Anticoagulants: A Review of History, Mechanism, and Indications PDF
Document Details
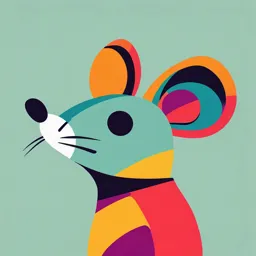
Uploaded by BeneficiarySetting
2022
Marco Heestermans, Géraldine Poenou, Hind Hamzeh-Cognasse, Fabrice Cognasse, and Laurent Bertoletti
Tags
Summary
This review article provides a short history of anticoagulants, including their mechanisms of action, pharmacology, and indications. It covers the evolution of anticoagulants from early discoveries to modern therapies and discusses the challenges in using new anticoagulants in various patient populations. The review emphasizes the importance of understanding the pharmacological properties of different anticoagulants for optimized medical care.
Full Transcript
cells Review Anticoagulants: A Short History, Their Mechanism of Action, Pharmacology, and Indications Marco Heestermans 1,2 , Géraldine Poenou 2,3 , Hind Hamzeh-Cognasse 2 , Fabrice Cognasse 1,2 and Laurent Bertoletti 2,3,4, * 1 2 3 4 * Citation: Heestermans, M.; Poenou, French Blood Establishment...
cells Review Anticoagulants: A Short History, Their Mechanism of Action, Pharmacology, and Indications Marco Heestermans 1,2 , Géraldine Poenou 2,3 , Hind Hamzeh-Cognasse 2 , Fabrice Cognasse 1,2 and Laurent Bertoletti 2,3,4, * 1 2 3 4 * Citation: Heestermans, M.; Poenou, French Blood Establishment (EFS) Auvergne Rhône Alpes-Scientific Department, F-42270 Saint-Etienne, France INSERM, U1059, SAINBIOSE, Jean Monnet University, F-42023 Saint-Etienne, France Service de Médecine Vasculaire et Thérapeutique, CHU de St-Etienne, F-42055 Saint-Etienne, France INSERM, CIC-1408, CHU Saint-Etienne, F-42055 Saint-Etienne, France Correspondence: [email protected] Abstract: Anticoagulant drugs antagonize coagulation and are used to prevent or cure (recurrent) venous thromboembolism (VTE). Drugs to prevent clotting have been used for more than a century, and, nowadays, physicians possess a broad panel of multiple anticoagulants to meet the individual needs of a patient. Within this review, we aimed to revise the history of the different anticoagulants that are currently prescribed in the clinic. In addition, we compared their pharmacological properties, medical indications, and the difficulties in implementing new anticoagulants in vulnerable patient populations. Since the introduction of unfractionated heparin in the 1930s, major advances in the mechanistic understanding and the medical use of anticoagulants have allowed for significant improvements to treat VTE patients. However, a new generation of anticoagulants is currently being tested in clinical trials, with the goal of further optimizing medical care. G.; Hamzeh-Cognasse, H.; Cognasse, F.; Bertoletti, L. Anticoagulants: A Short History, Their Mechanism of Keywords: venous thromboembolism; anticoagulant drugs; heparin; vitamin K antagonists; DOACs; pharmacology; drug–drug interactions; novel anticoagulants Action, Pharmacology, and Indications. Cells 2022, 11, 3214. https://doi.org/10.3390/ cells11203214 1. Introduction Academic Editors: Pierpaolo Di Thrombosis is the leading cause of death worldwide, and, in terms of prevalence, the first among the non-contagious diseases for which the WHO aims to reduce the incidence (https://www.who.int/news-room/fact-sheets/detail/noncommunicable-diseases (accessed on 1 September 2022)). The disease is a result of vascular occlusion and, in clinics, is mainly manifested by myocardial infarction (5 per 1000 individuals per year) , stroke (1.3–4.1 per 1000 individuals per year) , or venous thromboembolism (VTE, 1–3 per 1000 individuals per year). Antiplatelet drugs are mostly used to treat arterial thrombotic events such as myocardial infarction and stroke, while VTE is prevented and cured using anticoagulants. As the name already implies, anticoagulants antagonize coagulation, i.e., secondary hemostasis. Hemostasis is the physiological process where, in healthy individuals, vascular disruptions and bleedings as a result of an external aggression are stopped via the formation of a blood clot. In a pathological setting, coagulation drives the formation of undesired intravascular blood clots, which are referred to as thrombi. The first anticoagulant drugs to prevent VTE, unfractionated heparin and warfarin, were identified by serendipity in the early 20th century [6,7]. In the following years, these drugs have been optimized to prevent or treat VTE more efficiently. More recently, a novel generation of anticoagulant drugs has been introduced, which are designed to target coagulation factor IIa (thrombin) and coagulation factor Xa (FXa), two enzymes that are crucial for coagulation [8,9]. Depending on the medical indication, nowadays, physicians can prescribe different anticoagulant drugs that are best-suited for the needs of their patients. However, although anticoagulant drugs are effective in preventing VTE, they Micco, Vincenzo Russo and Egidio Imbalzano Received: 9 September 2022 Accepted: 12 October 2022 Published: 13 October 2022 Publisher’s Note: MDPI stays neutral with regard to jurisdictional claims in published maps and institutional affiliations. Copyright: © 2022 by the authors. Licensee MDPI, Basel, Switzerland. This article is an open access article distributed under the terms and conditions of the Creative Commons Attribution (CC BY) license (https:// creativecommons.org/licenses/by/ 4.0/). Cells 2022, 11, 3214. https://doi.org/10.3390/cells11203214 https://www.mdpi.com/journal/cells Cells 2022, 11, 3214 Cells 2022, 11, 3214 2 of 17 are crucial for coagulation [8,9]. Depending on the medical indication, nowadays, physi2 of 17 cians can prescribe different anticoagulant drugs that are best-suited for the needs of their patients. However, although anticoagulant drugs are effective in preventing VTE, they still have constraints in terms of maneuverability and associated hemorrhagic risk. The this review to revise the history and pharmacology of the different anticostillgoal haveofconstraints inis terms of maneuverability and associated hemorrhagic risk. The agulants thatreview are currently usedthe in history the clinic, to briefly outline challenges that face goal of this is to revise andand pharmacology of thethe different anticoagulants the community of thrombosis and hemostasis improve our current anticoagulants. that are currently used in the clinic, and to to briefly outline the challenges that face the community of thrombosis and hemostasis to improve our current anticoagulants. 2. A Short History of Anticoagulants: From Serendipity to Molecular Design 2. A Short History of Anticoagulants: From Serendipity to Molecular Design 2.1. Unfractionated Heparin 2.1. Unfractionated Heparin Unfractionated heparin (UFH) was the first anticoagulant drug, discovered in 1916 Unfractionated wasUniversity, the first anticoagulant in 1916 by a medical student heparin at Johns (UFH) Hopkins Jay McLean,drug, whilediscovered studying the puta-by a medical student at Johns Hopkins University, Jay McLean, while studying the putative tive prothrombotic properties of dog liver and heart extracts. Surprisingly, the comprothrombotic properties of (since dog liver andidentified heart extracts Surprisingly, the compound, pound, later named heparin it was in the. dog’s liver), demonstrated relater named heparin (since it was identified in the dog’s liver), demonstrated remarkably markably strong anticoagulant effects. In the 1930s, several labs were able to purify strong anticoagulant effects. In the 1930s, several labs were able to purify UFH, and UFH, and the compound was approved for clinical use. For the next couple of decades, the compound was approved for clinical use. For the next couple of decades, UFH was UFH was used to prevent clotting in humans, without a real understanding of the mechused to prevent clotting in humans, without a real understanding of the mechanism of anism of action. It was not until the 1970s that it was discovered that UFH is a mixture action. It was not until the 1970s that it was discovered that UFH is a mixture of of polymorphic polysaccharide chains obtained after the purification of vertebrate organs. polymorphic polysaccharide chains obtained after the purification of vertebrate organs. These polysaccharide chains significantly enhance the activity of the major natural inhibThese polysaccharide chains significantly enhance the activity of the major natural inhibitor itor of coagulation, antithrombin, which in healthy individuals accounts for the majority of coagulation, antithrombin, which in healthy individuals accounts for the majority of of all natural anticoagulation. Antithrombin is