BIOL 141 Study Guide Chapter 12+13 PDF
Document Details
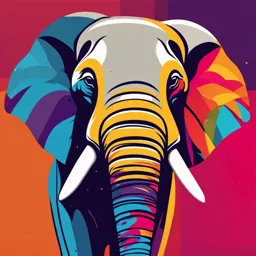
Uploaded by MatchlessConsonance
UMBC
Tags
Summary
This document is a study guide for a biology course, presenting detailed information on topics such as chromosomes, the cell cycle, mitosis, and meiosis. It includes definitions, diagrams, and questions related to these topics.
Full Transcript
BIOL 141 Study Guide - Chapter 12+13 1. What is a chromosome? What is a chromatid? How many DNA double helices are present in an unreplicated chromosome or a replicated chromosome? How do sister chromatids differ from homologous chromosomes? 1. Chromosome: A chromosome is a single, long...
BIOL 141 Study Guide - Chapter 12+13 1. What is a chromosome? What is a chromatid? How many DNA double helices are present in an unreplicated chromosome or a replicated chromosome? How do sister chromatids differ from homologous chromosomes? 1. Chromosome: A chromosome is a single, long DNA molecule, which contains many genes, and is associated with proteins to help organize its structure. Chromosomes are found in the nucleus of eukaryotic cells and are visible under a microscope during cell division. 2. Chromatid: A chromatid is one half of a duplicated chromosome. After DNA replication, a chromosome consists of two identical sister chromatids joined together at the centromere. 3. DNA Double Helices in Chromosomes: ◦ Unreplicated Chromosome: Contains one DNA double helix. ◦ Replicated Chromosome: Contains two DNA double helices, one in each sister chromatid. 4. Sister Chromatids vs. Homologous Chromosomes: ◦ Sister Chromatids: These are two identical copies of a single chromosome, connected by a centromere. They result from DNA replication and are identical in genetic information. ◦ Homologous Chromosomes: These are pairs of chromosomes (one from each parent) that are similar in shape, size, and genetic content, but may have different alleles for the same genes. They are not identical copies, unlike sister chromatids. 2. Draw a diagram showing the phases of the cell cycle. Describe the key events that occur during each stage. G1 Phase (Gap 1): The cell grows, synthesizes proteins, and performs its routine metabolic functions. It also checks for DNA damage and prepares for DNA replication. S Phase (Synthesis): DNA replication occurs, creating two identical copies (sister chromatids) of each chromosome. The cell also duplicates its centrosomes. G2 Phase (Gap 2): The cell continues to grow, prepares for mitosis, and checks for any errors in DNA replication. M Phase (Mitosis): The cell divides the duplicated chromosomes evenly, producing two nuclei in preparation for full division. Cytokinesis: The cytoplasm divides, resulting in two distinct daughter cells, each with a full set of chromosomes. 3. When during the cell cycle are chromosomes duplicated? If a diploid cell has 8 chromosomes during G1, how many DNA double helices will be present in G1? in G2? in metaphase? in each daughter cell after cell division is complete? When are chromosomes duplicated? Chromosomes are duplicated during the S phase (Synthesis phase) of the cell cycle. This is when DNA replication occurs, producing two identical sister chromatids for each chromosome. If a diploid cell has 8 chromosomes during G1: In G1: There are 8 chromosomes, each with a single DNA double helix. Therefore, there are 8 DNA double helices in total. In G2: Each chromosome has been duplicated to form two sister chromatids, so there are 8 chromosomes, each with two DNA double helices, resulting in 16 DNA double helices. In Metaphase: The chromosomes are still duplicated and aligned in the middle of the cell, so there are still 16 DNA double helices (each chromosome with two sister chromatids). After Cell Division (in each daughter cell): Following cytokinesis, each daughter cell receives 8 chromosomes, with each chromosome consisting of a single DNA double helix. Thus, each daughter cell has 8 DNA double helices. 4. Draw a diagram showing the steps of mitosis. Describe how sister chromatids are physically separated during the cell cycle, allowing each daughter cell to inherit a complement of chromosomes identical to the parent cell. What cell structures are required for this process? Steps of Mitosis: 1. Prophase: Chromosomes condense and become visible. The nuclear membrane begins to dissolve, and spindle bers start forming, extending from the centrosomes toward the chromosomes. 2. Prometaphase: The nuclear envelope fully dissolves, allowing spindle bers to access chromosomes. Each chromosome forms kinetochores at its centromere, and spindle bers attach to these kinetochores, starting to move chromosomes toward the cell's center. 