Cell & Molecular Biology - Lesson 1 - PDF
Document Details
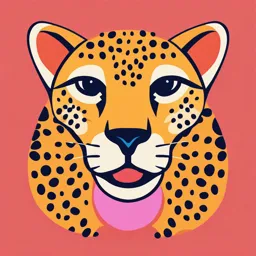
Uploaded by NeatestNovaculite5769
Mindanao State University – General Santos
Tags
Related
- Cell Theory & Microscopy PDF
- General Biology 1: Introduction, Cell Theory & Microscopy PDF
- General Biology 1: Introduction, Cell Theory & Microscopy PDF
- General Biology 1: Introduction, Cell Theory & Microscopy PDF
- Lesson 1 - Microscopy and Cell Theory PDF
- Lecture 2 - Macromolecules, Cell Theory, Microscopy PDF
Summary
This document provides an introduction to the study of cells and molecular biology. It details the discovery of cells through early observations with microscopes and describes different types of microscopes such as bright-field and phase-contrast microscopes.
Full Transcript
LESSON 1: INTRODUCTION TO THE STUDY OF CELL AND MOLECULAR BIOLOGY I. THE DISCOVERY OF CELLS Robert Hooke’s more ornate compound (double‐lens) microscopes. (Inset) Hooke’s drawing of a thin slice of cork, showin...
LESSON 1: INTRODUCTION TO THE STUDY OF CELL AND MOLECULAR BIOLOGY I. THE DISCOVERY OF CELLS Robert Hooke’s more ornate compound (double‐lens) microscopes. (Inset) Hooke’s drawing of a thin slice of cork, showing the omb‐like network of “cells.” ens microscope used by Antonie van Leeuwenhoek to observe bacteria and other microorganisms. The biconvex lens, which pable of magnifying an object approximately 270 times and providing a resolution of approximately 1.35 μm, was held between al plates. THE FIRST OBSERVATIONS 1665 – Robert Hooke observed a thin sliced of cork through a crude microscope - Life’s smallest structural units were “little boxes” or cells - CELL THEORY, all living things are composed of cells 1673 - 1723 – Antonie van Leeuwenhoek was probably the first to observe live microorganisms through the magnifying lenses of the more than 400 microscopes. - He wrote “animacules” - He made detailed drawings of organisms he found in rainwater, feces, and material scraped from teeth. The van Leeuwenhoek microscope DA replica of Antonie van Leeuwenhoek’s Microscope Van Leeuwenhoek’s drawings of bacteria, published in 1684. Even from these simple drawings we can recognize several shaped of Photomicrograph of human blood smear common bacteria: A, C, F and G taken through a van Leeuwenhoek are rod; E is a cocci; H is a microscope. Red blood cells are clearly packets of cocci. apparent. MICROSCOPY - the technical field of using microscopes to view samples & objects that cannot be seen with the unaided eye (first pic) A compound light microscope (second pic) Path of light through a compound light microscope. Besides 10*, ocular lenses are available in 15–30* magnifications. RESOLUTION – (resolving power) the ability of the lenses to distinguish fine detail and structure. Specifically, it refers to the ability of the lenses to distinguish two points that are a specified distance apart. LIGHT MICROSCOPY – refers to the use of any kind of microscope that uses light to observe specimen Several Types of Light Microscopy 1. Compound Light Microscope (LM) - has series of lenses and uses visible light as its source of illumination - examines very small specimens 2. Bright-field Microscope - allows for the observation of living cells, tissues, and other biological specimens for educational and research purposes. - image is formed with “the help” of the light that goes through the specimen and forms the light image against the dark background 3. Phase Contrast Microscope - offers a way to view the structures of many types of biological specimens in greater contrast without the need of stains. 4. Differential Interference Contrast - microscopy technique that introduces contrast to images of specimens which have little or no contrast when viewed using brightfield microscopy. - have a pseudo 3D-effect, making the technique ideal for electrophysiology experiments. 5. Dark-field Microscope - objects are lit at a very low angle from the side so that the background appears dark and the objects show up against this dark background. 6. Fluorescence HOW TO CALCULATE THE TOTAL MAGNIFICATION OF A SPECIMEN? Multiply the objective lens magnification (power) by ocular lens magnification (power) Objective lenses – 10X (low power), 40X (high power), and 100X (oil immersion). Most ocular lenses magnify specimens by a factor of 10 PROBING CELL STRUCTURE: Electron Microscopy Electron Microscopes - use electrons instead of visible light (photons) to image cells and cell structures. In the electron microscope, electromagnets function as lenses, and the whole system operates in a vacuum. - This instrument encompasses both transmission and scanning electron microscope functions. 