a serine protease inhibitor that primarall natural anticoagulation. Antithrombin is a serine protease inhibitor that primarily ily antagonizes thrombin and FXa, two pivotal actors within the coagulation cascade antagonizes thrombin and FXa, two pivotal actors within the coagulation cascade (Figure 1). Once antithrombin binds to thrombin or FXa, they form a complex that is rap(Figure 1). Once antithrombin binds to thrombin or FXa, they form a complex that is rapidly idly degraded circulation.The Theinteraction interaction of of UFH UFH with degraded by by thethe circulation. with antithrombin antithrombinisismostly mostly mediated by a unique pentasaccharide sequence that is randomly mediated by a unique pentasaccharide sequence that is randomlydistributed distributedalong alongthe the polysaccharide polysaccharidechains chains..Since SinceUFH UFHaccelerates acceleratesthe themode modeofofaction actionofofantithrombin antithrombinby by approximately approximately1000 1000times, times,ititfunctions functionsasasaahighly highlyefficient efficientanticoagulant anticoagulantdrug drug.. Figure1.1.Anticoagulants Anticoagulantsand andtheir theirtargets targetswithin withinthe thecoagulation coagulationcascade. cascade.The Thecoagulation coagulationcascade cascade Figure consists pathway,the thecontact contactactivation activationpathway, pathway, and comconsistsofofthree threeparts: parts:the thetissue tissue factor factor (TF) (TF) pathway, and thethe common mon pathway. Currently used anticoagulants target primarily coagulation factors from the common pathway. Currently used anticoagulants target primarily coagulation factors from the common pathway: (apixaban,edoxaban, edoxaban, fondaparinux, and rivaroxaban) and thrombin (FIIa; pathway: FXa FXa (apixaban, fondaparinux, and rivaroxaban) and thrombin (FIIa; dabigatran, dabigatran, bilvalirudin, and argatroban). UFH, VKA, and LMWH have multiple targets within the bilvalirudin, and argatroban). UFH, VKA, and LMWH have multiple targets within the coagulation cascade. Anticoagulants that are currently under development or undergoing clinical trials target factors from the TF pathway (TF-FVIIa complex) or the contact activation pathway (FIXa, FXIa, and FXIIa). Tenase complex: FVIIIa-FIXa complex. Prothrombinase complex: FVIIIa-FIXa-FXa complex. Cells 2022, 11, 3214 3 of 17 2.2. Vitamin K Antagonists In the 1930s, biochemist Karl Paul Link was contacted to investigate the mysterious massacre of cattle on several farms surrounding his laboratory at the University of Wisconsin. It took him and his team several years to determine that the hay fed to the cattle was spoiled after a series of wet summers and that one of the main constituents of spoiled hay, sweet clover, contained a toxic component. In 1939, dicumarol was extracted, and this turned out to be an effective vitamin K antagonist (VKA). In the bloodstream, dicumarol competes with vitamin K epoxide reductase, an enzyme that recycles vitamin K [20,21]. As a result, VKAs deplete circulating vitamin K over time. Several coagulation factors depend on vitamin K for their conversion from inactive zymogens to active enzymes. In contrast to heparins, VKAs are suitable for oral admission, thus avoiding the mandatory injections required for heparins. Initially, dicumarol was used to prevent thrombosis, and from the 1960s onwards its derivative warfarin, named after the Wisconsin Alumni Research Foundation, has become the reference treatment for thromboembolic events. 2.3. Low-Molecular-Weight Heparin In the 1960s–1970s, worldwide interest in antithrombotic research increased because of a growing awareness of the significant contribution of thrombosis to diseases such as stroke, myocardial infarction, and VTE. During this period, the actors within the coagulation cascade were identified one by one, and this resulted in a better mechanistic understanding of the sequence of events leading to thrombotic diseases. This improved understanding, together with advances in biochemical techniques that allowed for major advances in the development of anticoagulants. Different research groups within Europe and the US started working on the development of a subclass of heparins that are based on organ-derived UFH, the so-called low-molecular-weight heparins (LMWHs). LMWHs are derived from UFH by chemical splitting, into approximately one-third of the original size. The principal mechanism of action is similar for UFH and LMWHs (Figure 1), but LMWHs have fewer side effects and produce a more predictable anticoagulant response. Shortly after the LMWH preparations were standardized, the drugs were tested in clinical trials, and LMWHs such as enoxaparin have now replaced heparin for most thrombotic indications [26,27]. Since LMWHs are injected subcutaneously in a fixed dose without the need for consistent anticoagulant monitoring, these drugs paved the way for treating some patients with VTE as outpatients, rather than at the hospital [28,29]. 2.4. Fondaparinux, Argatroban, and Bivalirudin In recent years, rapid advances in biotechnology have enabled “designing” molecules or drugs with a predetermined purpose. To develop new anticoagulants, molecules can be generated that antagonize specific clotting factors. Structural chemists now fabricate small molecules designed to fit into the active component of clotting enzymes, like a key into a lock. Due to this specificity, these novel drugs normally coincide with fewer off-target effects and drug–drug interactions. Fondaparinux is a small molecule with a structure that is based on the active component of heparins, and it was the first of a new class of selective antithrombin-dependent FXa inhibitors (Figure 1). Compared to UFH and LMWH, although it is more expensive to fabricate the much smaller synthetic molecule, fondaparinux has more predictable pharmacokinetics that render intensive monitoring and dose adjustment unnecessary. Argatroban is a drug derived from L-arginine and is able to specifically block the active site of thrombin, thus functioning independently of antithrombin (Figure 1). For the development of novel anticoagulants, inspiration can be found from anticoagulant toxins produced by a variety of animals. Anti-clotting proteins from an array of exotic creatures, such as ticks, leeches, snakes, and vampire bats, can be studied, extracted or produced in vitro, genetically engineered, and used as drugs upon mass chemical synthesis. A synthetic drug developed using this approach is bivalirudin. Bivalirudin is an anticoagulant molecule based on the structure of hirudin, an anti-clotting substance Cells 2022, 11, 3214 4 of 17 found in leeches [35,36]. Bivalirudin is a direct thrombin inhibitor and was introduced as an alternative to heparins, to treat patients suffering from heparin-induced thrombocytopenia (Figure 1). Although fondaparinux, argatroban, and bivalirudin present significant benefits over heparins because of their pharmacokinetics, they continue to rely on parenteral admission. 2.5. Direct Oral Anticoagulants The biggest advantage of VKAs over heparins is their oral admission, even though the anticoagulant effect can be delayed for days, so intense monitoring is required. Therefore, until recently, patients requiring anticoagulants were, upon admission to a hospital, first given heparins for five to seven days, the period after which the anticoagulant effect of VKAs sets in. During this bridging period, the bleeding risk is also substantially increased, since two anticoagulant drugs are present at the same time in the circulation of the patient. The newest generation of direct oral anticoagulants (DOACs) circumvents these issues because they are administered orally and have a rapid mode of action [39,40]. Since their introduction in 2010, DOACs have largely replaced VKAs in the clinic, as they do not require the heparin bridging period. DOACs are small molecules that occupy the catalytic site of either FXa or thrombin, preventing their capacity to cleave and activate their substrates (Figure 1). Their mode of action allows for a wider therapeutic window, enables more lenient monitoring, and has a lower risk of drug–drug interaction. The direct thrombin inhibitor ximelagatran was the first DOAC that was generated based on computer modeling [42–44]. Unfortunately, in the majority of the clinical trials where ximelagatran was tested, the treatment coincided with asymptomatic elevations of liver transaminases levels through unknown mechanisms. For this reason, ximelagatran was withdrawn from the market, which highlights one of the main issues with DOACs: their liver toxicity, because