3. Metaphase: Chromosomes line up at the cell’s equatorial plane (metaphase plate). Spindle bers attach to the centromeres of each chromosome via the kinetochores, preparing for chromatid separation. fi fi fi fi 4. Anaphase: Spindle bers shorten, pulling sister chromatids apart toward opposite poles of the cell. This separation ensures each future daughter cell will inherit an identical set of chromosomes. This image shows how chromosomes are moved during anaphase using microtubules and the kinetochore structure. Here’s what you need to know based on the key components: 1. Kinetochore: ◦ The kinetochore (represented by black and green structures) is a complex of proteins attached to the chromosome at the centromere. It links the chromosome to the microtubule bers, allowing them to pull the chromosome during cell division. ◦ In the image, the kinetochore has plates (the darker parts) that connect to the chromosome and bers extending outward to interact with the microtubules. 2. Microtubules: ◦ The microtubules are long, tube-like structures made of tubulin subunits (the orange spheres). They are polarized, with a plus end where tubulin subunits can be added or removed. fi fi fi ◦ During anaphase, microtubules are anchored at the minus end (toward the spindle pole) and begin to disassemble at the plus end (near the kinetochore), causing them to "shorten" and pull the chromosome toward the poles. 3. Green Ring Structure: ◦ The green ring structure helps tether the chromosome to the microtubule, holding it in place as the microtubule shortens. ◦ As tubulin subunits are removed from the plus end, the ring allows the chromosome to slide along the microtubule toward the spindle pole, which generates the force to move the chromosome. 4. Chromosome Movement: ◦ As the microtubule disassembles at the kinetochore (plus end), it "reels in" the chromosome, pulling it closer to the spindle pole. ◦ This disassembly process creates the force needed to separate sister chromatids and ensures each daughter cell will get one copy of each chromosome. In summary: The kinetochore attaches the chromosome to the spindle bers. The microtubules shorten at the plus end to pull chromosomes toward opposite poles. The green ring structure allows the chromosome to move smoothly along the microtubule as it disassembles. 5. Telophase: Chromatids reach opposite poles, and new nuclear membranes form around each set of chromosomes. The chromosomes begin to de-condense, and the cell is nearly ready to divide. Key Structures Required: Mitotic Spindle: Network of microtubules responsible for moving and segregating the chromosomes. Centrosomes: Organize spindle bers that attach to chromosomes and facilitate their separation. Kinetochores: Protein complexes at each chromosome's centromere where spindle bers attach, ensuring accurate chromosome alignment and separation. Each daughter cell inherits an identical chromosome set to that of the parent cell through these coordinated steps and structures. 5. What happens during cytokinesis? Explain the role of the cytoskeleton in this process. fi fi fi During cytokinesis, the cytoplasm of a single cell divides to form two distinct daughter cells, each with a complete set of chromosomes and organelles. This process typically begins in late anaphase or telophase and completes after mitosis. Cytokinesis differs between animal and plant cells: In Animal Cells: A contractile ring made of actin laments (part of the cytoskeleton) forms just beneath the cell membrane. As the actin ring contracts, it pinches the cell membrane inward, creating a "cleavage furrow" that deepens until the cell is split in two. In Plant Cells: Due to the rigid cell wall, plant cells cannot form a cleavage furrow. Instead, vesicles carrying cell wall materials gather in the center of the cell, forming a cell plate. This cell plate expands outward, eventually fusing with the cell membrane to divide the cell. Role of the Cytoskeleton in Cytokinesis The cytoskeleton plays a critical role in cytokinesis by providing the structural framework and force necessary for cell division: Actin Filaments: In animal cells, actin laments form the contractile ring, which pulls the cell membrane inward to create the cleavage furrow. Microtubules: During plant cell cytokinesis, microtubules guide vesicles to the center of the cell to form the cell plate, ensuring proper distribution of cell wall materials. The cytoskeleton’s coordinated actions ensure that cytokinesis successfully divides the parent cell into two genetically identical daughter cells. fi fi 6. What is MPF, and what are its components? How do the concentration of these components