2 Types of Electron Microscopy 1. Transmission Electron Micrscope (TEM) used to examine cells and cell structure at very high magnification and resolution. The resolving power of a TEM is much greater than that of the light microscope, even allowing one to view structures at the molecular level. resolving power of a TEM is about 0.2 nanometer to view the internal structure of a cell, thin sections of the cell are needed, and the sections must be stabilized and stained with various chemicals to make them visible. To obtain sufficient contrast, the sections are treated with stains such as osmic acid, or permanganate, uranium, lanthanum, or lead salts. 2. Scanning Electron Microscopy (SEM) In scanning electron microscopy, the specimen is coated with a thin film of a heavy metal, typically gold. In the SEM, even fairly large specimens can be observed, and the depth of field (the portion of the image that remains in sharp focus) is extremely good. Electron micrographs taken by either TEM or SEM are originally taken as black and-white images. II. ORGANIZATION AND STRUCTURE OF CELLS A. PROKARYOTIC CELL ORGANIZATION PROKARYOTES - bacteria or green algae, most abundant organisms on earth - a prokaryotic cell does not contain a membrane-bound nucleus - bacteria are either cocci (spheroidal), bacilli (rod like) or spirilla (helically coiled) in shape - fall into two groups, the eubacteria and the archaebacteria BACTERIA (singular: bacterium) are relatively simple, single- celled/unicellular organisms bacterial cells generally appear in one of several shapes some bacteria are star shaped or square Individual bacteria may form pairs, chains, clusters, or other groupings, such formations are usually characteristic of a particular genus or species of bacteria. Enclosed in cell walls that are largely composed of a carbohydrate and protein complex called peptidoglycan. Bacteria are classified according to their cell wall as: Gram-positive (Gram-positive bacteria) - has a thick cell wall surrounding the plasma membrane. The peptidoglycan forms a thick (20- 80 nm) layer external to the cell membrane and may contain other macromolecules. Gram negative (Gram-negative bacteria) - have a thinner cell wall and an outer membrane. The peptidoglycan layer is thin (5-10 nm) and is overlaid by an outer membrane that are lipopolysaccharides and lipoprotein. Between the outer membrane and the cell wall is the periplasm, a space occupied by the proteins secreted by the cell. Bacterial cell walls – the peptidoglycan (protein and oligosaccharide) cell wall protects the prokaryotic cell from mechanical and osmotic pressure. Cell structure - each prokaryotic cell is surrounded by a cell membrane (plasma membrane) which consists of a lipid bilayer containing embedded proteins that control the passage of molecules in and out of the cell and catalyze a variety of reactions. - The cell has no subcellular organelles, only infoldings of the plasma membrane called mesosomes. - The deoxyribonucleic acid (DNA) is condensed within the cytosol to form the nucleoid. - Some prokaryotes have tail-like flagella. Bacteria generally reproduce by dividing into two equal cells (binary fission) For nutrition, most bacteria use organic chemicals, which in nature can be derived from either dead or living organisms. Cell wall structure of gram-negative and gram-positive bacteria ARCHAEBACTERIA Consists of prokaryotic cells Walls lack peptidoglycan Often found in extreme environments 3 Main Groups of Archaebacteria: - Methanogens – produce methane as waste product from respiration - Extreme Halophiles – (halo = salt; philic = loving) live in extremely salty environments such as the Great Salt Lake and Dead Sea - Extreme Thermophiles – (therm = heat) live in hot sulfurous water, such as hot springs at Yellowstone National Park Not known to cause disease in humans B. EUKARYOTIC CELL ORGANIZATION EUKARYOTES - eukaryotic cells have a membrane-bound nucleus and a number of other membrane bound subcellular (internal) organelles, each of which has a specific function - Has 2 Types; the Plant Cell and the Animal Cell ORGANELLES FOUND IN BOTH CELLS 1. Plasma Membrane - surrounds the cell, separating it from the external environment. - Selectively permeable barrier due to the presence of specific transport proteins. - Involved in receiving information when ligands bind to the receptor proteins on its surface, and in the processes of exocytosis (the fusion of secretory vesicles with the plasma membrane) and endocytosis (a cellular process in which substances are brought into the cell). 2. Nucleus - stores the cell’s genetic information as DNA in chromosomes. - bounded by a double membrane but pores in this membrane allow molecules to move in and out of the nucleus. - The nucleolus within the nucleus is the site of ribosomal ribonucleic acid (rRNA) synthesis. 3. Endoplasmic Reticulum - this interconnected network of membrane vesicles is divided into two distinct parts. Rough Endoplasmic Reticulum (RER) - studded with ribosomes - the site of membrane and secretory protein biosynthesis and their post transcriptional modification. Smooth Endoplasmic Reticulum (SER) - involved in phospholipid biosynthesis and in the detoxification of toxic compounds. 