of a high distribution volume. Another direct thrombin inhibitor, dabigatran, was selected from a panel of chemicals with a structure similar to benzamidinebased α-NAPAP (N-alpha-(2-naphthylsulfonylglycyl)-4-amidinophenylalanine piperidide), a compound, for which it has been known since the 1980s, that serves as a powerful inhibitor of various serine proteases, including thrombin. The addition of ethyl-ester and hexyloxycarbonyl carbamide hydrophobic side chains to α-NAPAP led to the orally absorbed prodrug dabigatran, which is used in clinics today. In addition to directly blocking thrombin using dabigatran, the serine protease upstream of thrombin in the coagulation cascade, FXa, appeared to be an intriguing target for DOACs [48,49]. FXa was already identified as a promising target for the development of new anticoagulants in the early 1980s, but the feasibility of FXa inhibition to impede thrombosis formation was not tested before the end of that decade. In 1987, the first FXa inhibitor, the naturally occurring compound antistasin, was isolated from the salivary glands of the Mexican leech, Haementeria officinalis. This specific compound did not pass clinical trials, but, in the 2000s, other FXa-antagonizing DOACs such as rivaroxaban, apixaban, and edoxaban have been introduced in clinics. Nowadays, FXa DOACs are widely used and are slowly but surely taking over the role of VKAs and heparins as the primary drugs to be prescribed to VTE patients. 3. Pharmacokinetics 3.1. UFH, LMWHs, and Fondaparinux UFH and its derivates, LMWHs and fondaparinux, are anticoagulants that are administered parenterally, either subcutaneously or intravenously. The average molecular weight of UFH is around 15,000 Da, while LMWHs are approximatively three times smaller (5000 Da). The synthetically produced drug fondaparinux, which is based on the active component of heparins, is 1780 Da. Heparins promote the activity of antithrombin, and activity can be rehearsed by the sulfate protamine that binds to circulating heparins, forming a stable salt and diminishing its anticoagulant potential. Cells 2022, 11, 3214 5 of 17 UFH is directly isolated from vertebrate tissues, and it has a number of limitations due to its unpredictable nature [56–59]. In the circulation, infused UFH has a half-life of 1–1.5 h and has peak activity 4–6 h after infusion. Since the introduction of LMWHs, drugs such as enoxaparin have largely replaced UFH in the clinic because of the lower risk of bleeding, decreased binding to plasma and endothelial proteins, and improved bioavailability. The biggest disadvantage of UFH and LMWHs is that approximately 0.2% of treated individuals develop potentially life-threatening heparin-induced thrombocytopenia (HIT) [61,62]. In short, for an unknown reason, a small group of heparin-treated individuals can generate an immune response against heparin that is in complex with circulating platelet factor 4 (PF4). Heparin–PF4–IgG-immune complexes subsequently activate platelets via their FcγIIa receptors, causing thrombocytopenia and thrombosis. The synthetic drug fondaparinux has similar pharmacological characteristics as LMWHs but has less affinity with PF4, thus rarely inducing HIT, which makes it an appealing alternative treatment option. The pharmacokinetic proprieties of UFH, the LMWH enoxaparin, and fondaparinux are summarized in Table 1. Table 1. Pharmacokinetics of UFH, enoxaparin, and fondaparinux. Other LMWHs have similar pharmacological characteristics as enoxaparin, the most frequently used. UFH: unfractionated heparin, Vd: volume of distribution, IV: intravenous, SC: subcutaneous. UFH Enoxaparin Fondaparinux Type Small molecule Small molecule Small molecule Origin Obtained from liver, lung, mast cells, and other cells of vertebrates Obtained from liver, lung, mast cells, and other cells of vertebrates Synthetic molecule FDA approval N/A, first used in clinical practice in 1941 1993 2001 Target Antithrombin Antithrombin Antithrombin Available administration route IV/SC (IV)/SC (IV)/SC Absorption N/A N/A N/A Bioavailability Unpredictable 90%–92% 100% Vd 40–70 mL/min 4.3 L 7–11 L Protein binding >90% 94% (specifically to antithrombin) Time to peak activity (h) Rapid after bolus, 4–6 after infusion 3–5 2–3 Monitoring aXa UFH aXa LMWH aXa Fondaparinux Half-life (h) 1.0–1.5 5 17–21 Elimination Reticuloendothelial system + renal Renal Renal Neutralizing agent No hemodialysis Sulfate protamine Hemodialysis Sulfate protamine Hemodialysis No sulfate protamine 3.2. Vitamin K Antagonists VKAs were the first generation of anticoagulants that are administered orally, a significant advantage over the parenterally administered heparins. Until recently, VKAs were the standard of care for oral anticoagulant treatment. Vitamin K promotes the biosynthesis of γ-carboxyglutamic acid residues in vitamin K-dependent coagulation factors (FII, FVII, FIX, and FX) that is essential for biological activity. VKAs inhibit VKCOR1, an enzyme responsible for reducing its cofactor vitamin K to its active form. As a result of VKA treatment, the pool of vitamin K in the circulation is gradually reduced and consequently coagulation Cells 2022, 11, 3214 6 of 17 is abrogated. VKA activity can be rehearsed by injecting exogenous vitamin K that serves as a neutralizing agent. Although oral admission is a great improvement, compared to parentally administered heparins, VKAs present three major flaws. First of all, VKA treatment only reaches its optimal therapeutic action 3 to 7 days after the first admission because it transiently depletes the circulating vitamin K pool. For this reason, a bridging period usually consisting of a fast-acting heparin is required [38,67,68]. Second, the dosage and admission of VKA must be monitored frequently with a coagulation test, where the international normalized ratio (INR) is determined, based on the results of a prothrombin time (PT) test performed in a laboratory. Based on the patient’s INR in response to the treatment, the dosage of VKA is adjusted. Finally, numerous interactions between VKAs and other drugs or food that can influence the efficacy of the treatment have been described [70,71]. The pharmacokinetic proprieties of warfarin, the first described and most commonly used VKA worldwide, will serve as a reference for the other VKAs, which are summarized in Table 2. Table 2. Pharmacokinetics of warfarin. Vd: volume of distribution, PO: per os (orally), INR: international normalized ratio. Warfarin Type Racemic mixture Origin Synthetic molecule FDA approval 1954 Target Vitamin K-dependent coagulation factors II, VII, IX, and X Available administration route PO Absorption Stomach and proximal small bowel Bioavailability 80%–100% Vd 8–10 L Protein binding 95%–97% Time to peak concentration (h) 1–2 Monitoring INR test Half-life (h) 20–60 Elimination Major hepatic metabolism, biotransformation > 90% of inactive metabolites (CYP 2C9) Neutralizing agent Vitamin K 3.3. Bivalirudin and Argatroban Bivalirudin and argatroban are two small synthetic molecules that are administered parentally. They both serve as direct inhibitors of thrombin and were introduced as an alternative to heparins in patients that developed HIT [72,73]. Compared to UFH and LMWH, the risk for bleeding events is comparable, but bivalirudin possesses a lower immunogenic profile and exclusively binds to thrombin in the circulation. It already reaches its peak activity several minutes after injection, and it is eliminated via the kidney or as a result of proteolytic cleavage [75,76]. In terms of general pharmacological characteristics, argatroban is comparable to bivalirudin, except for its prolonged time to peak (hours instead of minutes) and half-life (3–5 h instead of 1–1.5 h for bivalirudin). Moreover, clearance mostly takes place by hepatic hydroxylation, which has a direct effect on the endothelium, since argatroban is capable to upregulate the generation of nitric oxide. This means that argatroban treatment could have additional beneficial antithrombotic side effects because of NO-mediated inhibition of platelet activation [78,79]. The pharmacokinetic proprieties of bivalirudin and argatroban are summarized in Table 3. Cells 2022, 11, 3214 7 of 17 Table 3. Pharmacokinetics of bivalirudin and argatroban. Vd: Volume of distribution, IV: intravenous, aPTT: activated partial thromboplastin time, ACT: activated clotting time. Bivalirudin Argatroban Type Small molecule Small molecule Origin Synthetic molecule Synthetic molecule FDA approval 2000 2000 Target Thrombin Thrombin Available administration route IV IV Absorption N/A N/A Bioavailability 40%–80% 