Cell Bio 2 PDF
Document Details
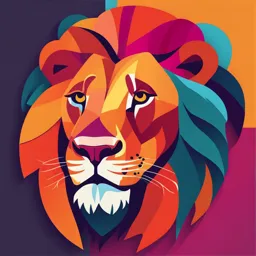
Uploaded by FirstRateIntegral
Tags
Summary
This document discusses the theories of membrane structures, including the Overton, Gorter-Grendell, Danielli-Davson, and fluid mosaic models. It covers concepts like unit membrane models, lipid composition, and membrane fluidity. The focus is on biological membrane structure and function.
Full Transcript
Theories on membrane structures 1902 it was thought that the membranes had only lipids (Overton). In 1926 Gorter and Grendell proposed that lipids are capable of forming a double layer. In 1935 Danielli and Davson proposed the lipid bilayer model that includes proteins...
Theories on membrane structures 1902 it was thought that the membranes had only lipids (Overton). In 1926 Gorter and Grendell proposed that lipids are capable of forming a double layer. In 1935 Danielli and Davson proposed the lipid bilayer model that includes proteins adhering to both lipid-aqueous interfaces Artificial model systems such as the liposomes supported the idea of DanielliandDevson. A droplet of lipid made soluble in an organic solvent can be spread over a small hole on a septum that divides two chambers containing water. This set up is useful to study biophysical properties of a bilayer such as permeability and electrical resistance. Channels for ions can be formed by adding certain proteins or polypeptides. Liposomes act as excellent carriers for different molecules such as chemotherapeutic compounds, insulin and antibodies. Unit membrane model Robertson in 1959 postulated the unit membrane model. This model stated that the central layer of plasma membranes is made up of hydrocarbon chains of lipids and the proteins constitute the dense surrounding layers on both sides when viewed through an electronmicroscope. Unit membrane model turned out to be an over simplification model as it can’t account for the number of protein molecules present across the membranes Fluid mosaic model Fluid mosaic model proposed by S.J. Singer and G.L. Nicolson (1972) was finally acceptable to most biologists This model recognizes that lipids and proteins are in a mosaic arrangement. It also recognizes that there is translational movement of lipids and proteins within the lipid bilayer. Non covalent interactions ensure a fluid like state for the membranes. Integral proteins are intercalated into the continuous lipid bilayer. Polar/hydrophilic regions of proteins protrude from the surface while the nonpolar/hydrophobic regions are embedded inside Key Concepts Major functionsof lipids: energy storage, major membrane components - Other functions: signals, electron carriers, emulsifying agents. Membrane lipids (amphipathic) -- responsible for spontaneous formation of lipid bilayers a. Glycerophospholipids: glycerol backbone + 2 fatty acyl "tails" in ester linkage + a polar "head group” (a phosphate ester of another alcohol like choline, ethanolamine, serine, inositol, etc.) a. Sphingolipids: sphingosine backbone (1 "tail") + fatty acid chain in amide linkage (another "tail") + either carbohydrate (glycosidic bond to sphingosine) or phosphate ester of another alcohol like choline or ethanolamine (ester bond to sphingosine) - glycosphingolipids(cerebrosides, gangliosis) - phosphosphingolipids(sphingomyelins) a. Cholesterol Membrane fluidity (vital to membrane function) depends on lipid composition of bilayer. - fatty acid chainlength (more C atoms ^ more packing of tails, less fluidity) - fatty acid numbers of double bonds (fewer double bonds ^ more packing of tails, less fluidity) - cholesterol content ("buffers" fluidity) Biological Membranes sheet-like structures, a few molecules thick, forming closed boundaries (self-sealing) -amphipathic lipids: polar "head" groups and nonpolar "tails” -amphipathic lipids form bilayers instead of micelles. -Proteins carry out most of the specific functions. -carbohydrates (covalently attached to lipids = glycolipids, or to proteins = glycoproteins) - important in communication/recognition non-covalent assembly (interactions between components) into a fluid.oteins and lipids can diffuse rapidly in plane of membrane, but Proteins and lipids do not rotate across the membrane (no "flip- flop" in orientation across membrane). Membranes always synthesized by growth of preexisting membranes Amphipathic nature of membrane lipids hydrophilic portion and hydrophobic