vary across the cell cycle? How does MPF act as a trigger for initiating M-Phase? What are the regulatory steps required for MPF activation? What causes the MPF concentration to decline sharply during M-Phase? M-Phase Promoting Factor (MPF) is a protein complex that acts as a key regulator, triggering the start of M-phase (mitosis) in the cell cycle. Here’s a breakdown of MPF and its role: 1. Components of MPF MPF is made up of two main components: Cyclin: A regulatory protein that controls the cell cycle's progression. The speci c cyclin involved in MPF is cyclin B. Cyclin-Dependent Kinase (Cdk): An enzyme that, when bound to cyclin, can phosphorylate other proteins to drive the cell cycle forward. The Cdk in MPF is Cdk1. 2. Concentration Changes Across the Cell Cycle Cyclin levels uctuate during the cell cycle, gradually increasing throughout interphase (G1, S, and G2 phases) and peaking at the beginning of M-phase. Cdk levels, on the other hand, remain constant but are only active when bound to cyclin. 3. How MPF Triggers M-Phase When cyclin levels are high enough in G2, cyclin binds to Cdk1 to form active MPF. Active MPF triggers a cascade of phosphorylation events, which: ◦ Leads to the breakdown of the nuclear envelope. ◦ Initiates chromosome condensation. ◦ Promotes the assembly of the mitotic spindle. These events together push the cell into M-phase, where mitosis occurs. 4. Regulatory Steps for MPF Activation Cyclin Binding: Cdk1 becomes active only when bound to cyclin. Phosphorylation and Dephosphorylation: Cdk1 is initially phosphorylated at two sites (an activating and an inhibitory site). Before M-phase begins, a phosphatase enzyme removes the inhibitory phosphate, fully activating MPF. Positive Feedback Loop: Active MPF can further activate phosphatases, amplifying its own activation and ensuring a strong transition into M-phase. 5. Decline of MPF Concentration During M-Phase During late M-phase, cyclin B is targeted for degradation by a protein complex called the anaphase-promoting complex (APC). The APC tags cyclin with ubiquitin, marking it for destruction by the proteasome. fl fi As cyclin degrades, Cdk1 loses its cyclin partner, inactivating MPF and leading to a sharp decline in MPF levels. This decline is necessary for the cell to exit mitosis and re-enter interphase. In summary: MPF is composed of cyclin B and Cdk1. Cyclin levels rise and fall through the cycle, while Cdk1 remains constant but only becomes active with cyclin binding. MPF initiates M-phase by triggering key mitotic events. MPF is regulated by phosphorylation and feedback mechanisms. MPF concentration declines in M-phase as cyclin B is degraded, allowing the cell to exit mitosis. 7. What category of proteins transfer a phosphate group to substrates? Give an example of one such protein that plays a role in cell cycle regulation. The category of proteins that transfer a phosphate group to substrates is called kinases. An example of a kinase that plays a crucial role in cell cycle regulation is the Cyclin-dependent kinase (Cdk). Cdk, when bound to its regulatory partner cyclin, becomes active and can phosphorylate other proteins, such as Rb (retinoblastoma protein). This phosphorylation of Rb releases E2F, allowing the cell to progress from the G1 phase into the S phase, where DNA replication occurs. 8. What are cell cycle checkpoints? Where in the cell cycle are the checkpoints found? How do they differ? How are Rb, p53, and MPF involved in cell cycle checkpoints? What events could lead to cell cycle arrest? Cell Cycle Checkpoints are control points in the cell cycle that ensure each phase is completed correctly before moving to the next phase. These checkpoints prevent damaged or incomplete cells from dividing, helping to maintain genetic stability. Major Cell Cycle Checkpoints: 1. G1 Checkpoint (Restriction Point): ◦ Location: At the end of G1, before entering S phase. ◦ Purpose: Ensures the cell has enough resources, checks for DNA damage, and con rms readiness for DNA replication. ◦ Key Players: ▪ Rb (Retinoblastoma protein): Rb binds to E2F, a protein needed for S- phase entry. Phosphorylation by Cdk (Cyclin-dependent kinase) inactivates Rb, releasing E2F and allowing S-phase entry. ▪ p53: If DNA damage is detected, p53 can halt the cycle by activating p21, which blocks Cdk activity, preventing Rb phosphorylation and stopping progression to S phase. 2. G2 Checkpoint: ◦ Location: At the end of G2, before entering mitosis. ◦ Purpose: Checks that DNA replication is complete and accurate, and ensures the cell is ready for mitosis. ◦ Key Player: ▪ MPF (M-phase Promoting Factor): MPF, a complex of cyclin B and Cdk1, drives the cell into mitosis. MPF is regulated by phosphorylation; only active MPF can push the cell into M-phase. If errors are detected, MPF activation is blocked, and the cell is arrested in G2. 