Concrete Technology-I Past Paper 2022-2023 PDF
Document Details
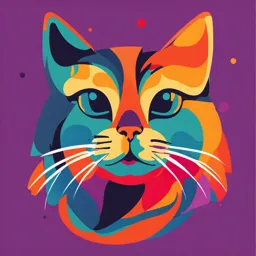
Uploaded by ArtisticMolybdenum
University of Halabja
2023
Tags
Summary
This document is a chapter from a course on concrete technology. It discusses cement, its historical background, manufacture, and types. The document seems to be part of a textbook or lecture notes from the University of Halabja for Civil Engineering students.
Full Transcript
University of Halabja Course Name: Concrete Technology-I Civil Engineering Department 2nd Year Students Date: 28/11/ 2023 First Semester 2022-2023...
University of Halabja Course Name: Concrete Technology-I Civil Engineering Department 2nd Year Students Date: 28/11/ 2023 First Semester 2022-2023 CHAPTER TWO CEMENT What is Cement Cement, in the general sense of the word, can be described as a material with adhesive and cohesive properties which make it capable of bonding mineral fragments into a compact whole. This definition embraces a large variety of cementing materials. Portland cement is made primarily from calcareous material, such as limestone or chalk, and from alumina and silica found as clay or shale. The cements of interest in the making of concrete have the property of setting and hardening under water by virtue of a chemical reaction with it and are, therefore, called hydraulic cements. Historical Background The history of cementing material is as old as the history of engineering construction. Some kind of cementing materials were used by Egyptians, Romans and Indians in their ancient constructions. It is believed that the early Egyptians mostly used cementing materials, obtained by burning gypsum. The story of the invention of Portland cement is, however, attributed to Joseph Aspdin, a Leeds builder and bricklayer, even though similar procedures had been adopted by other inventors. Joseph Aspdin took the patent of portland cement on 21st October 1824. The fancy name of portland was given owing to the resemblance of this hardened cement to the natural stone occurring at Portland in England. In his process Aspdin mixed and ground hard limestones and finely divided clay into the form of slurry and calcined it in a furnace similar to a lime kiln till the CO2 was expelled. The mixture so calcined was then ground to a fine powder. In the early period, cement was used for making mortar only. Later the use of cement was extended for making concrete. 1 As the use of Portland cement was increased for making concrete, engineers called for consistently higher standard material for use in major works. Association of Engineers, Consumers and Cement Manufacturers have been established to specify standards for cement. The German standard specification for Portland cement was drawn in 1877. The British standard specification was first drawn up in 1904. The first ASTM specification was issued in 1904. Manufacture of Portland Cement The raw materials required for manufacture of Portland cement are calcareous materials, such as limestone or chalk, and argillaceous material such as shale or clay. Cement factories are established where these raw materials are available in plenty The process of manufacture of cement consists of : (1) grinding the raw materials (2) mixing them intimately in certain proportions depending upon their purity and composition (3) burning them in a kiln at a temperature of about 1300 to 1500°C, at which temperature, the material sinters and partially fuses to form nodular shaped clinker The clinker is cooled and ground to fine powder with addition of about 3 to 5% of gypsum. The product formed by using this procedure is Portland cement. There are two processes known as “wet” and “dry” processes depending upon whether the mixing and grinding of raw materials is done in wet or dry conditions. The Mixture is fed into a rotary kiln. The kiln is slightly inclined. The mixture is fed at the upper end while pulverized coal ( or other source of heat) is blown in by an air blast at the lower end of the kiln, where the temperature may reach about 1500 C. As the mixture of raw materials moves down the kiln, it encounters a progressively higher temperature so that various chemical changes take place along the kiln : First any water is driven off and CO2 is liberated from the calcium carbonate. Further on, the dry material undergoes a series of chemical reactions until, finally, in the hottest part of the kiln, some 20 to 30 percent of the material becomes liquid, and lime, silica and alumina recombine. The mass then fuses into balls, 3 to 25 mm in diameter known as clinker. Afterward the clinker drops into coolers. The cool clinker which is very hard, is interground with gypsum in order to prevent flash- setting of the cement. 