100% Vd 0.24 L/Kg 0.174 L/Kg Protein binding No plasma proteins (just thrombin) 55% (20% albumin, 35% alpha-acid glycoprotein) Time to peak activity 2–4 min 3–4 h Monitoring aPTT, ACT, ecarin clotting time aPTT, ACT Half-life (hours) 1.0–1.5 3–5 Elimination Renal and proteolytic cleavage 70% hepatic hydroxylation, 16% renal excretion as unchanged drug, 14% biliary excretion as unchanged drug Neutralizing agent N/A N/A 3.4. DOACs Since their introduction during the second decade of the 21st century, DOACs are gradually replacing other anticoagulants to treat VTE patients. Currently four DOACs are prescribed in the clinic: dabigatran, which targets thrombin, and rivaroxaban, apixaban, and edoxaban, which target FXa [39,80]. The drugs have a similar mechanism of action, as they all block the active site of their respective target enzyme that is exposed upon activation. DOACs are small synthetic molecules that are administered orally. They are rapidly absorbed in the bloodstream and, because of their direct inhibition of coagulation proteins, they form a significant improvement for daily clinical practice compared to VKAs. In addition, DOACs have significantly less contraindications with food and other drugs, which makes them more predictable, thus requiring less intense monitoring. The neutralizing agent for dabigatran is a humanized Fab fragment of an antibody (idaruzicumab) that directly binds dabigatran and neutralizes its activity. Andexanet alpha is a recombinant protein that can serve as a decoy for FXa DOACs (although, for edoxaban, the efficacy needs to be investigated), by mimicking the FXa active site without being able to engage in the formation of the prothrombinase complex. Since andexanet alpha is an expensive product, prothrombin complex concentrates (PCCs) can alternatively be provided as a neutralizing agent to patients treated with rivaroxaban, apixaban, or edoxaban. PPCs circumvent FXa DOACs, since they function downstream of FXa in the coagulation cascade. DOACs have substantially facilitated VTE treatment, both for patients and physicians, and in the coming decades DOACs will most likely be more and more prescribed instead of other anticoagulants. The pharmacokinetic proprieties of dabigatran, rivaroxaban, apixaban, and edoxaban are summarized in Table 4. Cells 2022, 11, 3214 8 of 17 Table 4. Pharmacokinetics of dabigatran, rivaroxaban, apixaban, and edoxaban. Vd: volume of distribution, PO: per os (orally). PCC: prothrombin complex concentrates. Dabigatran Rivaroxaban Apixaban Edoxaban Type Small molecule Small molecule Small molecule Small molecule Origin Synthetic molecule Synthetic molecule Synthetic molecule Synthetic molecule FDA approval 2010 2011 2014 2014 Target FIIa FXa FXa FXa Available administration route PO PO PO PO Absorption Lower stomach + duodenum Proximal small bowel + gastric Proximal small bowel + gastric Proximal small bowel Bioavailability 6.5% 66% 50% 60% Vd 50–70 L 50 L 21 L >107 L Protein binding 35% >90% 87% 40%–60% Time to peak concentration (h) 1–2 2–4 1–4 1–2 Monitoring N/A N/A N/A N/A Half-life (h) 12–17 5–9 8–15 10–14 Elimination Up to 20% glucuronidation and then biliary excretion 80% unchanged renal excretion 66% undergoes metabolic degradation (30% CYP CYP3A4/5, metabolism) 66% renal excretion 28% feces 30%–35% O-demethylation 15% CYP CYP3A4/5, metabolism 1/3 biliary excretion 1/3 renal excretion Conjugation, oxidation by CYP 3A4 and hydrolysis 1/2 biliary excretion 1/2 renal excretion Neutralizing agent Idarucizumab Andexanet alfa, PCC Andexanet alfa, PCC Andexanet alfa (?), PCC 4. Medical Indications VTE patients treated with parentally administered drugs, i.e., heparin (derivates), bivalirudin, and argatroban, can experience major discomfort with imperative intravenous or subcutaneous injections. Oral anticoagulants are easier to administer, so, for this reason, they can be preferred to treat thrombotic diseases, depending on the individual patient’s needs and their medical indication. Medical indications for parentally and orally administered anticoagulants are summarized in Tables 5 and 6, respectively. Table 5. Indications for the parenterally administered anticoagulants unfractionated heparin (UFH), enoxaparin, fondaparinux, argatroban, and bilvarudin. * based on study using another LMWH, dalteparin. Indication UFH Enoxaparin Fondaparinux Bilvarudin Argatroban Arterial thrombosis Prophylaxis of ischemic complications in the setting of unstable angina or NSTEMI yes yes / / / Treatment of atrial fibrillation with embolization yes / / / / Prophylaxis of peripheral arterial embolism yes / / / / Prophylaxis of clotting in cardiac surgery yes / / / / Prophylaxis or treatment of thrombosis in adult patients with HIT, including during PCI procedures / / / yes yes Cells 2022, 11, 3214 9 of 17 Table 5. Cont. Indication UFH Enoxaparin Fondaparinux Bilvarudin Argatroban Venous thrombosis Extended treatment of symptomatic venous thromboembolism to reduce recurrence in patients yes yes yes / / Prophylaxis of postoperative VTE in patients undergoing abdominal surgery, hip replacement surgery, or knee replacement surgery yes yes yes / / Prophylaxis of VTE in acutely ill medical patients with severely restricted mobility / yes / / / Extended treatment of symptomatic venous thromboembolism to reduce recurrence in patients with cancer / yes * / / / Other indications Extracorporeal circulation and dialysis procedures yes / / / / Treatment of acute and chronic consumptive coagulopathies yes / / / / Table 6. Indications for the orally administered anticoagulants warfarin, dabigatran, rivaroxaban, apixaban, and edoxaban. Indication Warfarin Dabigatran Rivaroxaban Apixaban Edoxaban Arterial thrombosis Reduced risk of stroke and systemic embolism in patients with NVAF yes yes yes yes yes Prophylaxis and treatment of embolic complications associated with atrial fibrillation or cardiac valve replacement yes / / / / Reduced risk of death, recurrent myocardial infarction, and stroke or systemic embolism after myocardial infarction yes / / / / Venous thrombosis Prophylaxis of DVT and PE in patients who have undergone hip replacement surgery yes yes yes yes / Treatment of DVT and PE / yes yes yes yes Reduced risk of recurrent DVT and/or PE in patients at continued risk for recurrent of VTE yes yes yes yes yes Reduced risk of recurrent DVT and/or PE in patients at continued risk for recurrent of VTE in cancer patients / / yes yes yes Cells 2022, 11, 3214 10 of 17 5. Drug–Drug Interactions Oral anticoagulants come with a lower burden for VTE patients, since no medical personnel or injections are required for their admission. With their rapid mode of action, especially the novel VTE drugs, DOACs are preferred over other currently used drugs. However, compared to parenteral anticoagulants, oral anticoagulants are often accompanied by the risk of undesired drug–drug interaction (DDI). DDIs are secondary to the specific effect of a therapeutic and can occur when two administered drugs share the same cell membrane transporter or metabolic pathway. Oral anticoagulants need to pass from the gastrointestinal system into the circulation, and, subsequently, they are metabolized by renal or hepatic clearance. Their transport to and from the circulation is mediated by the transporter glycoproteins, such as P-GP. Drugs administered to treat atrial fibrillation may interfere with DOAC transport by P-GP, and this leads to an increase in the anticoagulant activity. For instance, dabigatran activity could increase by up to 70% in patients that are treated with dronedarone. For this reason, to treat atrial fibrillation, the drug amiodarone, a moderate P-GP competitor, is preferred to dronedarone in patients using DOACs. In cancer patients, imatinib and crizotinib, two tyrosine kinases, are contraindicated for DOAC patients, due to their strong inhibitory capacity of P-GP. Enzalutamide, a prostate cancer hormonotherapy, promotes P-GP function, and this could reduce the concentration of the administered DOAC, resulting in an increased risk for recurrent VTE. P450 cytochromes CYP2C9, CYP1A2, and CYP3A4 mediate the elimination of oral anticoagulants, and DOAC or VKA patients that receive P450-cytochromes-inhibiting drugs have an increased bleeding risk. Drugs to treat atrial fibrillation and cancer are most often cited for their DDI with oral anticoagulants, but DDIs with statins and antibiotic, antidepressant, and antiviral drugs have also been described [89–91]. Certain intrinsic characteristics of individual patients may cause anticoagulants to have a different metabolism. It has been demonstrated that polymorphisms on transporters or P450 cytochromes can lead to unexpected variability in drug efficacy. The same holds true for polymorphisms in the VKCOR1 gene, the target of VKAs, which can cause individuals to have a delayed response towards the drug. Patients with liver or kidney failure demonstrate a delay in the elimination of anticoagulants, thereby increasing the plasma concentration and the risk of bleeding [93,94]. 6. Introducing DOACs in Complex Patient Populations In many clinical trials to investigate the effects of a certain drug, children are excluded due to their physical vulnerability. For this specific patient population, the use of DOACs to prevent or cure (recurrent) VTE will most likely significantly improve the quality of care, since the drug is administered orally and it does not require intense monitoring. The EINSTEIN Junior study was the first study designed to test DOACs in pediatric VTE patients. Within this study, the efficacy and the safety of rivaroxaban was compared to regular treatment, i.e., treatment with VKA, LMWH, or UFH. Within four years, more than 500 children were recruited, and patients treated with rivaroxaban demonstrated a similar recurrence risk of VTE and bleeding risk, compared to other anticoagulants. Currently, the EINSTEIN Junior study is the largest study to be carried out in children testing DOACs, and, although DOACs appear safe and effective in pediatric VTE patients, we must remain vigilant for their use in this vulnerable population. Heparins are the only anticoagulants that are authorized during pregnancy, since VKAs and DOACs pass the placental barrier. However, unlike for VKAs, the exact effects of DOACs on the developing fetus are unknown. In 2020, a registry of women who had been pregnant while receiving DOACs was published by Beyer-Westendorf et al.. In that registry, from 2007 to 2020, 336 mothers were included that were exposed to DOACs during pregnancy. In total, 6% of the women displayed a non-normal fetus growth (95% confidence interval 4%–9%, comparable to the normal population). Despite these promising results, physicians remain reluctant to prescribe DOACs in pregnant women. In addition, Cells 2022, 11, 3214 11 of 17 DOACs are assumed to pass into breast milk, so, for this reason, the drugs are currently not prescribed in nursing mothers [98,99]. The RAPS and TRAPS clinical studies were designed to evaluate DOACs in preventing VTE in triple-positive antiphospholipid syndrome (APS) patients [100,101]. Although the first study provided promising results, making use of a biologic test as a clinical surrogate marker , the second study was prematurely stopped due to a major safety issue. It appeared that the use of the anti-FXa DOAC rivaroxaban was associated with an increased risk of thrombotic events, compared to VKA. Based on these results, the use of DOACs is, currently, strongly discouraged in APS patients. However, APS is a complex disease, for which the pathophysiology is not completely understood, so a dedicated study investigating the putative mechanism by which DOACs increase VTE risk in APS patients is required. However, the aforementioned study only tested rivaroxaban and no other DOAC and did not include single- or double-positive APS patients, while real-life data of non-triple-positive APS patients who remained on DOACs are reassuring. These shortcomings argue for new studies on DOACs in triple-positive APS patients. In 2013, Eikelboom at al. published a study comparing dabigatran to warfarin in patients with mechanical heart valves. The study was stopped prematurely because, for unknown reasons, an excess of thrombotic events in the dabigatran group was observed (32% vs. 6% in the warfarin group). Interestingly, in a recently published study, rivaroxabantreated patients with organic heart valve disease-associated atrial fibrillation showed an increase in cardiovascular events and death in DOAC patients, compared to patients under VKA treatment. These data strongly imply that DOACs should be avoided in patients with mechanical or organic heart valves. After a pulmonary embolism event, optimal follow-up comprises monitoring for longterm complications, such as chronic thromboembolic pulmonary hypertension (CTEPH). The efficacy and safety of DOACs to prevent recurrent VTE is challenged because of a higher incidence of CTEPH, compared to VKA-treated patients, possibly because of clinically relevant drug–drug interaction [106,107]. Hence, updated guidelines recommend to prescribe VKA over DOACs in patients with CTEPH. In atherosclerosis, the COMPASS trial (Cardiovascular Outcomes for People Using Anticoagulation Strategies) showed that combining regular aspirin treatment with rivaroxaban (2.5 mg twice daily) was beneficial for coronary, cerebrovascular, and peripheral end points in patients with and without diabetes mellitus. The beneficial effect of aspirin and rivaroxaban has not been confirmed yet for VTE patients. Combination therapy using anticoagulants and anti-aggregants in DVT was assessed by a Cochrane meta-analysis. Following the initial standard treatment with anticoagulants, there appeared to be lowcertainty evidence that antiplatelet agents, in addition to standard anticoagulation and other clinical practices (like stocking socks), reduce recurrent VTE, with no clear differences of adverse events such as major bleeding or recurrent VTE. For PE, no recent studies have been published on the subject of combination therapy. Fear of bleeding events should not be an obstacle to the production of reassuring data on the risk of bleeding associated with anticoagulant and anti-aggregant combination therapy. However, dedicated studies are needed to explore the potential of combining drugs to prevent or cure VTE. 7. A New Generation of Anticoagulants When introducing a new drug on the market, it should be beneficial compared to existing agents. This benefit can consist of improvements in, for instance, higher efficacy, easier admission, less strict monitoring, and reduced side effects. In VTE patients, bleeding as a consequence of anticoagulant treatment is a major issue, so, for this reason, the scientific community of thrombosis and hemostasis has proposed multiple innovative strategies, with the goal to combat thrombosis in humans without (excessive) bleeding as a side effect. These novel strategies can be based on new techniques, such as making use of antisense oligonucleotides, antibodies, or aptamers, or based on targeting other components from the coagulation cascade, such as the FVIIa–tissue factor complex, FIXa, Cells 2022, 11, 3214 12 of 17 FXIIa, or FXIa (Figure 1). The latter coagulation factor is of especially great interest, since inhibition of FXIa seems to prevent thrombosis without (excessive) bleeding as a side effect. Abelacimab is an anti-FXIa antibody that is currently being tested in Phase III studies in patients with cancer-associated thrombosis (https://clinicaltrials.gov/ ct2/show/NCT05171049 and https://clinicaltrials.gov/ct2/show/NCT05171075 (both accessed on 1 September 2022)). Cancer-associated thrombosis (CAT) patients form a frail population that is challenging to treat, since they have an increased risk for both (recurrent) VTE and anticoagulation-associated bleeding. According to current guidelines, to limit the risk of excessive bleedings, CAT patients should be treated with great caution when using DOACs or LMWHs [113–116]. FXIa drugs are associated with a lower bleeding risk and may be administered at a higher dosage in CAT patients to prevent recurrent VTE, thereby improving patient care. If these clinical studies on CAT patients prove to be successful, FXIa drugs will most likely be tested in other frail VTE patient populations. 8. Conclusions In the last 100 years, several anticoagulant drugs have been introduced on the market. All drugs have a different history, mechanism, admission route, pharmacokinetics, and medical indication. Anticoagulants have aided considerably in combatting thrombotic diseases, although they all have their specific flaws, so, for this reason, it is of great interest to the scientific community of thrombosis and hemostasis to investigate novel anticoagulants. The perfect anticoagulant should be well-tolerated by the entire patient population, be orally administered with a wide therapeutic window, and have favorable absorption, distribution, and elimination. In addition, efficacy of the drug should be easy to test, a proper neutralizing agent should be available, and, perhaps most importantly, the drug should have limited to no side effects such as bleeding. New anticoagulants such as the FXIa inhibitors might present an improvement compared to the current treatment options. Author Contributions: Conceptualization: M.H., G.P. and L.B. Literature searches: M.H. and G.P. Writing—original draft preparation: M.H. and G.P. Visualization: G.P. Revision of the original draft: H.H.-C., F.C. and L.B. All authors have read and agreed to the published version of the manuscript. Funding: This research received no external funding. Conflicts of Interest: The authors declare no conflict of interest. References 1. 2. 3. 4. 5. 6. 7. 8. 9. 10. Fang, J.; Alderman, M.H. Dissociation of hospitalization and mortality trends for myocardial infarction in the United States from 1988 to 1997. Am. J. Med. 2002, 113, 208–214. [CrossRef] Feigin, V.L.; Lawes, C.M.; Bennett, D.A.; Anderson, C.S. Stroke epidemiology: A review of population-based studies of incidence, prevalence, and case-fatality in the late 20th century. Lancet Neurol. 2003, 2, 43–53. [CrossRef] White, R.H. The Epidemiology of Venous Thromboembolism. Circulation 2003, 107, I-4–I-8. [CrossRef] Mackman, N.; Bergmeier, W.; Stouffer, G.A.; Weitz, J.I. Therapeutic strategies for thrombosis: New targets and approaches. Nat. Rev. Drug Discov. 2020, 19, 333–352. [CrossRef] Ten Cate, H.; Hackeng, T.M.; de Frutos, P.G. Coagulation factor and protease pathways in thrombosis and cardiovascular disease. Thromb. Haemost. 2017, 117, 1265–1271. [CrossRef] Mclean, J. The Discovery of Heparin. Circulation 1959, 19, 75–78. [CrossRef] Last, J.A. The Missing Link: The Story of Karl Paul Link. Toxicol. Sci. 2002, 66, 4–6. [CrossRef] Weitz, J.I.; Jaffer, I.H.; Fredenburgh, J.C. Recent advances in the treatment of venous thromboembolism in the era of the direct oral anticoagulants. F1000Research 2017, 6, 985. [CrossRef] Yeh, C.H.; Gross, P.L.; Weitz, J.I. Evolving use of new oral anticoagulants for treatment of venous thromboembolism. Blood 2014, 124, 1020–1028. [CrossRef] Pollack, C.V.; Peacock, F.W.; Bernstein, R.A.; Clark, C.L.; Douketis, J.; Fermann, G.J.; Fiore, G.J.; Frost, A.; Jahromi, B.; Johnson, C.; et al. The safety of oral anticoagulants registry (SOAR): A national, ED-based study of the evaluation and management of bleeding and bleeding concerns due to the use of oral anticoagulants. Am. J. Emerg. Med. 2020, 38, 1163–1170. [CrossRef] Cells 2022, 11, 3214 11. 12. 13. 14. 15. 16. 17. 18. 19. 20. 21. 22. 23. 24. 25. 26. 27. 28. 29. 30. 31. 32. 33. 34. 35. 36. 37. 38. 39. 40. 13 of 17 Oates, J.A.; Wood, A.J.J.; Hirsh, J. Heparin. N. Engl. J. Med. 1991, 324, 1565–1574. [CrossRef] Couch, N.P. About heparin, or... Whatever happened to Jay McLean? J. Vasc. Surg. 1989, 10, 1–8. Abildgaard, U. Highly Purified Antithrombin III with Heparin Cofactor Activity Prepared by Disc Electrophoresis. Scand. J. Clin. Lab. Investig. 1968, 21, 89–91. [CrossRef] Rosenberg, R.D.; Rosenberg, J.S. Natural anticoagulant mechanisms. J. Clin. Investig. 1984, 74, 1–6. [CrossRef] Rezaie, A.R.; Giri, H. Anticoagulant and signaling functions of antithrombin. J. Thromb. Haemost. 2020, 18, 3142–3153. [CrossRef] Huntington, J.A. Serpin structure, function and dysfunction: Serpin structure, function and dysfunction. J. Thromb. Haemost. 2011, 9, 26–34. [CrossRef] Casu, B.; Oreste, P.; Torri, G.; Zoppetti, G.; Choay, J.; Lormeau, J.C.; Petitou, M.; Sinäy, P. The structure of heparin oligosaccharide fragments with high anti-(factor Xa) activity containing the minimal antithrombin III-binding sequence. Chemical and 13C nuclear-magnetic-resonance studies. Biochem. J. 1981, 197, 599–609. [CrossRef] Damus, P.S.; Hicks, M.; Rosenberg, R.D. Anticoagulant Action of Heparin. Nature 1973, 246, 355–357. [CrossRef] Link, K.P. The Discovery of Dicumarol and Its Sequels. Circulation 1959, 19, 97–107. [CrossRef] Whitlon, D.S.; Sadowski, J.A.; Suttie, J.W. Mechanism of coumarin action: Significance of vitamin K epoxide reductase inhibition. Biochemistry 1978, 17, 1371–1377. [CrossRef] Bell, R.G. Metabolism of vitamin K and prothrombin synthesis: Anticoagulants and the vitamin K–epoxide cycle. Fed. Proc. 1978, 37, 2599–2604. Stenflo, J.; Fernlund, P.; Egan, W.; Roepstorff, P. Vitamin K Dependent Modifications of Glutamic Acid Residues in Prothrombin. Proc. Natl. Acad. Sci. USA 1974, 71, 2730–2733. [CrossRef] Sackett, D.L. Clinical epidemiology. J. Clin. Epidemiol. 2002, 55, 1161–1166. [CrossRef] Johnson, E.A.; Kirkwood, T.B.; Stirling, Y.; Perez-Requejo, J.L.; Ingram, G.I.; Bangham, D.R.; Brozović, M. Four heparin preparations: Anti-Xa potentiating effect of heparin after subcutaneous injection. Thromb. Haemost. 1976, 35, 586–591. [CrossRef] Fareed, J.; Kumar, A.; Walenga, J.M.; Emanuele, R.M.; Williamson, K.; Hoppensteadt, D. Antithrombotic actions and pharmacokinetics of heparin fractions and fragments. Nouv. Rev. Fr. d’Hematol. 1984, 26, 267–275. Colombus Investigators. Low-Molecular-Weight Heparin in the Treatment of Patients with Venous Thromboembolism. N. Engl. J. Med. 1997, 337, 657–662. [CrossRef] Simonneau, G.; Sors, H.; Charbonnier, B.; Page, Y.; Laaban, J.P.; Azarian, R.; Laurent, M.; Hirsch, J.L.; Ferrari, E.; Bosson, J.L.; et al. A Comparison of Low-Molecular-Weight Heparin with Unfractionated Heparin for Acute Pulmonary Embolism. N. Engl. J. Med. 1997, 337, 663–669. [CrossRef] Levine, M.; Gent, M.; Hirsh, J.; Leclerc, J.; Anderson, D.; Weitz, J.; Ginsberg, J.; Turpie, A.G.; Demers, C.; Kovacs, M. A comparison of low-molecular-weight heparin administered primarily at home with unfractionated heparin administered in the hospital for proximal deep-vein thrombosis. N. Engl. J. Med. 1996, 334, 677–681. [CrossRef] Koopman, M.M.; Prandoni, P.; Piovella, F.; Ockelford, P.A.; Brandjes, D.P.; van der Meer, J.; Gallus, A.S.; Simonneau, G.; Chesterman, C.H.; Prins, M.H. Treatment of venous thrombosis with intravenous unfractionated heparin administered in the hospital as compared with subcutaneous low-molecular-weight heparin administered at home. The Tasman Study Group. N. Engl. J. Med. 1996, 334, 682–687. [CrossRef] Zhou, S.-F.; Zhong, W.-Z. Drug Design and Discovery: Principles and Applications. Molecules 2017, 22, 279. [CrossRef] Anderson, A.C. The Process of Structure-Based Drug Design. Chem. Biol. 2003, 10, 787–797. [CrossRef] [PubMed] Walenga, J.M.; Jeske, W.P.; Samama, M.M.; Frapaise, X.F.; Bick, R.L.; Fareed, J. Fondaparinux: A synthetic heparin pentasaccharide as a new antithrombotic agent. Expert Opin. Investig. Drugs 2002, 11, 397–407. [CrossRef] [PubMed] Fitzgerald, D.; Murphy, N. Argatroban: A synthetic thrombin inhibitor of low relative molecular mass. Coron. Artery Dis. 1996, 7, 455–458. [CrossRef] Oliveira, A.L.; Viegas, M.F.; da Silva, S.L.; Soares, A.M.; Ramos, M.J.; Fernandes, P.A. The chemistry of snake venom and its medicinal potential. Nat. Rev. Chem. 2022, 6, 451–469. [CrossRef] [PubMed] Nowak, G. Pharmacology of Recombinant Hirudin. Semin. Thromb. Hemost. 2002, 28, 415–424. [CrossRef] [PubMed] Fenton, J.W. Leeches to hirulogs and other thrombin-directed antithrombotics. Hematol. Oncol. Clin. N. Am. 1992, 6, 1121–1129. [CrossRef] Bain, J.; Meyer, A. Comparison of bivalirudin to lepirudin and argatroban in patients with heparin-induced thrombocytopenia. Am. J. Health-Syst. Pharm. 2015, 72, S104–S109. [CrossRef] Siegal, D.; Yudin, J.; Kaatz, S.; Douketis, J.D.; Lim, W.; Spyropoulos, A.C. Periprocedural heparin bridging in patients receiving vitamin K antagonists: Systematic review and meta-analysis of bleeding and thromboembolic rates. Circulation 2012, 126, 1630–1639. [CrossRef] Chen, A.; Stecker, E.; Warden, B.A. Direct Oral Anticoagulant Use: A Practical Guide to Common Clinical Challenges. J. Am. Heart Assoc. 2020, 9, e017559. [CrossRef] Rose, D.K.; Bar, B. Direct Oral Anticoagulant Agents: Pharmacologic Profile, Indications, Coagulation Monitoring, and Reversal Agents. J. Stroke Cerebrovasc. Dis. 2018, 27, 2049–2058. [CrossRef] Cells 2022, 11, 3214 41. 42. 43. 44. 45. 46. 47. 48. 49. 50. 51. 52. 53. 54. 55. 56. 57. 58. 59. 60. 61. 62. 63. 64. 65. 66. 14 of 17 Gómez-Outes, A.; Suárez-Gea, M.L.; Lecumberri, R.; Terleira-Fernández, A.I.; Vargas-Castrillón, E. Direct-acting oral anticoagulants: Pharmacology, indications, management, and future perspectives. Eur. J. Haematol. 2015, 95, 389–404. [CrossRef] [PubMed] Eriksson, H.; Wåhlander, K.; Gustafsson, D.; Welin, L.T.; Frison, L.; Schulman, S.; Thrive Investigators. A randomized, controlled, dose-guiding study of the oral direct thrombin inhibitor ximelagatran compared with standard therapy for the treatment of acute deep vein thrombosis: THRIVE I. J. Thromb. Haemost. 