portion hydrophilic portion = "head"; hydrophobic chain(s) = "tails" Consequence: Amphipathic lipids form micelles or bilayers, to bury their hydrophobic tails so they're NOT exposed to H2O, but keep the hydrophilic head groups in contact with H2O. Lipids with single hydrophobic tails can form micelles, but Membrane lipids almost all have 2 tails, and thus form bilayers. Bilayers curve around and seal edges ^ closed vesicles (liposomes). The hydrophobic effect provides the major driving force for the formation of lipid bilayers. Bilayer structure stabilized by hydrophobic effect (the driving force for their formation) Hydration of polar/charged head groups Van der Waals interactions (packing between atoms in hydrophobic core) Hydrophobic core of the membrane is like a nonpolar solvent. Permeability coefficients correlated with solubility in nonpolar solvent relative to solubility in H2O. highly impermeable to ions and most polar molecules more permeable to nonpolar species Liposomes lipid vesicles, aqueous compartments enclosed by a lipid bilayer experimental tools for studying membrane permeability vehicles for delivery to cells of chemicals/drugs/DNA for gene therapy Membrane Functions 1. highly selective permeability barriers a. regulate molecular & ionic compositions of cells and intracellular organelles b. channels and pumps (proteins that act as selective transport systems) c. electrical polarization of membrane (inside of plasma membrane negative, typically - 60 millivolts) d. maintain different ionic concentrations on opposite sides of membrane. 2. INFORMATION PROCESSING - Biological communication a. signal reception by specific protein receptors (Binding) b. transmission/transduction of signals (via protein conformational changes sometimes generation of signals, chemical or electrical, e.g.,nerve impulses 3. ENERGY CONVERSION - ordered arrays of enzymes and other proteins and organization of reaction sequences a. photosynthesis (light energy ^ chemical bond energy): inner membranes of chloroplasts, and plasma membranes of some prokaryotes b. oxidative phosphorylation (oxidation of fuel molecules ^ chemical bond energy "stored" in ATP): inner membranes of mitochondria, and plasma membranes of prokaryotes MEMBRANE FLUIDITY Controlled by lipid composition Hydrocarbon chains: close packing, maximum interaction between chains at low temperatures ^ rigid "gel"; the longer the chains and the more saturated (fewer double bonds), the more ordered/rigid the state of the lipid bilayer Above transition temperature, lipid bilayer undergoes phase change ("melting") to more disorderly, FLUID state (chains not so closely packed). Transition temperature is lowered (so relative fluidity increases) by fatty acid structures that reduce favorable packing interactions. Shorter hydrocarbon chainlength, and/ormore double bonds (which make "bends" in the chain) Highly ordered packing of fatty acid side chains (stabilized by lots of close van der Waals interactions) is disrupted by cis double bonds (kinks). With more double bonds, membrane remains fluid at lower temperatures (transition temp. is lowered). Regulation of Membrane Fluidity Membranes of living cells must be fluid - Must have transition temperatures belowbody temperature of the organism. Regulation of fluidity (especially in organisms that don’t rigorously control their body temperature) by lipid composition: Fatty acid chainlength(shorter ^ more fluid) Number of double bonds (more d.b. ^ more fluid) Cholesterol (animal cells) "stiffens" membrane by packing between unsaturated HC tails, but also disrupts close packing between saturated tails, so broadens the transition sort of like a fluidity "buffer", when temperature or fatty acid composition changes. Membrane Proteins Three types based on association with membrane: 1. Peripheral 2. Integral 3. Lipid-anchored Peripheral Weakly associated with membrane at surface Bind to polar lipid heads and/or to integral membrane proteins Electrostatic interactions predominate (ionic bonds and/or hydrogen bonds) Easily extractable from membranes by high salt concentrations (disrupting electrostatic interactions), or by EDTA (chelates Ca 2+ and Mg2+) Usually water-soluble (globular) There can also be fibrous proteins attached to membrane surface (cytoskeletal proteins). Some peripheral "membrane" proteins come and go from membrane, e.g., using reversibly attached lipid