2 Diagrammatic representation of: (a) the wet process and (b) the dry process of manufacture of cement 3 TYPICAL SETUP OF CEMENT PLANT Comparison Wet process Dry Process the slurry contain 35 – 50 % of water quantity of water about 12 %) The amount of heat required is higher, So it Require The amount of heat required is lower, So it Require more fuel much less fuel The kiln and the equipment are grater The equipment used in the kiln is comparatively smaller Less maintenance because of low friction forces Need more maintenance between particles and kiln Less economic More economic (more production with lower fuel consumption More possible to get homogeneous mix Less accurate to control mixing of raw materials (Mixing of raw materials should be in powder form) 4 Chemical composition of Portland cement The raw materials used for the manufacture of cement consist mainly of lime, silica, alumina and iron oxide. These oxides interact with one another in the kiln at high temperature to form more complex compounds. The relative proportions of these oxide compositions are responsible for influencing the various properties of cement; in addition to rate of cooling and fineness of grinding. Table (Approximate Oxide Composition Limits of Ordinary Portland Cement) As mentioned earlier the oxides present in the raw materials when subjected to high clinkering temperature combine with each other to form complex compounds. The identification of the major compounds is largely based on R.H. Bogue’s work and hence it is called “Bogue‟s Compounds”. The four compounds usually regarded as major compounds are listed in table. Main Compounds of Portland Cement The calculation of the potential composition of Portland cement is based on the work of R. H. Bogue and others, and is often referred to as „Bogue composition‟. Bogue‟s equations for the percentages of main compounds in cement are given below. The terms in brackets represent the percentage of the given oxide in the total mass of cement 5 C3S = 4.07(CaO) – 7.60(SiO2) – 6.72(Al2O3) – 1.43(Fe2O3) – 2.85(SO3) C2S = 2.87(SiO2) – 0.75(3CaO.SiO2) C3A = 2.65(Al2O3) – 1.69(Fe2O3) C4AF = 3.04(Fe2O3). Table (The Oxide Composition of a Typical Portland Cement and the Corrosponding Calculated Compound Composition) In addition to the four major compounds, there are many minor compounds formed in the kiln. The influence of these minor compounds on the properties of cement or hydrated compounds is not significant. Two of the minor compounds are of particular interest: the oxides of sodium and potassium, Na2O and K2O, known as the alkalis (although other alkalis also exist in cement). They have been found to react with some aggregates, the products of the reaction causing disintegration of the concrete, Tricalcium silicate C3S and dicalcium silicate C2S are the most important compounds responsible for the strength of hydrated cement paste. The presence of C3A in cement is undesirable, it contributes little or nothing to the strength of cement except at early ages. However, C3A is beneficial in the manufacture of cement in that it facilitates the combination of lime and silica. C4AF is also present in cement in small quantities, and , compared with the other three compounds, it does not affect the behavior significantly. However it reacts with gypsum and accelerate the hydration of silicates. 6 The actual proportions of the various compounds vary considerably from cement to cement, and indeed different types of cement are obtained by suitable proportioning of the raw materials. Hydration of Cement Anhydrous cement does not bind fine and coarse aggregate. It acquires adhesive property only when mixed with water. The chemical reactions that take place between cement and water is referred as hydration of cement. The chemistry of concrete is essentially the chemistry of the reaction between cement and water. During hydration certain products are formed. These products are important because they have cementing or adhesive value. The quality, quantity, continuity, stability and the rate of formation of the hydration products are important. 7 Heat of Hydration The reaction of cement with water is exothermic. The reaction liberates a considerable quantity of heat. This liberation of heat is called heat of hydration. Different compounds hydrate at different rates and liberate different quantities of heat. The Fig. shows the rate of hydration of pure compounds. rate of hydration of pure compounds. The hydration process is not an instantaneous one. The reaction is faster in the early period and continues idenfinitely at a decreasing rate. Complete hydration cannot be obtained under a period of one year or more. It has been observed that after 28 days of curing, cement grains have been found to have hydrated to a depth of only 4μ. It has also been observed that complete hydration under normal condition is possible only for cement particles smaller than 50μ. Calcium Silicate Hydrates During the course of reaction of C3S and C2S with water, calcium silicate hydrate, abbreviated C-S-H and calcium hydroxide, Ca(OH)2 are formed. Calcium silicate hydrates are the most important products. It is the essence that determines the good properties of concrete. It makes up 50-60 per cent of the volume of solids in a completely hydrated cement paste. The following are the approximate equations showing the reactions of C3S and C2S with water. 8 It can be seen that C3S produces a comparatively lesser quantity of calcium silicate hydrates and more quantity of Ca(OH)2 than that formed in the hydration of C2S. Ca(OH)2 is not a desirable product in the concrete mass, it is soluble in water and gets leached out making the concrete porous, particularly in hydraulic structures. Under such conditions it is useful to use cement with higher percentage of C2S content. C3S readily reacts with water and produces more heat of hydration. It is responsible for early strength of concrete. A cement with more C3S content is better for cold weather concreting. C2S hydrates rather slowly. It is responsible for the later strength of concrete. It produces less heat of hydration. development of strength of pure compounds 9 Calcium Hydroxide The other products of hydration of C3S and C2S is calcium hydroxide. It constitutes 20 to 25 per cent of the volume of solids in the hydrated paste. The lack of durability of concrete, is on account of the presence of calcium hydroxide. The calcium hydroxide also reacts with sulphates present in soils or water to form calcium sulphate which further reacts with C3A and cause deterioration of concrete. The only advantage is that Ca(OH)2, being alkaline in nature maintain pH value around 13 in the concrete which resists the corrosion of reinforcements. Structure of Hydrated Cement Concrete is generally considered as two phase material i.e., paste phase and aggregates phase. At macro level it is seen that aggregate particles are dispersed in a matrix of cement paste. At the microscopic level, the complexities of the concrete begin to show up, particularly in the vicinity of large aggregate particles. This area can be considered as a third phase, the transition zone, which represents the interfacial region between the particles of coarse aggregate and hardened cement paste. Transition zone is generally a plane of weakness and, therefore, has far greater influence on the mechanical behaviour of concrete. Due to drying shrinkage or temperature variation, the transition zone develops microcracks even before a structures is loaded. When structure is loaded and at high stress levels, these microcracks propagate and bigger cracks are formed resulting in failure of bond. Therefore, transition zone, generally the weakest link of the chain, is considered strength limiting phase in concrete. It is because of the presence of transition zone that concrete fails at considerably lower stress level than the strength of bulk paste or aggregate. Fresh cement paste is a plastic mass consisting of water and cement. With the lapse of time, say one hour, the hardening paste consists of hydrates of various compounds, unhydrated cement particles and water. With further lapse of time the quantity of unhydrated cement left in the paste decreases and the hydrates of the various compounds increase. Some of the mixing water is used up for chemical reaction, and some water occupies the gel- pores and the remaining water remains in the paste. 10 After a sufficiently long time (say a month) the hydrated paste can be considered to be consisting of about 85 to 90% of hydrates of the various compounds and 10 to 15 per cent of unhydrated cement. The mixing water is partly used up in the chemical reactions. Part of it occupies the gel-pores and the remaining water unwanted for hydration or for filling in the gel-pores causes capillary pores. These capillary pores may have been fully filled with water or partly with water or may be fully empty depending upon the age and the ambient temperature and humidity conditions. The nominal diameter of gel pores is about 3 nm while capillary pores are one or two orders of magnitude larger. Simplified model of paste structure. Solid dots represent gel particles; interstitial spaces are gel pores; spaces such as those marked C are capillary pores. Water Requirements for Hydration It has been estimated that for C3S and C2S compounds, on an average 23 % of water by weight of cement is required for chemical reaction. Thus 23 % of water chemically combines with cement and, therefore it is called bound water. A Certain Quantity of water is inadequate to fill up the gel pores, the formation of gel itself will stop and gel pores will not form. Gel water of about 15 % by weight of cement is required. Therefore, a total 23 + 15= 38 % of water by weight of cement is required for complete hydration. 11 If less than 38 % of water is used than complete hydration is not possible as the volume available is insufficient to accommodate all the product of hydration. Hence, Strength of Concrete will be reduced. If more than 38 % of water is used, then the excess of water will cause undesirable capillary cavities and concrete becomes porous. (Fig. Diagrammatic representation of the Hydration process and formation of cement gel.) Setting and Hardening of cement Setting refers to a change from a fluid to a rigid stage. Although, during setting, the paste acquires some strength, for practical purposes it is important to distinguish setting from hardening, which refers to the gain of strength of a set cement paste. Initial setting time: is regarded as the time elapsed between the moment that the water is added to the cement, to the time that the paste starts losing its plasticity. Final setting time: is the time elapsed between the moment the water is added to the cement, and the time when the paste has completely lost its plasticity and has attained sufficient firmness to resist certain definite pressure. Flash set : this Takes Place in cement with insufficient gypsum to control the rapid reaction of C3A with water. This reaction generates a considerable amount of heat causes the cement to stiffen rapidly. This can only be overcome by adding more water and re-agitating the mix. False set : False set is the name given to the abnormal stiffening of cement within a few minutes of mixing with water. It differs from flash set in that no appreciable heat is evolved, and 12 remixing the cement paste without addition of water restores plasticity of the paste until it sets in the normal manner and without a loss of strength. Fineness of Cement Because hydration starts at the surface of the cement particles, it is the total surface area of cement that represents the material available for hydration. The fineness of cement has an important influence on the rate of hydration and hence on the rate of gain of strength and also on the rate of evolution of heat. Finer cement offers a greater surface area for hydration and hence faster the development of strength. Different cements are ground to different fineness. Maximum number of particles in a sample of cement should have a size less than about 100 microns. The smallest particle may have a size of about 1.5 microns. By and large an average size of the cement particles may be taken as about 10 micron. In commercial cement it is suggested that there should be about 25-30 per cent of particles of less than 7 micron in size Soundness of Cement It is very important that the cement after setting shall not undergo any appreciable change of volume. Certain cements have been found to undergo a large expansion after setting causing disruption of the set and hardened mass. This will cause serious difficulties for the durability of structures when such cement is used. 13 Unsoundness in cement is due to 1- excess of lime (CaO) 2- excess of magnesia (MgO) 3- excessive proportion of sulphates , gypsum (CaSo4) If the addition of gypsum is more 3% to 5%. Strength of Cement Strength tests are not made on a neat cement paste because of difficulties of moulding and testing with a consequent large variability of test results. Cement–sand mortars are used for the purpose of determining the strength of cement. There are several forms of strength tests: direct tension, direct compression, and flexure. nowadays, it is the compressive strength of cement that is considered to be crucial, and it is believed that the appropriate test on cement is that on sand–cement mortar. 