2003, 1, 41–47. [CrossRef] [PubMed] Francis, C.W.; Berkowitz, S.D.; Comp, P.C.; Lieberman, J.R.; Ginsberg, J.S.; Paiement, G.; Peters, G.R.; Roth, A.W.; McElhattan, J.; Colwell, C.W., Jr.; et al. Comparison of Ximelagatran with Warfarin for the Prevention of Venous Thromboembolism after Total Knee Replacement. N. Engl. J. Med. 2003, 349, 1703–1712. [CrossRef] [PubMed] Schulman, S.; Wåhlander, K.; Lundström, T.; Clason, S.B.; Eriksson, H.; THRIVE III Investigators. Secondary prevention of venous thromboembolism with the oral direct thrombin inhibitor ximelagatran. N. Engl. J. Med. 2003, 349, 1713–1721. [CrossRef] Cully, M. Ximelagatran sets the stage for NOACs. Nat. Rev. Cardiol. 2017. [CrossRef] Svendsen, L.; Brogli, M.; Lindeberg, G.; Stocker, K. Differentiation of thrombin- and factor Xa-related amidolytic activity in plasma by means of a synthetic thrombin inhibitor. Thromb. Res. 1984, 34, 457–462. [CrossRef] Hauel, N.H.; Nar, H.; Priepke, H.; Ries, U.; Stassen, J.; Wienen, W. Structure-Based Design of Novel Potent Nonpeptide Thrombin Inhibitors. J. Med. Chem. 2002, 45, 1757–1766. [CrossRef] Milling, T.J.; Ziebell, C.M. A review of oral anticoagulants, old and new, in major bleeding and the need for urgent surgery. Trends Cardiovasc. Med. 2020, 30, 86–90. [CrossRef] Perzborn, E.; Roehrig, S.; Straub, A.; Kubitza, D.; Misselwitz, F. The discovery and development of rivaroxaban, an oral, direct factor Xa inhibitor. Nat. Rev. Drug Discov. 2011, 10, 61–75. [CrossRef] Nutt, E.; Gasic, T.; Rodkey, J.; Gasic, G.J.; Jacobs, J.W.; Friedman, P.A.; Simpson, E. The amino acid sequence of antistasin. A potent inhibitor of factor Xa reveals a repeated internal structure. J. Biol. Chem. 1988, 263, 10162–10167. [CrossRef] Mavrakanas, T.; Bounameaux, H. The potential role of new oral anticoagulants in the prevention and treatment of thromboembolism. Pharmacol. Ther. 2011, 130, 46–58. [CrossRef] [PubMed] Bertoletti, L.; Gusto, G.; Khachatryan, A.; Quignot, N.; Chaves, J.; Moniot, A.; Mokgokong, R. Effectiveness and Safety of Oral Anticoagulants in the Treatment of Acute Venous Thromboembolism: A Nationwide Comparative Cohort Study in France. Thromb. Haemost. 2022, 122, 1384–1396. [CrossRef] [PubMed] Hirsh, J.; Warkentin, T.E.; Shaughnessy, S.G.; Anand, S.S.; Halperin, J.J.; Raschke, R.; Granger, C.; Ohman, E.M.; Dalen, J.E. Heparin and low-molecular-weight heparin: Mechanisms of action, pharmacokinetics, dosing, monitoring, efficacy, and safety. Chest 2001, 119, 64S–94S. [CrossRef] Weitz, J.I. Low-molecular-weight heparins. N. Engl. J. Med. 1997, 337, 688–698. [CrossRef] [PubMed] Sokolowska, E.; Kalaska, B.; Miklosz, J.; Mogielnicki, A. The toxicology of heparin reversal with protamine: Past, present and future. Expert Opin. Drug Metab. Toxicol. 2016, 12, 897–909. [CrossRef] [PubMed] Howell, W.H.; Holt, E. Two New Factors in Blood Coagulation—Heparin and Pro-Antithrombin. Am. J. Physiol. -Leg. Content 1918, 47, 328–341. [CrossRef] Hirsh, J.; van Aken, W.G.; Gallus, A.S.; Dollery, C.T.; Cade, J.F.; Yung, W.L. Heparin kinetics in venous thrombosis and pulmonary embolism. Circulation 1976, 53, 691–695. [CrossRef] Young, E.; Prins, M.; Levine, M.N.; Hirsh, J. Heparin binding to plasma proteins, an important mechanism for heparin resistance. Thromb. Haemost. 1992, 67, 639–643. [CrossRef] Bârzu, T.; Molho, P.; Tobelem, G.; Petitou, M.; Caen, J. Binding and endocytosis of heparin by human endothelial cells in culture. Biochim. Biophys. Acta BBA -Mol. Cell Res. 1985, 845, 196–203. [CrossRef] Young, E.; Wells, P.; Holloway, S.; Weitz, J.; Hirsh, J. Ex-vivo and in-vitro evidence that low molecular weight heparins exhibit less binding to plasma proteins than unfractionated heparin. Thromb. Haemost. 1994, 71, 300–304. [CrossRef] Bloemen, A.; Testroote, M.J.G.; Janssen-Heijnen, M.L.G.; Janzing, H.M.J. Incidence and diagnosis of heparin-induced thrombocytopenia (HIT) in patients with traumatic injuries treated with unfractioned or low-molecular-weight heparin: A literature review. Injury 2012, 43, 548–552. [CrossRef] [PubMed] Marchetti, M.; Zermatten, M.G.; Bertaggia Calderara, D.; Aliotta, A.; Alberio, L. Heparin-Induced Thrombocytopenia: A Review of New Concepts in Pathogenesis, Diagnosis, and Management. J. Clin. Med. 2021, 10, 683. [CrossRef] [PubMed] Amiral, J.; Bridey, F.; Dreyfus, M.; Vissoc, A.M.; Fressinaud, F.; Wolf, M.; Meyer, D. Platelet factor 4 complexed to heparin is the target for antibodies generated in heparin-induced thrombocytopenia. Thromb. Haemost. 1992, 68, 95–96. [CrossRef] [PubMed] Burch, M.; Cooper, B. Fondaparinux-Associated Heparin-Induced Thrombocytopenia. Bayl. Univ. Med. Cent. Proc. 2012, 25, 13–15. [CrossRef] Nelsestuen, G.L.; Zytkovicz, T.H.; Howard, J.B. The mode of action of vitamin K. Identification of gamma-carboxyglutamic acid as a component of prothrombin. J. Biol. Chem. 1974, 249, 6347–6350. [CrossRef] Hanley, J.P. Warfarin reversal. J. Clin. Pathol. 2004, 57, 1132–1139. [CrossRef] Cells 2022, 11, 3214 67. 68. 69. 70. 71. 72. 73. 74. 75. 76. 77. 78. 79. 80. 81. 82. 83. 84. 85. 86. 87. 88. 89. 90. 91. 92. 15 of 17 Hull, R.D.; Raskob, G.E.; Hirsh, J.; Jay, R.M.; Leclerc, J.R.; Geerts, W.H.; Rosenbloom, D.; Sackett, D.L.; Anderson, C.; Harrison, L. Continuous Intravenous Heparin Compared with Intermittent Subcutaneous Heparin in the Initial Treatment of Proximal-Vein Thrombosis. N. Engl. J. Med. 1986, 315, 1109–1114. [CrossRef] [PubMed] Salzman, E.W.; Deykin, D.; Shapiro, R.M.; Rosenberg, R. Management of Heparin Therapy: Controlled Prospective Trial. N. Engl. J. Med. 1975, 292, 1046–1050. [CrossRef] [PubMed] Poller, L. Standardization of anticoagulant control. Ric. Clin. Lab. 1978, 8, 237–247. [CrossRef] Wang, M.; Zeraatkar, D.; Obeda, M.; Lee, M.; Garcia, C.; Nguyen, L.; Agarwal, A.; Al-Shalabi, F.; Benipal, H.; Ahmad, A.; et al. Drug-drug interactions with warfarin: A systematic review and meta-analysis. Br. J. Clin. Pharmacol. 2021, 87, 4051–4100. [CrossRef] Hirsh, J.; Dalen, J.; Anderson, D.R.; Poller, L.; Bussey, H.; Ansell, J.; Deykin, D. Oral Anticoagulants: Mechanism of Action, Clinical Effectiveness, and Optimal Therapeutic Range. Chest 2001, 119, 8S–21S. [CrossRef] [PubMed] Lewis, B.E.; Wallis, D.E.; Berkowitz, S.D.; Matthai, W.H.; Fareed, J.; Walenga, J.M.; Bartholomew, J.; Sham, R.; Lerner, R.G.; Zeigler, Z.R.; et al. Argatroban Anticoagulant Therapy in Patients With Heparin-Induced Thrombocytopenia. Circulation 2001, 103, 1838–1843. [CrossRef] [PubMed] Warkentin, T.E. Heparin-Induced Thrombocytopenia: Diagnosis and Management. Circulation 2004, 110, e454–e458. [CrossRef] [PubMed] Eichler, P.; Lubenow, N.; Strobel, U.; Greinacher, A. Antibodies against lepirudin are polyspecific and recognize epitopes on bivalirudin. Blood 2004, 103, 613–616. [CrossRef] [PubMed] Shammas, N.W. Bivalirudin: Pharmacology and clinical applications. Cardiovasc. Drug Rev. 2005, 23, 345–360. [CrossRef] [PubMed] Van de Car, D.A.; Rao, S.V.; Ohman, E.M. Bivalirudin: A review of the pharmacology and clinical application. Expert Rev. Cardiovasc. Ther. 2010, 8, 1673–1681. [CrossRef] Jeske, W.P.; Fareed, J.; Hoppensteadt, D.A.; Lewis, B.; Walenga, J.M. Pharmacology of argatroban. Expert Rev. Hematol. 2010, 3, 527–539. [CrossRef] Bellomo, T.R.; Jeakle, M.A.; Meyerhoff, M.E.; Bartlett, R.H.; Major, T.C. The Effects of the Combined Argatroban/Nitric OxideReleasing Polymer on Platelet Microparticle-Induced Thrombogenicity in Coated Extracorporeal Circuits. ASAIO J. 2021, 67, 573–582. [CrossRef] Major, T.C.; Handa, H.; Brisbois, E.J.; Reynolds, M.M.; Annich, G.M.; Meyerhoff, M.E.; Bartlett, R.H. The mediation of platelet quiescence by NO-releasing polymers via cGMP-induced serine 239 phosphorylation of vasodilator-stimulated phosphoprotein. Biomaterials 2013, 34, 8086–8096. [CrossRef] Ageno, W.; Haas, S.; Weitz, J.I.; Goldhaber, S.Z.; Turpie, A.G.C.; Goto, S.; Pantep Angchaisuksiri, P.; Dalsgaard Nielsen, J.; Kayani, G.; Pieper, K.S.; et al. Characteristics and Management of Patients with Venous Thromboembolism: The GARFIELD-VTE Registry. Thromb. Haemost. 2019, 119, 319–327. [CrossRef] Hauptmann, J.; Stürzebecher, J. Synthetic Inhibitors of Thrombin and Factor Xa. Thromb. Res. 1999, 93, 203–241. [PubMed] Pollack, C.V.; Reilly, P.A.; Eikelboom, J.; Glund, S.; Verhamme, P.; Bernstein, R.A.; Dubiel, R.; Huisman, M.V.; Hylek, E.M.; Kamphuisen, P.W.; et al. Idarucizumab for Dabigatran Reversal. N. Engl. J. Med. 2015, 373, 511–520. [CrossRef] Connolly, S.J.; Milling, T.J., Jr.; Eikelboom, J.W.; Gibson, M.C.; Curnutte, J.T.; Gold, A.; Bronson, M.D.; Lu, G.; Conley, P.B.; Verhamme, P.; et al. Andexanet Alfa for Acute Major Bleeding Associated with Factor Xa Inhibitors. N. Engl. J. Med. 2016, 375, 1131–1141. [CrossRef] [PubMed] Cuker, A.; Burnett, A.; Triller, D.; Crowther, M.; Ansell, J.; Van Cott, E.M.; Wirth, D.; Kaatz, S. Reversal of direct oral anticoagulants: Guidance from the Anticoagulation Forum. Am. J. Hematol. 