirs → It is called the Carnot Cycle and is named after a French Engineer, Sadi Carnot (1796 – 1832) ▪ Heat Engines and Refrigerators that work on Reversible Cycles serve as models to which Actual Heat Engines and Refrigerators can be compared Mechanical Engineering Dept. HITEC Univ. 40 THE SECOND LAW OF THERMODYNAMICS THE CARNOT CYCLE Execution of the Carnot Cycle in a Closed System ✓ Reversible Isothermal Expansion (process 1-2, TH = constant) ✓ Reversible Adiabatic Expansion (process 2-3, temperature drops from TH to TL) ✓ Reversible Isothermal Compression (process 3-4, TL = constant) ✓ Reversible Adiabatic Compression (process 4-1, temperature rises from TL to TH) Further detail of Processes refer to: Chap-6 Mechanical Engineering Dept. HITEC Univ. 41 (page: 299-301) Cengel, 5th Ed. THE SECOND LAW OF THERMODYNAMICS THE CARNOT CYCLE ▪ Area under Curve 1-2-3 is the work done by the gas during the Expansion part of the cycle ▪ Area under Curve 3-4-1 is the work done on the gas during the Compression part of the cycle ▪ Area enclosed by the path of the cycle (area 1-2-3-4-1) is the difference between these two and represents the net work done during the cycle ▪ if gas is compressed at state 3 adiabatically instead of isothermally in an effort to save QL, we would end up back at state 2, retracing the process path 3-2 → But no net work output from this engine would be obtained → illustrates the necessity of a heat engine exchanging heat with at least two reservoirs at different temperatures to operate in a cycle and produce a net amount of work Mechanical Engineering Dept. HITEC Univ. 42 THE SECOND LAW OF THERMODYNAMICS THE CARNOT CYCLE The Reversed Carnot Cycle ▪ Carnot Heat-Engine cycle is a totally reversible cycle all the processes that comprise it can be reversed → Then it becomes the Carnot Refrigeration Cycle → Heat in the amount of QL is absorbed from the low- temperature reservoir → heat in the amount of QH is rejected to a high- temperature reservoir, and → work input of Wnet,in is required to accomplish all this Mechanical Engineering Dept. HITEC Univ. 43 THE SECOND LAW OF THERMODYNAMICS THE CARNOT CYCLE The Reversed Carnot Cycle Carnot Heat Engine Cycle Carnot Refrigeration Cycle Mechanical Engineering Dept. HITEC Univ. 44 THE SECOND LAW OF THERMODYNAMICS THE CARNOT PRINCIPLES 1. Efficiency of an Irreversible Heat Engine is always less than the efficiency of a Reversible One operating between the same two reservoirs 2. Efficiencies of all Reversible Heat Engines operating between the same two reservoirs are the same → two statements can be proved by demonstrating that the violation of either statement results in the violation of the second law of thermodynamics Self Study: Page 297-298, Cengel 8th ed. Mechanical Engineering Dept. HITEC Univ. 45 THE SECOND LAW OF THERMODYNAMICS THE THERMODYNAMIC TEMPERATURE SCALE ▪ Temperature Scale that is independent of the properties of the substances that are used to measure temperature is called a Thermodynamic Temperature Scale ▪ Efficiencyof a Reversible Engine is independent of the working fluid employed and its properties, the way the cycle is executed, or the type of Reversible Engine used ▪ SinceEnergy Reservoirs are characterized by their Temperatures, the Thermal Efficiency of Reversible Heat Engines is a function of the Reservoir Temperatures only OR ⇒ Mechanical Engineering Dept. HITEC Univ. 46 THE SECOND LAW OF THERMODYNAMICS THE THERMODYNAMIC TEMPERATURE SCALE ⇒Relation between the Thermal Efficiency of a Carnot Cycle and the Absolute Temperatures of the two reservoirs is: Section 5.8: Fundamentals of Thermodynamics by Borgnakke and Sontag, 8th Ed. : derivation of correlation QH / QL = TH / TL Any Heat Engine Carnot Heat Engine → Highest Efficiency a heat engine operating between the two thermal energy reservoirs at temperatures TL and TH can have → TL and TH in are Absolute Temperatures → Using °C or °F gives results grosslyMechanical in error Engineering Dept. HITEC Univ. 47 THE SECOND LAW OF THERMODYNAMICS THE CARNOT HEAT ENGINE →Performance of actual heat engines should not be compared with efficiency of 100 percent →They should be compared to the efficiency of a Reversible Heat Engine operating between the same