3. M (Mitosis) Checkpoint (Spindle Checkpoint): ◦ Location: During mitosis, speci cally at metaphase. ◦ Purpose: Ensures all chromosomes are properly attached to spindle bers before anaphase. This prevents incorrect chromosome separation. ◦ Key Mechanism: Proteins like Mad2 and BubR1 inhibit the anaphase-promoting complex (APC) if chromosomes are not correctly attached, preventing premature separation. 4. G0 Phase: ◦ Purpose: G0 is not a checkpoint but a resting phase for cells that are not actively dividing. Some cells enter G0 temporarily, while others (like nerve cells) stay there permanently. Cells in G0 can re-enter the cycle if needed. How Rb, p53, and MPF Are Involved: fi fi fi Rb: Acts as a gatekeeper at the G1 checkpoint by controlling E2F. If Rb is phosphorylated by Cdk, it releases E2F, allowing entry into S phase. p53: Known as the "guardian of the genome," p53 can stop the cell cycle at G1 or G2 if DNA damage is detected. It activates proteins that prevent Cdk activation, stopping the cycle for repairs. MPF: Controls the G2-to-M transition, ensuring the cell is fully prepared to enter mitosis. MPF is activated when cyclin B binds Cdk1 and speci c phosphates are removed. Events That Could Lead to Cell Cycle Arrest: DNA Damage: Activates p53, which can halt the cell cycle for repair or trigger apoptosis if the damage is irreparable. Insuf cient Resources or Signals: At the G1 checkpoint, if conditions are not favorable, the cell may pause or enter G0. Incomplete DNA Replication: Prevents progression at the G2 checkpoint until replication is complete. Improper Chromosome Attachment: Stops cell division at the M checkpoint to avoid uneven chromosome distribution. These checkpoints and their associated proteins work together to maintain healthy cell division and prevent the development of cancer by stopping cells with errors from continuing to divide. fi fi 9. Explain the cellular basis of cancer. What defects are commonly found in cancer cell? Do all cancer cells have mutations in the same genes? Explain. The Cellular Basis of Cancer: Cancer results from uncontrolled cell division due to a breakdown in the regulatory mechanisms that typically govern the cell cycle. In normal cells, strict controls ensure that division only occurs when conditions are right and in response to signals from surrounding cells. This coordination, called social control, prevents cells from dividing unless it’s bene cial for the organism as a whole. Cancerous cells ignore these signals, leading to abnormal growth and division. Key Defects in Cancer Cells: 1. Failure of Cell Cycle Checkpoints: ◦ Cancer cells often bypass important checkpoints, particularly the G1 checkpoint, which regulates entry into the DNA replication (S) phase. This failure can result from defects in proteins that enforce the checkpoint, allowing cells to divide without being checked for errors. 2. Overactivation of Growth Signals (Oncogenes): ◦ Cancer cells have mutations in proto-oncogenes that turn them into oncogenes, which are permanently "on" and drive cell growth. For example, Ras is a protein that normally responds to growth signals to trigger cell division. In cancer, Ras can be mutated to remain active, continuously signaling the cell to divide. 3. Loss of Tumor Suppressors: ◦ Tumor suppressor genes, like p53 and Rb (Retinoblastoma protein), normally act as brakes to stop cell division when DNA is damaged. In many cancers, these genes are either mutated or inactivated, removing the cell’s ability to halt division and repair damage. ◦ p53 is crucial for DNA damage response; without functional p53, cells with damaged DNA proceed to divide, accumulating more mutations. ◦ Rb controls the G1 checkpoint by binding to E2F, a protein that drives the cell into S phase. If Rb is defective or overly phosphorylated due to high cyclin-Cdk activity, it cannot restrain E2F, leading to unregulated cell division. 4. Loss of Social Control: ◦ Cancer cells often produce their own growth signals or ignore the need for external growth factors, allowing them to grow without the social control signals that normally restrict cell division to the needs of the organism. 