4. Golgi Apparatus - a system of flattened membrane-bound sacs, is the sorting and packaging center of the cell. - It receives membrane vesicles from the RER, further modifies the proteins within them, and then packages the modified proteins in other vesicles which eventually fuse with the plasma membrane or other subcellular organelles. 5. Mitochondria - have an inner and an outer membrane separated by the intermembrane space. - Outer membrane is more permeable than the inner membrane due to the presence of porin proteins. - Inner membrane, which is folded to form cristae, is the site of oxidative phosphorylation, which produces ATP. - The central matrix is the site of fatty acid degradation and the citric acid cycle. 6. Cytosol - Is the soluble part of the cytoplasm where a large number of metabolic reactions take place. - Within the cytosol is the cytoskeleton, a network of fibers (microtubules, intermediate filaments, and microfilaments) that maintain the shape of the cell. 7. Peroxisomes - contain enzymes involved in the breakdown of amino acids and fatty acids, a by-product of which is hydrogen peroxide. This toxic compound is rapidly degraded by the enzyme catalase, also found within the peroxisomes. ORGANELLES ONLY FOUND IN THE ANIMAL CELLS - Have gap junctions and centrioles - Have glycogen for energy storage 1. Centrosomes or Pair of Centrioles - self-replicating organelles made up of nine bundles of microtubules 2. Lysosomes - lysosomes in animal cells are bounded by a single membrane. - They have an acidic internal pH (pH 4-5), maintained by proteins in the membrane that pump in H+ ions. - Within the lysosomes are acid hydrolases, enzymes involved in the degradation of macromolecules, including those internalized by endocytosis. ORGANELLES ONLY FOUND IN THE PLANT CELLS - Have a plasmodesmata - Undergoes photosynthesis - During mitosis, a cell plate is formed in plant cells - Uses starch for energy storage - Large central vacuole stores more water and carbohydrates then animal cell vacuoles 1. Cell Wall - a rigid covering that protects the cell, provides structural support, and gives shape to the cell - made up of the polysaccharide cellulose. - woody plants have a phenolic polymer called lignin which gives the cell wall additional strength and rigidity. 2. Chloroplasts - surrounded by a double membrane and have an internal membrane system of thylakoid vesicles (contains chlorophyll and are the site of photosynthesis) that are stacked up to form grana. - Carbon dioxide (CO2) fixation takes place in the stroma, the soluble matter around the thylakoid vesicles. 3. Plant Cell Central Vacuole - the membrane- bound vacuole is used to store nutrients and waste products, has an acidic pH and, due to the influx of water, creates turgor pressure inside the cell as it pushes out against the cell wall. PROKARYOTES VS EUKARYOTES PROKARYOTES EUKARYOTES DNA In “nucleoid” Region Within membrane-bound nucleus SIZE Usually, smaller Usually, larger ORGANIZATION Usually single-celled Often multicellular METABOLISM May not need oxygen Usually need oxygen to exist ORGANELLES No membrane-bound organelles Membrane-bound organelles III. EARLY SCHOOLS OF THOUGHT How should we study biological processes? A. VITALISM Intact cells possess a vital force one must study an intact cell B. MECHANISM A cell should be dissected like a machine C. HOLISM The whole is greater thant the sum of its parts D. REDUCTIONISM All biological phenomena can be reduced into molecules and atoms (the laws of physics and chemistry) IV. MOLECULAR BIOLOGY MOLECULAR BIOLOGY (William Astbury, 1845) ▪ The study of the chemical and physical structure of biological macromolecules BIOCHEMISTRY Structural root Physical and chemical structure of biomolecules Nucleic acids, proteins, lipids, carbohydrates MICROBIAL GENETICS Informational Root Information on the genetic material, its transmission and expression Simple systems, i.e. phages and bacteria MOLECULAR BIOLOGY (present day definition) ▪ The study of genes and their products and how these products function and interact in the orgnization and perpetuation of living things PROBLEMS FACED IN THE PAST 1. What is a genetic material? 2. How are macromolecules (especially proteins) synthesized? 3. How does DNA replicate? 4. How is information obtained from DNA? 5. How is gene expression regulated? 