anchor. Covalently attached C14 fatty acyl chain (myristoyl group) slips into lipid bilayer, holding protein to surface of membrane. Transmembrane Proteins How does an integral membrane protein accommodate its polar backbone (peptide N-H and C=O groups) in a stable way across hydrophobic core of lipid bilayer? By hydrogen-bonding as many as possible of polar backbone groups (really ALL of them), i.e., by forming secondary structures, either a-helical or p barrel motifs for membrane-spanning parts of transmembrane protein Glycophorin A (erythrocyte membranes) Bacteriorhodopsin (purple membrane of Halobacteriumhalobium, a salt- loving bacterium) Prostaglandin H2 synthase (COX, enzyme involved in biosynthesis of prostaglandins/inflammatory response) Porins(channel-forming proteins -- outer membranes of gram-negative bacteria and outer membranes of mitochondria and chloroplasts) Membrane Transport Diffusion Diffusion is the net passive movement of particles (atoms, ions or molecules) from a region in which they are in higher concentration to regions of lower concentration. It continues until the concentration of substances is uniform throughout. Some major examples of diffusion in biology: Gas exchange at the alveoli — oxygen from air to blood, carbon dioxide from blood to air. Gas exchange for photosynthesis — carbon dioxide from air to leaf, oxygen from leaf to air. Gas exchange for respiration — oxygen from blood to tissue cells, carbon dioxide in opposite direction. Transfer of transmitter substance — acetylcholine from presynaptic to postsynaptic membrane at a synapse. Osmosis — diffusion of water through a semipermeable membrane. High Diffusion Rate: Short distance, large surface area, big concentration difference (Fick’s Law). High temperatures increase diffusion; large molecules slow diffusion. Facilitated Diffusion This is the movement of specific molecules down a concentration gradient, passing through the membrane via a specific carrier protein. Thus, rather like enzymes, each carrier has its own shape and only allows one molecule (or one group of closely related molecules) to pass through. Selection is by size; shape; charge. Common molecules entering/leaving cells this way include glucose and amino-acids. It is passive and requires no energy from the cell. If the molecule is changed on entering the cell (glucose + ATP ^ glucose phosphate + ADP), then the concentration gradient of glucose will be kept high, and there will a steady one-way traffic. Osmosis Osmosis is a special example of diffusion. It is the diffusion of water through a partially permeable membrane from a more dilute solution to a more concentrated solution - down the water potential gradient) Note: diffusion and osmosis are both passive, i.e. energy from ATP is not used. A partially permeable membrane is a barrier that permits the passage of some substances but not others; it allows the passage of the solvent molecules but not some of the larger solute molecules. Cell membranes are described as selectively permeable because not only do they allow the passage of water but also allow the passage of certain solutes. The presence of particular solutes stimulates the membrane to open specific channels or trigger active transport mechanisms to allow the passage of those chemicals across the membrane. Some major examples of osmosis Absorption of water by plant roots. Re-absorption of water by the proximal and distal convoluted tubules of the nephron. Re-absorption of tissue fluid into the venule ends of the blood capillaries. Absorption of water by the alimentary canal — stomach, small intestine and the colon. Osmoregulation Osmoregulation is keeping the concentration of cell cytoplasm or blood at a suitable concentration. Amoeba, living in freshwater, uses a contractile vacuole to expel the excess water from its cytoplasm (thus need more respiration/O2/ATP than isotonic (marine) Amoebae). The kidneys maintain the blood (thus, whole body) at the correct concentration. Osmosis and Plant Cells a. Plant Cells in a hypotonic (= weaker) solution - cells have lower water potential The plant cells gain water by osmosis. The vacuole and cytoplasm increase in volume. The cell membrane is pushed harder against the cell wall causing it to stretch a little The plant tissue becomes stiffer (=TURGID) b. Plant Cells in a hypertonic (=stronger) solution - cells have higher water potential The plant cells lose