5. Metastasis: fi ◦ Cancer cells become invasive, detaching from their original location, traveling through the bloodstream or lymphatic system, and forming secondary tumors in new areas. This spreading, or metastasis, is what makes malignant cancers particularly dangerous. Do All Cancer Cells Have Mutations in the Same Genes? No, not all cancer cells have mutations in the same genes. Cancer is a highly diverse set of diseases, and while some genes (like p53 or Rb) are frequently mutated in various types of cancer, the exact mutations can vary signi cantly. Different types of cancer often have unique mutation patterns based on the tissue they arise from, the speci c triggers (like environmental exposure or inherited genetic predispositions), and other cellular conditions. For example: BRCA1 and BRCA2 mutations are often seen in breast and ovarian cancers but are not common in other types. KRAS mutations are common in colon and lung cancers. Summary Cancer arises when cells lose control over the cell cycle due to mutations that (1) activate growth-promoting proteins (oncogenes) or (2) disable tumor suppressor proteins. These defects allow cancer cells to bypass normal regulatory checkpoints, avoid apoptosis, and proliferate without social control. Because cancer can involve mutations in various genes, and not all cancers share the same mutations, each type of cancer may require a different approach to treatment. Understanding the molecular basis of these defects has greatly improved cancer detection and treatment options, though there is no universal cure for all cancers. 10. What are growth factors, and what role do they play in the control (or loss of control) of the cell cycle? What is the relationship of cancer to the G1 checkpoint? Growth Factors and Their Role in the Cell Cycle: Growth factors are signaling molecules, usually proteins or polypeptides, released by certain cells to stimulate growth and division in nearby cells. In multicellular organisms, cells respond to these growth factors to know when to divide, ensuring cell division occurs only when it’s needed for growth, repair, or maintenance. Role of Growth Factors in Cell Cycle Control: Social Control: Growth factors serve as a form of social control over the cell cycle, particularly at the G1 checkpoint. When growth factors bind to receptors on the cell surface, they activate intracellular signaling pathways that lead to the production of regulatory proteins, such as cyclins and E2F. Progression Through the G1 Checkpoint: One critical effect of growth factors is the stimulation of the G1 checkpoint. This checkpoint decides whether the cell will enter S fi fi phase (DNA synthesis) or remain in G1. When growth factors are present, they activate cyclin-Cdk complexes, which phosphorylate Rb (Retinoblastoma protein). Phosphorylated Rb releases E2F, a transcription factor that activates genes needed for S phase, pushing the cell to proceed with DNA replication and division. Loss of Control and Cancer: Cancer is closely related to a loss of control over the G1 checkpoint, often due to defects in the pathways that normally respond to growth factors. Here’s how it happens: 1. Overproduction of Growth Factors or Oncogene Activation: ◦ Cancer cells may overproduce growth factors themselves or mutate genes (proto- oncogenes) involved in growth factor signaling pathways, turning them into oncogenes. For example, mutations in Ras, a protein involved in growth factor signaling, can keep it permanently active, continuously signaling the cell to divide even in the absence of external growth factors. 2. Disruption of Rb Function: ◦ In cancer, Rb protein is often defective or excessively phosphorylated due to high levels of cyclin-Cdk activity. This prevents Rb from binding to E2F, leading to uncontrolled cell cycle progression through G1, even without the proper growth signals. 3. Mutation in Tumor Suppressors like p53: ◦ p53 is another checkpoint regulator that can stop the cell cycle if DNA damage is detected. Mutations in p53 prevent this halt, allowing cells with damaged DNA to continue dividing. When p53 is nonfunctional, cells skip this critical safety check, contributing to tumor formation and growth. Summary Growth factors are essential for controlling cell division by promoting progression through the G1 checkpoint. In cancer, this control is often lost due to mutations in genes that respond to or regulate growth factor signaling, particularly at the G1 checkpoint. Overactive growth signaling, defective Rb, or nonfunctional p53 are common in cancer cells, leading to unchecked cell division and tumor formation. This breakdown in cell cycle control is a key factor in cancer’s development and progression. BIOL 141 Study Guide - Chapter 13 1. Compare and contrast meiosis and mitosis. Consider the following factors: Number of times DNA is replicated Number of cell divisions Number of daughter cells produced “Ploidy” of daughter cells Type of cells in which the process occurs Number and genetic make-up of chromosomes in parent cell compared to daughter cells Here's a comparison between meiosis and mitosis based on the factors you provided: 1. Number of Times DNA is Replicated Mitosis: DNA is replicated once, during the S phase of the cell cycle, prior to mitosis. Meiosis: DNA is also replicated once, during the S phase, before the rst meiotic division. However, meiosis includes two cell divisions afterward. 