6. How are cellular substances transported in and out of the cell? 7. How do specialized cell work? (e.g. muscle cells and nerve cells) 8. What is the molecular basis of bioogical phenomena? Aging Development Immune reaction Cancer and other human diseases APPROACHES TO PROBLEMS 1. Correlation of structure and function Examples Structure Function Collagen (tendon protein) Triple Stranded Aggregate for additional strength DNA Double-stranded specific base pairing Stability and replicability Cell Membrane Non-polar fatty acids Prevents polar molecules to freely pass through 2. Physical Measurements Using methods like: a. Ultracentrifugation b. Electrophoresis c. Infrared absorption spectroscopy 3. In vitro approach Crude extracts and reconstituted systems THE LOGIC OF MOLECULAR BIOLOGY Arguments are based on: A. Efficiancy - Living cells have had millions of years to evolve – e.g. competition and survival selected for efficiency – In biological processes, little energy and material are wasted. Ex. Gene expression B. Development and evaluation of MODELS - Tentative explanation of how a system works - Tested of validity Ex. C. Strong Inference - Based on observations, facts and data - Inductive methods to draw conclusions Initial Observation → Hypothesis → Experiments → Results → Conclusion → Theory LESSON 2: CELLS AND GENOMES I. THE CELL THEORY ▪ Cells are the basic structural and functional units of life Matthias Schleiden and Theodore Schwann (1838 – 1839) - All organisms are composed of one or more cells. - The cell is the structural unit of life. Rudolf Virchow (1855) - Cells can arise only by division from a preexisting cell. ▪ Living things, though infinitely varied when viewed from the outside, are fundamentally similar inside ▪ Heredity and variation ▪ The cell carries all hereditary information (i.e. DNA) that defines each species 1.1 All cells store their hereditary information in the form of DNA - DNA is a long, unbranched, double-stranded molecule formed by four types of nucleotides (discrete pair of nucleotides in order to have fidelity and accuracy to the DNA) - Each nucleotide has a phosphate, a sugar, and a base - Covalent bonds link the nucleotides in each strand while H-bonds hold the two DNA strands together. - The sequence of bases determines the genetic information. FREDERICK GRIFFITH EXPERIMENT (1928) - Transformation experiment on Diplococcus pneumoniae (or known as Streptococcus pneumoniae) Possible Explanations: a. R types caused dead S types to come back to life b. Information from the heat-killed S type was transferred to the live R type. RELATED EXPERIMENTS 1. M.H. Dawson (1930) and J.L. Alloway (1932) – different experiments but same process and outcome - did an in vitro transformation which did not used mice - transformation of the avirulent R type to the virulent S type - a transforming principle or agent is responsible 2. O. Avery, C. MacLeod, M. McCarty Experiment a. General Properties of Transforming substance 1. Viscous, silky sheen 2. Loses activity in distilled water 3. Activity retained for months in physiological salt solution 4. Withstands heating at 65C for 30-60 minutes 5. Loses activity with increased acid concentration HERSHEY-CHASE BLENDER EXPERIMENT In 1951 and 1952, Alfred Hershey and Martha Chase performed their experiments, later named the Hershey-Chase experiments, on viruses that infect bacteria, also called bacteriophages. The Hypothesis of the Hershey and Chase blender experiment was that DNA, rather than protein, is the genetic material responsible for transmitting hereditary information. This hypothesis was based on previous knowledge about the composition of bacteriophages and the roles of DNA and protein in cellular processes. Hershey and Chase utilized a technique called radioactive isotope labeling. Hershey and Chase marked phages by incorporating radioactive isotopes of phosphorus and sulfur in those phages. They allowed some phages to replicate by infecting bacteria, specifically Escherichia coli, or E. Coli, that scientists had grown in radioactive sulfur. The researchers let other phages infect and replicate in E. Coli that scientists had grown in radioactive phosphorus. DNA contains phosphorus, but not sulfur, whereas protein contains sulfur, but not phosphorus. Bacteriophages produced within bacteria growing in radioactive culture medium will themselves be radioactive. If radioactive sulfur atoms (35S) are present, they will be incorporated into the protein coats of the bacteriophages since two of the amino acids — cysteine and methionine — contain sulfur. However, the DNA will be nonradioactive because there are no sulfur atoms in DNA. If radioactive phosphorus ( 32P) is used instead, the DNA become radioactive — because of its many phosphorus atoms — but not the proteins. Using ultra centrifugation, they were able to extract the pellet (the solid part of extracted in the centrifuge) containing the 32P and the supernatant (the liquid part in the extract) containing 35S and they found out that when bacteriophages containing 32P (radioactive), were allowed to infect nonradioactive bacteria, all the infected cells became radioactive and, in fact, much of the radioactivity was passed on to the next generation of bacteriophages. However, when the bacteria were infected with bacteriophages labeled with 35S, and then the virus coats removed (by whirling them in an electric blender), practically no radioactivity could be detected in the infected cells. CRUCIAL DATA FROM OTHER RESEARCHES 1. Phoebus Levene (a Russian-American biochemist) discovered that DNA is made of nucleotides (phosphate, sugar, and the ATCG) 2. Erwin