water by osmosis. The vacuole and cytoplasm decrease in volume. The cell shrinks away from the cell wall. Shrinkage stops when the cell sap is at the same concentration as the external solution. The plant tissue becomes flaccid, it has shrunk slightly may go on to become plasmolysed. Turgor Turgor is the pressure of the swollen cell contents against the cell wall when the external solution more dilute than the cell sap of the vacuole. Role of Turgor in Plants Mechanical support for soft non-woody tissue, e.g., leaves. Change in shape of guard cells forming the stomatal opening between them. Enlargement of young immature plant cells to mature size. Water Potential This is the tendency of water to move from one place to another. Values are always negative! Water always flows downhill i.e. towards the more negative number. Units are pressure (kPa) Calculations are not set, but this formula may be: Water Potential (y) = Pressure Potential (yp) + Solute Potential (ys) Pressure Potential = the force of the cell wall on the contents, So for animal cells, this is zero, thus, in animals: Water Potential (y) = Solute Potential (ys) Active Transport Active transport is the energy-demanding transfer of a substance across a cell membrane against its concentration gradient, i.e., from lower concentration to higher concentration. Special proteins within the cell membrane act as specific protein ‘carriers’. The energy for active transport comes from ATP generated by respiration (in mitochondria). Major examples of Active Transport Re-absorption of glucose, amino acids and salts by the proximal convoluted tubule of the nephron in the kidney. Sodium/potassium pump in cell membranes (especially Kinds of transport 1) Passive transport Solute moving in favorable direction, from higher concentration to lower concentration, and/or (for charged solutes) in direction that would reduce charge gradient a) Simple diffusion Solute moves freely across lipid bilayer; no membrane protein needed to assist process -lipophilic solutes, passing freely across membrane -- don't need help b) Facilitated diffusion - Specific protein required to "assist" solute to cross lipid bilayer - Solutes pass "down" concentration or charge gradient, but high activation energy to get them across membrane - Polar or charged solutes have to lose H2O molecules solvating them in aqueous phase before they can cross hydrophobic lipid core of membrane. - Protein transporter ("assisting" molecule) in membrane reduces activation energy barrier to get polar solute, e.g., glucose, across - By binding solute and transporting it across membrane, but only in direction dictated by concentration or charge gradient, OR - By providing an opening (a channel) for solute to cross membrane Solute moves only in direction dictated by concentration or charge gradient. Transporters and channels both display substrate specificity. Some transporters and channels working/"open" all the time; others "gated" (opening regulated by some signal that opens or closes) Cell junctions Tight junctions Tight junctions are structures that are present just below the apical surface to seal the intercellular space to prevent fluid exchange with the lumen. They are formed by the fusion of two adjacent plasma membranes at certain points constituted by two rows of particles (one from each cell). Kidney epithelial cells involved in the transport of water and ions contain a lot of tight junctions. Gall bladder has fewer tight junctions. Tight junctions can thus act as barriers to the diffusion of macromolecules and influence the composition and physiological characteristics of the cell membrane. Belt and Spot Desmosomes Desmosomes are structures that help in mechanical adhesion between cells. There are two types of desmosomes called Belt and Spot desmosomes. Belt desmosomes/terminal bars/intermediary junctions are present just below the tight junctions usually between the columnar cells. They contain actin microfilaments and a group of intermediate filaments. Spot desmosomes are dark bodies on the cell surface. They contain a non-contractile type of filaments called tonofilaments. Tissues experiencing mechanical stress tend to have more spot desmosomes since the tonofilaments can provide intra cellular mechanical support. Some epithelial cells contain fine structures similar to desmosomes but represent only half of them and hence are called hemidesmosomes Gap Junctions In multicellular organisms, cells interact with each other and thereby exert many coordinated functions. Many animal cells show regions of low resistance and are electrically coupled. This type of coupling called junctional communication is often noticed in embryonic cells, epithelial cells, cardiac cells and liver cells but not much in skeletal and neuronal cells. Gap junctions are plaque-like contacts between two cells in which the plasma membranes of adjacent cells are separated only by a space of 2-4 nm. Gap junctions resist proteolysis, mechanical disruption and calcium removal. The gap junctions of the adjacent two cells align with each other and the unit structure is called connexon. Connexon is like a cylinder connecting the membranes of two neighboring cells thus favoring intercellular communication. Connexons are made up of six polypeptides each having a mol. wt. of 26,000. Closing of the channel is achieved by sliding of these hydrophobic subunits against each other. The gap junctions of the adjacent two cells align with each other and the unit structure is called connexon. Connexon is like a cylinder connecting the membranes of two neighboring cells thus favoring intercellular communication. Connexons are made up of six polypeptides each having a mol. wt. of 26,000. Closing of the channel is achieved by sliding of these hydrophobic subunits against each other. Gap junctions can thus regulate the transport of small molecules between the cells. Calcium regulates the channels present in gap junctions. When the intracellular Ca++ concentration is low (10-7M) permeability is high but if these ions are in high concentration, then the permeability is lowered. Gap junction communication is linked with the energy provided by ATP and the membrane potential. Gap junctions are also important for the passage of sugars, nucleotides, vitamins and other metabolites. CELL ORGANELLES Introduction Early electron micrographs of cells in 1945 showed certain tubules that did not reach the periphery and were named as endoplasmic reticulum. Endoplasmic reticulum (ER) is the major component of cytoplasmic vacuolar system also called endomembrane system that includes the nuclear envelope, ER and the Golgi complex. ER with ribosomes is called rough/granular ER. ER without ribosomes is called smooth/agranular ER Similar to the plasma membranes, ER also has a membrane made up of lipid bilayers of 5-6 nm in thickness with peripheral and integral proteins. The size of ER differs with the differentiation status of cells In undifferentiated cells such as eggs or embryonic cells ER is small. Rough Endoplasmic Reticulum (RER) RER is well developed in cells involved in protein synthesis on a large scale. Like plasma membrane ER also has an inner and outer membrane and a space in between. The outer surface is attached with ribosomes present as polysomes in attachment with mRNA. 60S subunit of ribosomes is attached with RER but can freely exchange the subunit present in the cytosol as well during protein synthesis. RER contains ribophorins I (mol. Wt. 65,000) and II (mol. Wt. 64,000) that are not present in the smooth endoplasmic reticulum (SER) Ribophorins interact with each other and mediate the attachment of ribosomes to the RER Smooth Endoplasmic Reticulum (SER) SER lacks ribosome attachment and is present in regions rich in glycogen appearing as dense particles The glycogen particles or glycosomes are about 50-200 nm in size and are made up of smaller particles constituting an overall spheroidal structure. ER formation and its functions ER is thought to be formed be evagination from the nuclear envelope During cell division at telophase the nuclear envelope is formed again by vesicles of ER A multi-step mechanism is involved in the formation of ER After the membrane of lipids and proteins is formed, other components such as enzymes and sugars are added resembling the process of differentiation In the ER formation there is a notable and rapid distribution of phospholipids between the monolayers and proteins are inserted independent of lipids ER functions like a circulatory system carrying various substances such as RNA and nucleoproteins ER also provides a mechanical support for the colloidal structure of the cytoplasm Carriers and permeases help in the transport of substances across the endomembrane Striated muscle fibers contain a special form of ER called sarcoplasmic reticulum that acts as an intracellular conducting system Detoxification of many endogenous and exogenous compounds is an important function of SER SER is also involved in glucogenolysis through the action of glucose-6- phosphatase The synthesis of proteins is one of the major functions of RER and the synthesis of lipids and lipoproteins is an important function for both RER and SER. Golgi Complex Golgi complex or Golgi apparatus receives the proteins from ER and processes them further and then helps in sorting them to be transported to their destinations such as endosomes, lysosomes or secretion Golgi also serves as a place for the synthesis of glycolipids and sphingomyelin In plants the complex polysaccharides of cell wall are synthesized in Golgi Golgi is essentially made up of flat membrane-enclosed sacs called cisternae/dictyosome units and associated vesicles Proteins from ER enter into its convex face called cis face or entry face oriented towards the nucleus Proteins exit through its concave trans/exit face There are three regions in Golgi that include cis Golgi network, Golgi stack (medial and trans) and trans Golgi network Proteins from ER first enter an intermediate compartment and then into the cis Golgi network Lysosomes The organelles involved in intracellular and extracellular digestion are called lysosomes Lysosomes typically contain many digestive enzymes More than 50 hydrolases have been identified in lysosomes capable of digesting a variety of biological substances Lysosomes are present in protozoa, plants and animals but not in bacteria in which the periplasmatic space between the cell wall and plasma membrane plays a similar role Lysosomal enzymes are enclosed by a membrane and thus are not immediately available to their substrates and since the digestion takes place within lysosomes, other parts of the cell are protected from being digested by these enzymes Lysosomal enzymes include lysozyme, N-acetyl-beta-glucosaminidase, aryl sulfatase, acid phosphatase, phosphodiesterase, cathepsins, collagenase, elastase and carboxy peptidases Intralysosomal pH is about 5 and acidification depends on a proton pump present in the lysosomal membrane that acts in an ATP-dependent manner and + accumulates H ions inside the lysosomes. Endocytosis Endocytosis is a process by which solid or fluid materials in bulk are taken up by the cells and thus this is a general term Bulk ingestion of solid materials by the cell is called phagocytosis Bulk ingestion of fluid materials by the cell is called pinocytosis Phagocytosis is a defense mechanism against bacteria, dust and various colloids encountered by the cell Particles or bacteria generally first adhere to the cell and then penetrate and finally destroyed by the process of phagocytosis It is well developed in macrophages and endothelial cells Pinocytosis is induced by proteins, amino acids and certain ions in ameba In cells addition of ATP has been shown to increase the rate of endocytosis and can be inhibited by inhibitors of respiration Increase in oxygen consumption, glucose uptake and glycogen break down occurs in leukocytes during phagocytosis Contraction of microfilaments of actin and myosin facilitates the invagination of the plasma membrane to form the endocytic vacuoles Cytochalasin B, known to disrupt microfilaments also interferes with endocytosis Endocytic vacuoles move towards the Golgi apparatus where the primary lysosomes attach with them and fuse to liberate their contents forming the secondary lysosomes. Autophagy and Phagocytosis NUCLEUS STRUICTURE Nuclear envelope The nuclear envelope structure is better visualized through electron microscope. It has two membranes separated by perinuclear space of about 10-15 nm in width. The membranes have lipid bilayer structure like any other membrane including the plasma membrane. The outer surface contains ribosomes and the nuclear membrane directly continues as ER membrane especially the one with ribosomes. Nuclear envelope is interrupted with many nuclear pores. A fibrous lamina of proteins (50-80 nm thick) attach with the inner membrane but not with the pores. The NPC with 100-nm diameter is a hollow cylinder embedded in the nuclear envelope. It has about 30 different proteins termed nucleoporins (Nups). The NPC acts as the gate between the nucleus and the cytoplasm. Macromolecules carrying specific import and export signals are only allowed to