2. Number of Cell Divisions Mitosis: One cell division occurs, resulting in two daughter cells. Meiosis: Two cell divisions occur (Meiosis I and Meiosis II), resulting in four daughter cells. 3. Number of Daughter Cells Produced Mitosis: Produces two daughter cells. Meiosis: Produces four daughter cells. 4. Ploidy of Daughter Cells Mitosis: Produces diploid (2n) cells, maintaining the same ploidy level as the parent cell. Meiosis: Produces haploid (n) cells, reducing the ploidy level by half compared to the parent cell. fi 5. Type of Cells in Which the Process Occurs Mitosis: Occurs in somatic (body) cells, enabling growth, repair, and asexual reproduction. Meiosis: Occurs in germ cells to produce gametes (sperm and eggs) for sexual reproduction. 6. Number and Genetic Make-up of Chromosomes in Parent Cell Compared to Daughter Cells Mitosis: The daughter cells have the same number and genetic make-up of chromosomes as the parent cell. They are genetically identical to the parent cell and to each other (assuming no mutations). Meiosis: The daughter cells have half the number of chromosomes compared to the parent cell. They are genetically distinct from the parent cell and from each other due to processes like crossing over and independent assortment. In summary, mitosis produces two identical diploid cells and supports growth and maintenance, while meiosis produces four diverse haploid cells necessary for sexual reproduction. 2. What is the difference between diploid and haploid cells? What cells in the body are diploid and which are haploid? What kind of diploid cells can become haploid cells? The terms somatic and germ line should be included in your answer. The terms diploid and haploid refer to the number of chromosome sets in a cell. Difference Between Diploid and Haploid Cells Diploid (2n) cells contain two sets of chromosomes—one set inherited from each parent. In humans, diploid cells have 46 chromosomes in total (23 pairs). Haploid (n) cells contain only one set of chromosomes. In humans, haploid cells have 23 chromosomes. Types of Cells in the Body Diploid Cells: Most of the body’s cells, known as somatic cells (e.g., skin, muscle, and liver cells), are diploid. These cells undergo mitosis to create identical diploid cells for growth, repair, and maintenance. Haploid Cells: Only gametes (sperm and egg cells) are haploid. These cells are produced through meiosis, which reduces the chromosome number by half to prepare for sexual reproduction. Transformation from Diploid to Haploid Germ Line Cells: Certain specialized diploid cells in the germ line can become haploid cells. Germ line cells are precursor cells located in the ovaries and testes that undergo meiosis to produce haploid gametes. This reduction is essential for maintaining a stable chromosome number across generations when fertilization occurs (sperm and egg combine to form a diploid zygote). In summary, somatic cells are diploid and make up the body, while germ line cells are diploid cells that undergo meiosis to become haploid gametes for reproduction. 3. Relate the concept of ploidy to chromosome numbers in specific organisms. For example: If a particular species of plant is made up of cells that each have 30 separate chromosomes, 15 of which appear to be paired in terms of size and shape, what is the “ploidy” of this plant? What is the term for these similar chromosomes? What is the ploidy of a different plant species in which the cells also have 30 separate chromosomes, but there seem to be 10 triplets of similar size and shape? The concept of ploidy refers to the number of sets of chromosomes in a cell. Chromosomes that are similar in size, shape, and genetic content are known as homologous chromosomes. Let’s apply these ideas to the two plant species you mentioned: First Plant Species If a plant has 30 separate chromosomes, with 15 pairs of chromosomes that appear similar in size and shape, this suggests that the plant is diploid (2n). In this case, each chromosome has a homologous partner, making a total of 15 pairs. These similar chromosomes are called homologous chromosomes. Therefore, the ploidy of this plant is diploid (2n = 30). Second Plant Species For the second plant species, the cells have 30 separate chromosomes, but they are arranged in 10 sets of triplets of similar size and shape. This con guration suggests that the plant is triploid (3n) because each chromosome type has three homologous copies instead of two. In this case, the ploidy of this plant is triploid (3n = 30). In summary: The rst plant is diploid (2n = 30), with 15 pairs of homologous chromosomes. The second plant is triploid (3n = 30), with 10 triplets of homologous chromosomes. 4. How many different alleles for the same characteristic can be present in a population? How many different alleles for the same characteristic can be present in a diploid individual? fi fi In a population, there can be many different alleles for the same characteristic. For example, in humans, the gene for eye color can have multiple alleles across the population (e.g., alleles for brown, blue, green, and hazel eyes). The diversity of alleles in a population depends on factors such as mutation, genetic recombination, and natural selection, and the number of possible alleles is generally unrestricted. In a diploid individual, however, there can only be two alleles for any given gene or characteristic—one allele inherited from each parent. This is because diploid organisms have two sets of chromosomes (one set from each parent), which means they have two copies of each gene. Therefore, while a population can carry many different alleles for the same characteristic, a diploid individual can carry at most two alleles for that characteristic. 5. Draw cells in metaphase of mitosis, metaphase I of meiosis, and metaphase II of meiosis. What is different between the chromosomes in your three drawing? 1. Metaphase of Mitosis: ◦ In mitosis, chromosomes are aligned individually along the metaphase plate. ◦ Each chromosome consists of two sister chromatids joined at a centromere. ◦ The chromatids are identical and will be pulled apart to opposite poles, resulting in two identical daughter cells. 2. Metaphase I of Meiosis: ◦ In metaphase I, homologous chromosomes (each consisting of two sister chromatids) are paired and aligned along the metaphase plate. ◦ This stage is characterized by the presence of tetrads (pairs of homologous chromosomes), where each homolog contains two chromatids. ◦ These homologous pairs will be separated, with each resulting daughter cell receiving one chromosome from each pair, which contributes to genetic diversity. 3. Metaphase II of Meiosis: ◦ In metaphase II, chromosomes are aligned individually (like in mitosis) along the metaphase plate. ◦ However, each chromosome still consists of two sister chromatids because there was no further replication after meiosis I. ◦ These chromatids will separate in anaphase II, resulting in four genetically unique haploid cells by the end of meiosis. Summary of Differences: Mitosis Metaphase: Single chromosomes line up independently. Meiosis Metaphase I: Homologous chromosomes pair up (tetrads). Meiosis Metaphase II: Single chromosomes (with two chromatids) line up, similar to mitosis, but each chromosome is now in a haploid cell. 6. What happens during anaphase in mitosis, meiosis I, and meiosis II? What happens if the steps you just described do not occur correctly during meiosis? State the term for this sort of meiotic error and the term that describes the resulting daughter cells. During anaphase, chromosomes are separated and pulled to opposite poles of the cell. Here’s what occurs during anaphase in each process and the consequences if these steps go wrong in meiosis: Anaphase in Mitosis In mitosis anaphase, the sister chromatids of each chromosome are separated at the centromere and pulled to opposite poles of the cell by the spindle bers. This separation ensures that each daughter cell receives an identical set of chromosomes. Anaphase I in Meiosis In meiosis I anaphase, the homologous chromosomes (each consisting of two sister chromatids) are pulled apart to opposite poles. Unlike mitosis, the sister chromatids are not separated here; instead, each homologous pair is split so that one chromosome from each pair goes to each daughter cell. This reductional division reduces the chromosome number by half, resulting in haploid cells. Anaphase II in Meiosis In meiosis II anaphase, the sister chromatids of each chromosome are nally separated and pulled to opposite poles, similar to anaphase in mitosis. This step results in four genetically unique haploid cells by the end of meiosis II. Consequences of Errors During Anaphase in Meiosis If chromosomes do not separate correctly during anaphase in meiosis, it leads to a type of meiotic error called nondisjunction. Nondisjunction can occur either during: Anaphase I, when homologous chromosomes fail to separate, or Anaphase II, when sister chromatids fail to separate. Resulting Daughter Cells from Nondisjunction When nondisjunction occurs, it results in daughter cells with an abnormal number of chromosomes, a condition known as aneuploidy. Some cells may have an extra chromosome (trisomy) or may lack a chromosome (monosomy). This can lead to genetic disorders. For example, trisomy 21 (an extra chromosome 21) causes Down syndrome. 