Chargaff said that 1 purine (adenine and guanine) is equivalent to 1 pyrimidine (thymine and cytosine) Rollin Hotchkiss and Ronald Fischer conducted related experiments to Erwin Chargaff 3. Linus Pauling suggested that the DNA is triple-stranded finding out later that it was collagen and not DNA. 4. Maurice Wilkins and Rosalind Franklin provided X-ray diffraction photograph of crystalline DNA which later helped 5. James Watson and Francis Crick (1953) in defining that the DNA is a double helix structure with two long helical strands twisted together 1.2 All cells replicate their DNA by templated polymerization DNA is synthesized using a template formed by a preexisting DNA strand. The nucleotide sequence in one strand is complementary to the other strand DNA synthesis occurs in a semi-conservative manner. (semi-conservative in a sense that it keeps the one part of the parent strand and pairs with a new strand) 1) Semiconservative Model - after one round of replication, every new DNA double helix would be a hybrid that consisted of one strand of old DNA bound to one strand of newly synthesized DNA. Then, during the second round of replication, the hybrids would separate, and each strand would pair with a newly synthesized strand. Afterward, only half of the new DNA double helices would be hybrids; the other half would be completely new. Every subsequent round of replication therefore would result in fewer hybrids and more completely new double helices. 2) Conservative Model - after one round of replication, half of the new DNA double helices would be composed of completely old, or original, DNA, and the other half would be completely new. Then, during the second round of replication, each double helix would be copied in its entirety. Afterward, one-quarter of the double helices would be completely old, and three-quarters would be completely new. Thus, each subsequent round of replication would result in a greater proportion of completely new DNA double helices, while the number of completely original DNA double helices would remain constant. 3) Dispersive Model - every round of replication would result in hybrids, or DNA double helices that are part original DNA and part new DNA. Each subsequent round of replication would then produce double helices with greater amounts of new DNA. The Meselson-Stahl Experiment Meselson and Stahl Experiment was an experimental proof for semiconservative DNA replication. In 1958, Matthew Meselson and Franklin Stahl conducted an experiment on E. coli which divides in 20 minutes, to study the replication of DNA. Sample no. 1 (after 20 minutes): The sample had bacterial DNA with an intermediate density. Sample no. 2 (after 40 minutes): The sample contained DNA with both intermediate and light densities in the same proportion. Based on observations and experimental results, Meselson and Stahl concluded that DNA molecules can replicate semi-conservatively. Investigation of semi- conservative nature of replication of DNA or the copying of the cells, DNA didn’t end there. Followed by Meselson and Stahl experiment, Taylor and colleagues conducted another experiment on Vicia faba (fava beans) which again proved that replication of DNA is semi-conservative. Straight or Circular? Following publication of Meselson and Stahl's results, many scientists confirmed that semiconservative replication was the rule, not just in E. coli, but in every other species studied as well. To date, no one has found any evidence for either conservative or dispersive DNA replication. Scientists have found, however, that semiconservative replication can occur in different ways—for example, it may proceed in either a circular or a linear fashion, depending on chromosome shape. In fact, in the early 1960s, English molecular biologist John Cairns performed another remarkably elegant experiment to demonstrate that E. coli and other bacteria with circular chromosomes undergo what he termed theta replication, because the structure generated resembles the Greek letter theta (Θ). Specifically, Cairns grew E. coli bacteria in the presence of radioactive nucleotides such that, after replication, each new DNA molecule had one radioactive (hot) strand and one nonradioactive strand. He then isolated the newly replicated DNA and used it to produce an electron micrograph image of the Θ-shaped replication process 1.3 Gene is a DNA fragment corresponding to one protein or RNA Genome dictates the nature of proteins, when and where they are to be made Gene expression is a regulated process Coding segments specify the transcripts and protein products Non-coding regions serve as punctuations and regulatory sequences that control the local rate of transcription. Long non-coding RNA is ~1000 – 10 000 nt (nucleotides) long with little to no protein-coding potential 1.4 All cells transcribe portions of their DNA into RNA RNA is a single-stranded polynucleotide closely related to DNA. o Sugar: ribose o Bases: A, G, C, U RNA molecule is flexible and