temperature limits → Because this is the True Theoretical Upper Limit for the efficiency, not 100 percent No heat engine can have a higher efficiency than a reversible heat engine operating between the same high- and low-temperature reservoirs Mechanical Engineering Dept. HITEC Univ. 48 THE SECOND LAW OF THERMODYNAMICS THE CARNOT HEAT ENGINE Problem An inventor claims to have developed a power cycle capable of delivering a net work output of 300 kJ for an energy input by heat transfer of 700 kJ. The system undergoing the cycle receives the heat transfer from hot gases at a temperature of 500 K and discharges energy by heat transfer to the atmosphere at 290 K. Evaluate this claim. Problem A heat engine is operating on a CARNOT CYCLE and has a thermal efficiency of 55 percent. The waste heat from this engine is rejected to a nearby lake at 60 oF at a rate of 800 Btu/min. Determine: (a) the power output of the engine and (b) the temperature of the source. Mechanical Engineering Dept. HITEC Univ. 49 THE SECOND LAW OF THERMODYNAMICS THE CARNOT HEAT ENGINE Problem An inventor claims to have developed a power cycle capable of delivering a net work output of 300 kJ for an energy input by heat transfer of 700 kJ. The system undergoing the cycle receives the heat transfer from hot gases at a temperature of 500 K and discharges energy by heat transfer to the atmosphere at 290 K. Evaluate this claim. ⇒ Mechanical Engineering Dept. HITEC Univ. 50 THE SECOND LAW OF THERMODYNAMICS THE CARNOT HEAT ENGINE Problem A heat engine is operating on a CARNOT CYCLE and has a thermal efficiency of 55 percent. The waste heat from this engine is rejected to a nearby lake at 60 oF at a rate of 800 Btu/min. Determine: (a) the power output of the engine and (b) the temperature of the source. ⇒ Mechanical Engineering Dept. HITEC Univ. 51 THE SECOND LAW OF THERMODYNAMICS The Quality of Energy → Thermal Efficiency decreases as the Source Temperature is lowered → Efficiency values show that energy has Quality as well as Quantity → Higher the temperature, the higher the Quality of the energy ▪ Large quantities of solar energy, for example, can be stored in large bodies of water called Solar Ponds at about 350 K → Efficiency of Solar Pond Power Plants is very low (under 5 percent) because of the low quality of the energy stored in the source When heat is transferred from a high-temperature body to a lower temperature one, it is Degraded since less of it now can be converted to work The fraction of heat that can be converted to work as a function of source temperature Mechanical Engineering Dept. HITEC Univ. 52 THE SECOND LAW OF THERMODYNAMICS Problem A Carnot Heat Engine receives heat from a reservoir at 900°C at a rate of 800 kJ/min and rejects the waste heat to the ambient air at 27°C. The entire work output of the heat engine is used to drive a Refrigerator that removes heat from the refrigerated space at - 5°C and transfers it to the same ambient air at 27 °C. Determine: (a) the Maximum Rate of Heat Removal from the refrigerated space and (b) the total rate of heat rejection to the ambient air. Mechanical Engineering Dept. HITEC Univ. 53 THE SECOND LAW OF THERMODYNAMICS Problem A geothermal power plant uses geothermal liquid water extracted at 160°C at a rate of 440 kg/s as the heat source and produces 22 MW of net power. If the environment temperature is 25°C, determine: (a) the Actual Thermal Efficiency, (b) the Maximum Possible Thermal Efficiency, and (c) The actual rate of heat rejection from this power plant. Mechanical Engineering Dept. HITEC Univ. 54 THE SECOND LAW OF THERMODYNAMICS Problem A geothermal power plant uses geothermal liquid water extracted at 160°C at a rate of 440 kg/s as the heat source and produces 22 MW of net power. If the environment temperature is 25°C, determine: (a) the Actual Thermal Efficiency, (b) the Maximum Possible Thermal Efficiency, and (c) The actual rate of heat rejection from this power plant. Mechanical Engineering Dept. HITEC Univ. 55 THE SECOND LAW OF THERMODYNAMICS Practice Problems: Book: Yunus Cengel 8th Ed. ▪ Examples: 6.1 – 6.5 ▪ Problems: 6.1 – 6.15, 6.19 – 6.26, 6.28 – 6.45, 6.55 – 6.78, 6.84 – 6.91, 6.101 – 6.105 Mechanical Engineering Dept. HITEC Univ. 56