14 TESTING OF CEMENT (a) Field testing It is sufficient to subject the cement to field tests when it is used for minor works. The following are the field tests: (1) Open the bag and take a good look at the cement. There should not be any visible lumps. (2) The colour of the cement should normally be greenish grey. (3) Thrust your hand into the cement bag. It must give you a cool feeling. There should not be any lump inside (4) Take a pinch of cement and feel-between the fingers. It should give a smooth and not a gritty feeling. (5) Take a handful of cement and throw it on a bucket full of water, the particles should float for some time before they sink. (b) Laboratory testing. (1) Fineness test. (2) Setting time test. (3) Strength test. (4) Soundness test. (5) Heat of hydration test. (6 ) Chemical composition test. Types of Portland Cement Several types of Portland cement are available commercially, and additional Special cements can be produced for special uses. Main Types of Portland Cement 15 Typical Values of Compound Composition of Portland Cements of Different Types Ordinary Portland cement (Type I) This is by far the most common cement in use: about 90 per cent of all cement used in the United States. For every man, woman and child in the world, the consumption in 2007 was 420 kg per annum, which is second only to the consumption of water. Ordinary Portland (Type I) cement is admirably suitable for use in general concrete construction when there is no exposure to sulfates in the soil or groundwater. British Standard BS 12 : 1996 classifies Portland cements according to their compressive strength, as shown in Table. The 28-day minimum strength in MPa gives the name of the class: 32.5, 42.5, 52.5, and 62.5. Moreover, cements of class 32.5 and 42.5 are each subdivided into two subclasses, one with an ordinary early strength denoted by the letter N, the other with a high early strength. The two subclasses with a high early strength, denoted by the letter R, are rapid-hardening cements, 16 Compressive Strength Requirements of Cement According to BS 12 : 1996 Rapid-hardening Portland cement (Type III) This cement comprises Portland cement subclasses of 32.5 and 42.5 MPa as prescribed by BS EN 197-1 : 2000. Rapid-hardening Portland cement (Type III), as its name implies, develops strength more rapidly, and should, therefore, be correctly described as high early strength cement. The rate of hardening must not be confused with the rate of setting: in fact, ordinary and rapid- hardening cements have similar setting times. Rapid hardening-portland cement should not be used in mass concrete construction or in large structural sections because of its higher rate of heat development. The increased rate of gain of strength of the rapid-hardening Portland cement is achieved by : 1- a higher C3S content (higher than 55 per cent, but sometimes as high as 70 per cent) and lower C2S content. 2-by a finer grinding of the cement clinker. In practice, rapid-hardening Portland cement has a higher fineness than ordinary Portland cement. Typically, ASTM Type III cements have a specific surface, measured by the Blaine method, of 450 to 600 m2/kg, compared with 300 to 400 m2/kg for Type I cement. The higher fineness significantly increases the strength at 10 to 20 hours, The use of rapid heading cement is recommended in the following situations: (a) In pre-fabricated concrete construction. (b) Where formwork is required to be removed early for re-use elsewhere, 17 (c ) Road repair works, (d ) In cold weather concrete where the rapid rate of development of strength reduces the vulnerability of concrete to the frost damage. Low heat Portland cement (Type IV) The rise in temperature in the interior of a large concrete mass due to the heat development by the hydration of cement, can lead to serious cracking. For this reason, it is necessary to limit the rate of heat evolution of the cement used in this type of structure. Cement having this property was developed in U.S.A. during 1930 for use in mass concrete construction, such as dams, where temperature rise by the heat of hydration can become excessively large. A low-heat evolution is achieved by reducing the contents of C3S and C3A which are the compounds evolving the maximum heat of hydration and increasing C2S. (Law heat cement is made use of in construction of massive dams) modified cement (Type II) In some applications, a very low early strength may be a disadvantage, and for this reason a so called modified (Type II) cement was developed in the United States. This modified cement successfully combines a somewhat higher rate of heat development than that of low heat cement with a rate of gain of strength similar to that of ordinary Portland cement. 