pass through water and metabolites can freely pass through. The central channel is filled and surrounded with FG Nups having many large domains rich in phenylalanine and glycine repeats. The nuclear basket has eight filaments that reach into the nucleoplasm, attached to each other by a ring at the end. Electron microscopy tomographs have shown that filaments extend from this basket into the nucleus The cytoplasmic filaments form highly mobile molecular rods projecting into the cytoplasm. NLS and NES The transport of molecules through the NPC is restricted by size; below a mass of approximately 60 kDa, macromolecules can passively diffuse across the NPC. Such small macromolecules below this cut-off also often contain a nuclear localization signal. Nuclear localization sequences (NLSs) for import into the nucleus and nuclear export sequences (NESs) for export are required. These signals are recognized by transport factors, each with specific signal preferences. Cargoes NPC can be considered an enzyme for transport, in which only the correct substrates (such as transport factors and their cargoes) can bind to the active site and so pass across the nuclear envelope. In living cells, this high transport rate is represented by several transport factors carrying many importing and exporting cargoes, including ribonucleoproteins (RNPs). Import cargoes are mostly proteins that have been synthesized in the cytoplasm and are needed in the nucleus. There are also proteins that, once they reach the nucleoplasm, are exported out again by karyopherins such as XPO1 (also known as CRM1). Export cargoes are usually RNAs, as complexes made of RNA and proteins. The ribosomal subunits and messenger RNPs (mRNPs) are the most abundant of these export cargoes. Protein cargoes (60kDa) are smaller than mRNP cargoes (100 MDa) Nucleolus The nucleolus is involved in the synthesis and processing of ribosomal RNAs (rRNAs) and their assembly into ribosomal subunits It is the largest nuclear domain. Nucleoli show lightly staining fibrillar centres (FCs) of about 0.1-1.0 ^m in size, surrounded by dense fibrillar component (DFC), which is usually more heavily stained than the rest of the nucleolus. Fibrillar centres are the interphase equivalents of the nucleolus organizer regions. The other part of the nucleolus is filled with granules (called the granular component— GC).The granules probably represent pre-ribosomal particles in various stages of maturation. These filaments help NPCs to reach about 100 nm into the nucleus and cytoplasm. Nucleolus structure is evolved The complex structural organization of the nucleolus differs between anamniotes to amniotes. Anamniotes are vertebrates not having an amnion; they lay eggs in water. Amniotes are the organisms (reptiles, birds, etc) laying eggs in the environment. In this transition the Rdna intergenic region increases. Amnion is a protective membrane which covers the embryo in mammals, birds and reptiles. It contains the amniotic fluid. This fluid provides the embryo with the required nutrients. Nucleolus organizer region (NOR) Nucleolus is formed in the Nucleolus Organizer Region. It is surrounded by filaments called the pars fibrosa (PF). The filaments are formed of ribosomal RNA that is newly transcribed. After the division of nucleus, this region gets associated with the nucleus. Several copies of genes of ribosomal RNA are present in this region. Many tandem copies of the genes of these ribosomal RNAs are found in the NOR. Karyotype analysis by means of silver nitrate staining can be used to identify NOR. About 10 NORs found in a cell. Functions of nucleolus The major function of nucleolus is the production and assembling of ribosomal subunits. 50% of the total production of RNA in cells occurs in the nucleolus. Nucleolus is the site for the transcription of rRNA precursor molecule from DNA. These RNAs are packaged with certain specific forms of proteins to produce ribosomal units of varying size. The ribosomal units are then transported into the cytoplasm for translation and protein synthesis. Non-ribosomal functions of nucleolus Nucleoli have nonstandard functions apart from being ribosome factories Nucleolus is also involved in mRNA export or degradation, Biosynthesis of the signal recognition particle (SRP), Biogenesis of some snRNAs and tRNAs, The sequestration of regulatory proteins, Control of the cell cycle, Aging, and stress sensing.