can fold up into specific shapes The shape of RNA may enable it to recognize and selectively bind molecules, even catalyze some chemical changes Transcription is a form of templated polymerization that faithfully rewrites DNA to RNA Noncoding strand serves as the template to produce RNA transcripts In RNA… o Upper strand is called sense strand or coding, this is the result from the mRNA produced by the anti-sense. o Lower strand or downward is called anti-sense or non-coding, this becomes a template for making mRNA In DNA… o The sense strand is used for synthesizing mRNA and are generated as complementary strand during DNA semi- conservative replication o The anti-sense strand is the template used for DNA replication and can't be used for transcription These transcripts function as intermediates in the transfer of genetic information Messenger RNA (mRNA) guides the synthesis of proteins 1.5 All cells translate RNA into protein in the same way Proteins are long, unbranched polymers of amino acids and are the main molecules that put the cell’s genetic information into action. Proteins are implementers of the genomic code. DNA sequence specifies the amino acid sequence, which in turn determines the function of the protein product. There are 20 types of amino acids, each built around the same core structure where a specific side chain attaches giving the protein a distinct chemical property 1.6 All cells use proteins as catalysts Catalytic protein folds into a specific conformation forming reactive sites → enzymes 1.7 All cells require and utilize energy ATP as the energy currency in cells Sources of free energy 1.8 Cells acquire free-energy from various sources Living organisms obtain their free energy in different ways Organotrophic organisms feed on other living things or the organic chemicals they produce Phototrophic organisms harvest sunlight and convert it into chemical forms that can be used by the cells Lithotrophic organisms capture their energy from energy-rich systems of inorganic chemicals in the environment. 1.9 All cells are enclosed in a plasma membrane across which nutrients and waste materials must pass Plasma membrane acts as a selective barrier Membrane transport proteins determine which molecules enter the cell Amphiphilic molecules aggregate spontaneously to form a bilayer in water Cells produce molecules whose chemical properties cause them to self-assemble into the structures that a cell need 1.10 Cells respond to stimuli and self-regulate Cells are covered with receptors that interact with substances in the environment in highly specific ways Receptors provide pathways through which external stimuli can evoke specific responses in target cells Cells may respond to specific stimuli by altering their metabolic activities, moving from one place to another, or even activating death pathways Cells are robust, under constant regulation Cells use specific feedback circuits to regulate cellular responses to maintain homeostasis LESSON 3: NUCLEIC ACIDS I. NUCLEIC ACIDS Informational molecule of all loving organisms Long, chain-like polymers assembled from repeating sub-units, the nucleotides. 2 TYPES OF NUCLEIC ACIDS 1. Deoxyribonucleic Acid 2. Ribonucleic Acid NUCLEOSIDE a unit of only the base and sugar without phosphates NUCLEOTIDE made up of a nitrogen-containing base, a phosphate group, and a sugar molecule both nucleotide and nucleoside can be anti- cancer or anti-viral synthetic analogs from some nucleotides to be a pathogen or to be manufactured as a drug ex. ATP is an example of nucleotide 3 MAIN COMPONENTS OF NUCLEOTIDES 1. NITROGENOUS BASES a) Pyrimidines - 6 membered rings made up of carbons and nitrogen atoms o Uracil (U) – 2,4-dioxypyrimidine o Thymine (T) – 2,4-dioxy-5-methylpyrimidine or 5-methyluracil o Cytosine (C) – 2-oxy-4-aminopyrimidine b) Purines - a 6-membered ring fused to a 5-membered ring o Adenine (A) – 6-aminopurine o Guanine (G) – 2-amino-6-oxypurine TAUTOMERIC SHIFT in DNA Bases (Tautomerization) Reversible change of one DNA base isomer to another shifts in the location of H atoms and double bonds Tautomers - 2 forms of the same molecule differing only in the location of a proton and a double bond In DNA bases... usually, amino (NH2) form and keto (C=O) rarely imino (NH) form and enol (C-OH) if H atom had no fixed positions the A could pair with C and G could pair with T 2. FIVE-CARBON SUGAR (PENTOSE) - links covalently to nitrogenous base Types of Sugar -D-Ribose -D-Deoxyribose 3. Phosphate Group - a chain of 1,2 or 3 phosphates linked to the pentose sugar at its 5'-carbon CHEMICAL BONDS in the DNA 1. Numerous hydrogen bonds between base pairs in the interior of the molecule Factors Affecting DNA Stability 2. Covalent Bonds -N-glycosidic bond (sugar to base) Phosphodiester bond (nucleotide to nucleotide) PRIMARY STRUCTURE OF THE DNA 1. Two-stranded 2. Sugar-phosphate backbone on the outside 3. Bases (specific pairing and sequence) 4. Each strand is a string of nucleotides 