18 Modified cement is recommended for use in (1) structures where a moderately low heat generation is desirable or (2) where moderate sulfate attack may occur. Sulfate-resisting cement (Type V) Ordinary Portland cement is susceptible to the attack of sulphates, in particular to the action of magnesium sulphate. The salts particularly active are magnesium sulfate and sodium sulfate. Sulphates react both with the free calcium hydroxide in set cement to form calcium sulphate and with hydrate of calcium aluminate to form calcium sulphoaluminate, the volume of which is approximately 227% of the volume of the original aluminates. Their expansion within the frame work of hadened cement paste results in cracks and subsequent disruption. These reactions are known as sulfate attack. Sulfate attack is greatl accelerated if accompanied by alternating wetting and drying. Such cement with low C3A and comparatively low C4AF content is known as Sulphate Resisting Cement. ASTM C 150-09. specification limits the C3A content to 5 per cent, and also restricts the sum of the content of C4AF plus twice the C3A content to 25 per cent. The magnesia content is limited to 6 per cent. The use of sulphate resisting cement is recommended under the following conditions: (a) Concrete to be used in marine condition; (b) Concrete to be used in foundation and basement, where soil is infested with sulphates; (c) Concrete used for fabrication of pipes which are likely to be buried in marshy region or sulphate bearing soils; (d) Concrete to be used in the construction of sewage treatment works. 19 The figure shows Strength development of concrete containing 335 Kg/m3 and made with different types of portland cements. The figure Shows Development of heat of hydration of different portland cements cured at 21 C. 20 Portland blastfurnace cement Cements of this name consist of an intimate mixture of Portland cement and ground granulated blastfurnace slag. This slag is a waste product in the manufacture of pig iron, about 300 kg of slag being produced for each tonne of pig iron. Chemically, slag is a mixture of lime, silica, and alumina, that is, the same oxides that make up Portland cement but not in the same proportions. The major advantages currently recognized are: (a) Reduced heat of hydration; (b) Refinement of pore structure; (c) Reduced permeability; (d ) Increased resistance to chemical attack. White cement and pigments For architectural purposes, white or a pastel colour concrete is sometimes required. White Portland cement is made from raw materials containing very little iron oxide (less than 0.3 per cent by mass of clinker) and manganese oxide. China clay is generally used, together with chalk or limestone, free from specified impurities. Portland Pozzolana Cement Portland Pozzolana cement (PPC) is manufactured by the intergrinding of OPC clinker with 10 to 25 per cent of pozzolanic material. A pozzolanic material is essentially a silicious or aluminous material which while in itself possessing no cementitious properties, which will, in finely divided form and in the presence of water, react with calcium hydroxide, liberated in the hydration process, at ordinary temperature, to form compounds possessing cementitious properties. The pozzolanic materials generally used for manufacture of PPC is fly ash. Fly ash is a waste material, generated in the thermal power station, when powdered coal is used as a fuel. These are collected in the electrostatic precipitator. (It is called pulverised fuel ash in UK). Advantages of PPC (a) In PPC, costly clinker is replaced by cheaper pozzolanic material - Hence economical. (b) Soluble calcium hydroxide is converted into insoluble cementitious products resulting in improvement of permeability. (c) It generates reduced heat of hydration and that too at a low rate. 21 (d) PPC being finer than OPC and also due to pozzolanic action, it improves the pore size distribution and also reduces the microcracks at the transition zone. (e) The long term strength of PPC beyond a couple of months is higher than OPC if enough moisture is available for continued pozzolanic action. Other Types of Cement 1. Ordinary Portland Cement (OPC) 11. Hydrophobic Cement 2. Rapid Hardening Cement (RHC) 12. Air Entraining Cement 3. Extra-rapid Hardening Cement 13. Masonry Cement 4. Quick Setting Cement 14. Oil Well Cement 5. Low Heat Cement 15. Expansive Cement 6. Sulphate Resisting Cement 16. High Alumina Cement 7. Super Sulphated Cement 17. Concrete Sleeper Grade Cement 8. Portland Pozolana cement 18. Waterproof Cement 9. Portland slag cement 19. Rediset Cement 10. Colored cement 20. Very High Strength Cement 22