2019, 94, 697–709. [CrossRef] [PubMed] Ferri, N.; Colombo, E.; Tenconi, M.; Baldessin, L.; Corsini, A. Drug-Drug Interactions of Direct Oral Anticoagulants (DOACs): From Pharmacological to Clinical Practice. Pharmaceutics 2022, 14, 1120. [CrossRef] Raymond, J.; Imbert, L.; Cousin, T.; Duflot, T.; Varin, R.; Wils, J.; Lamoureux, F. Pharmacogenetics of Direct Oral Anticoagulants: A Systematic Review. J. Pers. Med. 2021, 11, 37. [CrossRef] Steffel, J.; Collins, R.; Antz, M.; Cornu, P.; Desteghe, L.; Haeusler, K.G.; Oldgren, J.; Reinecke, H.; Roldan-Schilling, V.; Rowell, N.; et al. 2021 European Heart Rhythm Association Practical Guide on the Use of Non-Vitamin K Antagonist Oral Anticoagulants in Patients with Atrial Fibrillation. EP Eur. 2021, 23, 1612–1676. Gibbons, J.A.; de Vries, M.; Krauwinkel, W.; Ohtsu, Y.; Noukens, J.; van der Walt, J.; Mol, R.; Mordenti, J.; Ouatas, T. Pharmacokinetic Drug Interaction Studies with Enzalutamide. Clin. Pharmacokinet. 2015, 54, 1057–1069. [CrossRef] Li, A.; Li, M.K.; Crowther, M.; Vazquez, S.R. Drug-drug interactions with direct oral anticoagulants associated with adverse events in the real world: A systematic review. Thromb. Res. 2020, 194, 240–245. [CrossRef] Baillargeon, J.; Holmes, H.M.; Lin, Y.; Raji, M.A.; Sharma, G.; Kuo, Y. Concurrent use of warfarin and antibiotics and the risk of bleeding in older adults. Am. J. Med. 2012, 125, 183–189. [CrossRef] Forbes, H.L.; Polasek, T.M. Potential drug–drug interactions with direct oral anticoagulants in elderly hospitalized patients. Ther. Adv. Drug Saf. 2017, 8, 319–328. [CrossRef] [PubMed] McDonough, C.W. Pharmacogenomics in Cardiovascular Diseases. Curr. Protoc. 2021, 1, e189. [CrossRef] [PubMed] Cells 2022, 11, 3214 93. 94. 95. 96. 97. 98. 99. 100. 101. 102. 103. 104. 105. 106. 107. 108. 109. 110. 111. 112. 113. 16 of 17 Padrini, R. Clinical Pharmacokinetics and Pharmacodynamics of Direct Oral Anticoagulants in Patients with Renal Failure. Eur. J. Drug Metab. Pharmacokinet. 2019, 44, 1–12. [CrossRef] [PubMed] Ballestri, S.; Capitelli, M.; Fontana, M.C.; Arioli, D.; Romagnoli, E.; Graziosi, C.; Lonardo, A.; Marietta, M.; Dentali, F.; Cioni, G. Direct Oral Anticoagulants in Patients with Liver Disease in the Era of Non-Alcoholic Fatty Liver Disease Global Epidemic: A Narrative Review. Adv. Ther. 2020, 37, 1910–1932. [PubMed] Caldwell, P.H.Y.; Murphy, S.B.; Butow, P.N.; Craig, J.C. Clinical trials in children. Lancet 2004, 364, 803–811. [CrossRef] Male, C.; Lensing, A.W.A.; Palumbo, J.S.; Kumar, R.; Nurmeev, I.; Hege, K.; Bonnet, D.; Connor, P.; Hooimeijer, H.L.; Torres, M. Rivaroxaban compared with standard anticoagulants for the treatment of acute venous thromboembolism in children: A randomised, controlled, phase 3 trial. Lancet Haematol. 2020, 7, e18–e27. [CrossRef] Beyer-Westendorf, J.; Tittl, L.; Bistervels, I.; Middeldorp, S.; Schaefer, C.; Paulus, W.; Thomas, W.; Kemkes-Matthes, B.; Sandra Marten, S.; Bornhauser, M. Safety of direct oral anticoagulant exposure during pregnancy: A retrospective cohort study. Lancet Haematol. 2020, 7, e884–e891. [CrossRef] Wiesen, M.H.J.; Blaich, C.; Müller, C.; Streichert, T.; Pfister, R.; Michels, G. The Direct Factor Xa Inhibitor Rivaroxaban Passes Into Human Breast Milk. Chest 2016, 150, e1–e4. [CrossRef] Daei, M.; Khalili, H.; Heidari, Z. Direct oral anticoagulant safety during breastfeeding: A narrative review. Eur. J. Clin. Pharmacol. 2021, 77, 1465–1471. [CrossRef] Pengo, V.; Banzato, A.; Bison, E.; Zoppellaro, G.; Padayattil Jose, S.; Denas, G. Efficacy and safety of rivaroxaban vs. warfarin in high-risk patients with antiphospholipid syndrome: Rationale and design of the Trial on Rivaroxaban in AntiPhospholipid Syndrome (TRAPS) trial. Lupus 2016, 25, 301–306. [CrossRef] Pengo, V.; Denas, G.; Zoppellaro, G.; Padayattil Jose, S.; Hoxha, A.; Ruffatti, A.; Andreoli, L.; Tincani, A.; Cenci, C.; Prisco, D.; et al. Rivaroxaban vs. warfarin in high-risk patients with antiphospholipid syndrome. Blood 2018, 132, 1365–1371. [CrossRef] [PubMed] Pengo, V.; Hoxha, A.; Andreoli, L.; Tincani, A.; Silvestri, E.; Prisco, D.; Fierro, T.; Gresele, P.; Cafolla, A.; De Micheli, V.; et al. Trial of Rivaroxaban in AntiPhospholipid Syndrome (TRAPS): Two-year outcomes after the study closure. J. Thromb. Haemost. 2021, 19, 531–535. [CrossRef] [PubMed] Eikelboom, J.W.; Connolly, S.J.; Brueckmann, M.; Granger, C.B.; Kappetein, A.P.; Mack, M.J.; Blatchford, J.; Devenny, K.; Friedman, J.; Guiver, K.; et al. Dabigatran versus Warfarin in Patients with Mechanical Heart Valves. N. Engl. J. Med. 2013, 369, 1206–1214. [CrossRef] [PubMed] Connolly, S.J.; Karthikeyan, G.; Ntsekhe, M.; Haileamlak, A.; El Sayed, A.; El Ghamrawy, A.; Damasceno, A.; Avezum, A.; Dans, A.M.L.; Gitura, B.; et al. Rivaroxaban in Rheumatic Heart Disease–Associated Atrial Fibrillation. N. Engl. J. Med. 2022, 387, 978–988. [CrossRef] Klok, F.A.; Ageno, W.; Ay, C.; Bäck, M.; Barco, S.; Bertoletti, L.; Becattini, C.; Carlsen, J.; Delcroix, M.; van Es, N.; et al. Optimal follow-up after acute pulmonary embolism: A position paper of the European Society of Cardiology Working Group on Pulmonary Circulation and Right Ventricular Function, in collaboration with the European Society of Cardiology Working Group on Atherosclerosis and Vascular Biology, endorsed by the European Respiratory Society. Eur. Heart J. 2022, 43, 183–189. Bertoletti, L.; Mismetti, V.; Giannakoulas, G. Use of Anticoagulants in Patients with Pulmonary Hypertension. Hämostaseologie 2020, 40, 348–355. [CrossRef] Margelidon-Cozzolino, V.; Delavenne, X.; Catella-Chatron, J.; De Magalhaes, E.; Bezzeghoud, S.; Humbert, M.; Montani, D.; Bertoletti, L. Indications and potential pitfalls of anticoagulants in pulmonary hypertension: Would DOACs become a better option than VKAs? Blood Rev. 2019, 37, 100579. [CrossRef] Humbert, M.; Kovacs, G.; Hoeper, M.M.; Badagliacca, R.; Berger, R.M.F.; Brida, M.; Carlsen, J.; Coats, A.J.S.; Escribano-Subias, P.; Ferrari, P.; et al. 2022 ESC/ERS Guidelines for the diagnosis and treatment of pulmonary hypertension. Eur. Respir. J. 2022, 60, 2200879. [CrossRef] Bhatt, D.L.; Eikelboom, J.W.; Connolly, S.J.; Steg, G.P.; Anand, S.S.; Verma, S.; Branch, K.R.H.; Probstfield, J.; Bosch, J.; Shestakovska, O.; et al. Role of Combination Antiplatelet and Anticoagulation Therapy in Diabetes Mellitus and Cardiovascular Disease: Insights From the COMPASS Trial. Circulation 2020, 141, 1841–1854. [CrossRef] Flumignan, C.D.; Nakano, L.C.; Baptista-Silva, J.C.; Flumignan, R.L. Antiplatelet agents for the treatment of deep venous thrombosis. Cochrane Database Syst. Rev. 2022, 25, CD012369. [CrossRef] Giraud, M.; Catella, J.; Cognet, L.; Helfer, H.; Accassat, S.; Chapelle, C.; Mismetti, P.; Laporte, S.; Mahé, I.; Bertoletti, L. Management of acute venous thromboembolism in patients taking antiplatelet therapy. Thromb. Res. 2021, 208, 156–161. [CrossRef] [PubMed] Poenou, G.; Dumitru Dumitru, T.; Lafaie, L.; Mismetti, V.; Heestermans, M.; Bertoletti, L. Factor XI Inhibition for the Prevention of Venous Thromboembolism: An Update on Current Evidence and Future perspectives. Vasc. Health Risk Manag. 2022, 18, 359–373. [CrossRef] [PubMed] Key, N.S.; Khorana, A.A.; Kuderer, N.M.; Bohlke, K.; Lee, A.Y.Y.; Arcelus, J.I.; Wong, S.L.; Balaban, E.P.; Flowers, C.R.; Francis, C.W.; et al. Venous Thromboembolism Prophylaxis and Treatment in Patients With Cancer: ASCO Clinical Practice Guideline Update. J. Clin. Oncol. 2020, 38, 496–520. [CrossRef] [PubMed] Cells 2022, 11, 3214 17 of 17 114. Farge, D.; Frere, C.; Connors, J.M.; Ay, C.; Khorana, A.A.; Munoz, A.; Brenner, B.; Kakkar, A.; Rafii, H.; Solymoss, S.; et al. 2019 international clinical practice guidelines for the treatment and prophylaxis of venous thromboembolism in patients with cancer. Lancet Oncol. 2019, 20, e566–e581. [CrossRef] 115. Khorana, A.A.; Noble, S.; Lee, A.Y.Y.; Soff, G.; Meyer, G.; O’Connell, C.; Carrier, M. Role of direct oral anticoagulants in the treatment of cancer-associated venous thromboembolism: Guidance from the SSC of the ISTH. J. Thromb. Haemost. 2018, 16, 1891–1894. [CrossRef] 116. Lyman, G.H.; Carrier, M.; Ay, C.; Di Nisio, M.; Hicks, L.K.; Khorana, A.A.; Leavitt, A.D.; Lee, A.Y.Y.; Macbeth, F.; Morgan, R.L.; et al. American Society of Hematology 2021 guidelines for management of venous thromboembolism: Prevention and treatment in patients with cancer. Blood Adv. 2021, 5, 927–974. [CrossRef]