5. Two complementary strands are held by H-bonds SECONDARY STRUCTURE OF THE DNA 1. Two intertwined antiparallel strands of nucleotides (meaning it has specific diameter, distance and 1 turn = 10 base pair) 2. Major and Minor Grooves which is essential for binding of proteins 3. Right-handed helix - Looking down on the central axis, the strands follow a clockwise path, away from the observer 4. Helix has a constant diameter of 20 Å (Angstrom) - The space separating the sugar-phosphate backbone of a DNA double helix is just wide enough to accommodate a purine-pyrimidine base pair 5. Bases are perpendicular to helix axis. - Bases are planar and stacked on top of each other. - 10 base pairs in 1 complete turn - 3.4 Å is the distance between base pairs 6. Hydrophobic associations between the base pairs in the interior of the molecule forming a stable non-polar environment a) Schematic representation b) Stick representation c) Space filling model DNA CONFIRMATIONS - Several confirmations exist (A, B, C, D, E, Z,...) Helix Type A B C Z Overall proportion Short and broad Long and thin Long and thin Elongated and slim Helix rotation right right right left Base pair per turn 11 10 9.33 12 Conditions of 75% hum K+, Na+, 92% hum low salt 66% hum - occurrence Ca++ Helix diameter 23 Å 20 Å 20 Å 18 Å Major groove Extremely, narrow, Wide, intermed - Flattened out on helix very deep surface Minor groove Very broad, shallow Narrow, intermed - Extremely narrow, very deep Found in dsRNA, RNA-DNA Biologically In vitro, dehydrated dCGCGCG, in vitro, duplexes, rRNA, dominant, conditions in vivo, methylated tRNA, dsRNA, viral polypyrimidine strand DNA RNA, polypurine strand RIBONUCLEIC ACID (RNA) Exist largely as single-stranded chains in living cells It's sugar is called ribose Bases are C, G, A and U (Uracil) Genetic material of some viruses Segments of RNA molecules may: o form RNA-DNA hybrid o pair temporarily in double-helical form o fold back on themselves to set up extensive double-helical regions RNA whose single strands are not perfectly complementary → form a double-stranded RNA at certain regions PECULIAR NUCLEIC ACID SEQUENCES 1. Mirror Repeat - symmetrical sequence within each strand 2. Inverted Repeats or Palindrome - two copies of an identical sequence present in the reverse orientation MORE SECONDARY STRUCTURES OF DNA 1. Internal Loops - when there are bases that cannon pair on both sides of the helix 2. Bulge Loops - unpaired bases occur only on one side of the helix 3. Cruciform - in DNA with a region of dyad symmetry in which the axis of symmetry separates the inverted repeats 4. Hairpin - in single stranded DNA molecule with an inverted repeat or in an RNA molecule copied from a palindromic DNA - when it folds back on itself to form a double-helical segment in the region of the sequence 5. Stem-and-Loop Structure - similar with that of the hairpin but occurs when the loop at the end would have many unpaired bases - plays an important role in attenuation 6. Cloverleaf Structure - the characteristic confirmation of the tRNA molecule - made up of three stem-loops - has four arms SIGNIFICANCE OF OTHER 2° or 3° STRUCTURE IN DNA/RNA: 1) Means of stabilizing genome structure (e.g. the single stranded DNA of some viruses form hairpins or lollipop structures) 2) Serve as recognition site for initiation of DNA replication in single stranded DNA viruses (palindromes at the ori-site) 3) Serve as a signal for the initiation and termination of transcription (operator and terminator regions have protrusions recognized by proteins) 4) Important in the function of the Nucleic Acid made from it (e.g. cloverleaf tRNA complex folding of rRNA) 5) Form the core of catalytic RNA molecules (ribozymes) as binding sites for the substrates and cofactors LESSON 4: PROPERTIES OF GENETIC MATERIAL I. PROPERTIES OF GENETIC MATERIAL → genetic material must and should be F.A.I.R.S F – flexible/respond to external signals A – altered/generate genetic diversity I – information R – replicated and transmitted accurately S – stable (very) PACKING OF EUKARYOTIC DNA Chromosomes Composed of DNA and associated proteins organized into genes Chromatin → mixture of DNA and associated proteins that forms the chromosome 2 TYPES OF PROTEINS IN CHROMATIN a. Histones – small, well defined, basic proteins. Also called as octamer. b. Non-histone chromosomal protein – include diverse structural, enzymatic and regulatory proteins Chromatid → one of the two identical halves of a chromosome. The two “sister” chromatids are joined at a constricted region called centromere. Centromere → divides the chromosome into two sections, or “arms.” The short arm of the chromosome is labeled the “p arm.” The long arm of the chromosome is labeled the “q arm.” Telomere → a region of repetitive DNA sequences at the end of a chromosome. It protects the ends of chromosomes from becoming frayed or tangled. Each time a cell divides, the telomeres become slightly shorter leading to apoptosis or