7. What happens during crossing over? When does crossing over occur? How does crossing over influence the genetic diversity of gametes? fi fi Crossing over is a process in which homologous chromosomes exchange segments of genetic material. Here’s a breakdown of how it occurs and its effects: What Happens During Crossing Over? During crossing over, non-sister chromatids from homologous chromosomes align closely at points called chiasmata. At these chiasmata, the chromatids break and exchange corresponding segments of DNA. This results in new combinations of alleles on each chromatid. When Does Crossing Over Occur? Crossing over occurs during prophase I of meiosis. Speci cally, it happens when homologous chromosomes pair up in a process called synapsis before being separated in anaphase I. How Does Crossing Over In uence Genetic Diversity? Crossing over creates new combinations of alleles on each chromatid, which leads to genetic recombination. As a result, each gamete receives a unique set of genetic information. This increased variability means that offspring inherit a mixture of traits, not just direct copies of either parent's chromosome arrangements. In summary, crossing over during meiosis increases genetic diversity by mixing alleles between homologous chromosomes, leading to a greater variety of possible genetic combinations in gametes. 8. What is independent assortment? Explain which step(s) in meiosis lead to independent assortment. Sketch the genetically distinct daughter cells that could result from independent assortment if a diploid parent cell (2n=6) undergoes meiosis. fi fl Independent assortment is a process during meiosis in which homologous chromosome pairs are distributed randomly into daughter cells. This randomness leads to different combinations of maternal and paternal chromosomes in each gamete, contributing to genetic diversity. Steps in Meiosis Leading to Independent Assortment Independent assortment occurs during metaphase I of meiosis, when homologous chromosome pairs (tetrads) line up at the metaphase plate. Each pair can align with the maternal or paternal chromosome on either side of the plate, and this alignment is independent for each pair. When these pairs are separated during anaphase I, the chromosomes are distributed randomly to the daughter cells. llustration of Independent Assortment with a Diploid Parent Cell (2n = 6) If we have a diploid parent cell with 2n = 6 chromosomes (meaning there are 3 pairs of homologous chromosomes), independent assortment allows for multiple possible arrangements. Each chromosome pair can align in two ways, so for three pairs, there are 8 possible combinations of gametes. 9. Is self-fertilization likely to result in offspring that are genetically identical to the parent? to each other? Explain why or why not. Self-fertilization is not likely to produce offspring that are genetically identical to the parent or to each other. Here’s why: 1. Genetic Variation Through Meiosis: ◦ Even in self-fertilization, meiosis occurs to produce gametes (e.g., sperm and egg in plants or hermaphroditic animals). ◦ Meiosis includes processes like crossing over and independent assortment, which create genetic variation by shuf ing alleles and producing unique combinations of chromosomes in each gamete. 2. Fusion of Gametes: ◦ In self-fertilization, two genetically distinct gametes (produced by meiosis) from the same individual combine. Because each gamete is genetically unique, the resulting offspring inherit a new combination of alleles. ◦ This means the offspring will not be genetically identical to the parent, even though both gametes came from the same individual. 3. Offspring Diversity: ◦ Each offspring receives a different combination of alleles due to the random nature of meiosis, meaning siblings will also not be genetically identical to each other (unless a mutation causes uniformity, which is rare). In summary, self-fertilization produces genetically unique offspring, both in comparison to the parent and to each other, due to genetic recombination and random assortment during meiosis. However, self-fertilization does result in less genetic diversity than cross-fertilization because all genetic material originates from the same individual. fl