senescence, affecting the health and lifespan of an organism. The Nucleosome Repeating units of chromatin composed of DNA and histones Around 147 base pairs supercoiled DNA wrapped twice around a histone Octamer → two copies each of histones (a) a nucleosome core particle viewed; (b) the disk shape of the nucleosome core particle; (c) a simplified, schematic model of half of a nucleosome core particle Histone H1 - Referred to as linker histone and is associated with linker DNA - A segment of the DNA connects one nucleosome to the next - H1 runs down the center of the coil acting as a stabilizer Octamer Histones (H2A to H4) - Highly conservative sequence (meaning once a mutation takes place in that area protein function will be affected and could cause apoptosis) Levels of Chromatin Packing i. “Beads on as string” - Nucleosome beads in chromatin isolated from an erythrocyte nucleus ii. Chromatin Fiber - Approximately 30nm thick - Structure of fiber remains a subject of debate thus having 2 models: iii. Looped Domain iv. Small portions of the mitotic chromosome II. NUCLEIC ACID ISOLATION APPLICATIONS AND PURPOSE OF NA EXTRACTION High quality DNA is obtained for other applications/analyses o Restriction digestion - uses molecular scissors or restriction enzymes or endonucleases - to check certain features in a certain gene of interest o Gene cloning - Get the same exact copy in the gene of interest o Mutagenesis - How mutation is occurring in a certain organism and genotyping o Amplification - To make a lot of copy of gene of interest o DNA sequencing - Just to see what happens if you do something with a gene DNA Profiling – compare the DNA sequence of one organism to another Molecular Biotechnology Phylogenetic Studies – trace the diversification to identify the evolutionary process of organisms COMMON SOURCES OF DNA Whole blood Nails Buccal (cheek) swabs Bacterial cultures Hair Tissues Epithelial cells Fungal cultures Sperm Blood stains Urine Animal tissues Bones Saliva Paper cards with collected sample Plants GENERAL STEPS IN NUCLEIC ACID ISOLATION 1. Tissue Homogenization and Cell Lysis Mechanical Method a) Sonication - process of applying sound energy to agitate particles or discontinuous fibers in a liquid b) Grinding – in this process, we will grind the liquid nitrogen to help the sample to be brittle Chemicals for Extraction a) Buffer (e.g. Tris-HCL or Tris hydrochloride) – resist drastic change when upon addition of an acid or base in a narrow range. To maintain the pH level and stabilize. b) Salt (e.g. NaCL or Potassium acetate) – to neutralize the DNA and make it cluster in a certain area and make a compact DNA and separate it from other cellular components c) Cell Lysis Reagents (e.g. SDS or Sodium dodecyl sulfate) – a surfactant to break lipids d) Denaturants (e.g. guanidium) – it inactivates RNAses or DNAses depending on what you want to extract Enzymatic treatment (e.g. lysozyme, cellulase, pectinase) – lose all the enzymes that are not needed 2. Denaturation and separation of other biomolecules from the nucleic acid Chemical Treatment a) Phenol – denatures proteins b) Chloroform – removes proteins and lipids c) Isoamyl alcohol – removes phenol and chloroform as it could affect the purity of the DNA d) CTAB (cetyltrimethylammonium bromide) – removes polysaccharides e) PVP (polyvinylpyrrolidone) – removes polyphenols which can be seen in plants Enzymatic Treatment – to make sure that proteins are completely remove Centrifugation 3. Precipitation of nucleic acid from aqueous phase Apply monovalent cations - sodium, potassium - apply salt to neutralize negative charge DNA – so that no repulsion will happen – with a mixture of alcohol Alcohol - Ethanol 95% - Absolute 99% - isopropanol Centrifugation 4. Washing of precipitated nucleic acid Wash it with 70% alcohol Centrifugation – may be done for two to three times - To make sure that the DNA is totally clear from debris - Dehydrate the DNA or to remove excess water to preserve the DNA for a long time 5. Drying of pellet and dissolution of dried pellet Air drying or vacuum drying Dissolution in sterilized molecular water or TE (Tris-EDTA) EDTA – inactivates DNAses for it to not degrade the DNA - For storage you store it in a -20C to -80C storage and if you need to thaw it to make it warm and is up for usage - there are commercially available kits where all the solutions and chemicals are already prepared After isolation RNAses treatment if DNA is isolated DNAses treatment if RNA is isolated Storage: - stock solution at -20C to -80C - working solution at 4C Determination of purity and concentration - using a DNA standard for the positive control (different concentration) - UV spectroscopy Gel Electrophoresis Determination of Purity and Quantity Requirement: UV spectrophotometer Steps: 1. To assess purity: a) Take readings at 260 nm (nanometer) and 280 nm b) Get ratio A260/A280 (A – absorbance of a solution) A260/A280 = 1.8 – 2.0 high purity for Nucleic Acid If A260/A280 is: