Geology for Engineers Reviewer (Finals) PDF
Document Details
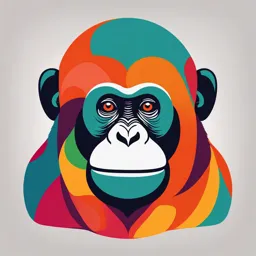
Uploaded by EasierDerivative2114
Tags
Summary
This document is a review of petrology and rocks, covering their definitions, importance, and applications. It details the rock cycle, mineral testing methods, and various types of rocks.
Full Transcript
Geology for Engineers Reviewer (Finals) Petrology and Rocks Introduction to Petrology Definition: o The study of the compositions, structures, and origins of rocks. o Derived from Ancient Greek: Petros (rock) and logos (study). o...
Geology for Engineers Reviewer (Finals) Petrology and Rocks Introduction to Petrology Definition: o The study of the compositions, structures, and origins of rocks. o Derived from Ancient Greek: Petros (rock) and logos (study). o Focuses on the conditions under which rocks form. Importance of Petrology: o Earth History: Offers insights into Earth's geological past. o Mineral Resources: Guides the discovery and development of minerals and ores. o Industrial Applications: ▪ Metallurgy and crystallography began with studying rocks and minerals. ▪ Crystallography is critical for developing microchips and electronics. Subcategories: 1. Igneous Petrology 2. Metamorphic Petrology 3. Sedimentary Petrology Rock Cycle Definition: o A continuous natural process forming, breaking down, and reforming rocks. o Converts between igneous, metamorphic, and sedimentary forms. Processes in the Cycle: 1. Magma Formation: The primary source of all rocks. 2. Cooling and Solidification: Forms igneous rocks. ▪ Intrusive: Cools below the surface. ▪ Extrusive: Cools above the surface as lava. 3. Weathering and Erosion: Breaks down rocks into sediments. 4. Heat and Pressure: Transforms rocks into metamorphic forms. 5. Melting: Converts rocks back into magma. Significance: o Explains Earth's material recycling. o Helps geologists understand Earth’s history and natural resources. Processes and Tests for Minerals A. Mining Methods 1. Open-Pit Mining: Large pits excavated to extract minerals. 2. Underground Mining: Tunnels are dug to access deposits. 3. Solution Mining: Chemicals dissolve and extract minerals. B. Equipment for Mineral Testing 1. Streak Plate: Tests the streak of minerals. 2. Mohs Hardness Test Kit: Measures hardness on a scale from 1 to 10. 3. Hand Lens (Loupe): Magnifies minerals for closer examination. 4. Microscope: Examines internal structures and textures. 5. Hydrochloric Acid (HCl): Performs the "acid test" for carbonate minerals. 6. Hydrostatic Balance: Measures specific gravity. 7. Magnet: Identifies magnetic properties. 8. Fluorescence Lamp: Detects fluorescence under UV light. 9. Geologist’s Hammer: Breaks and splits rocks. 10. Refractometer: Measures the refractive index of minerals. C. Mineral Processing Steps 1. Crushing and Grinding: Reduces ore size to liberate minerals. 2. Concentration: Separates valuable minerals from waste: o Gravity Separation o Flotation o Magnetic Separation 3. Leaching: Uses chemicals to extract minerals like gold and copper. 4. Purification: Refines minerals using techniques like smelting or electrolysis. D. Testing Methods 1. Chemical Analysis: Identifies elemental composition. 2. Physical Properties Testing: Assesses hardness, density, luster, etc. 3. Mechanical Testing: Evaluates strength and durability. 4. Microscopic Analysis: Examines grain size, inclusions, and textures. 5. Petrographic Analysis: Studies mineral origin and history using polarized light microscopy. Different Types of Rocks I. Igneous Rocks Formation: From melted rock (magma/lava) that cools and solidifies. Types: o Extrusive Rocks (Volcanic): Formed at the surface (e.g., basalt). o Intrusive Rocks (Plutonic): Formed beneath the surface (e.g., granite). Formation Process 1. Crystallization: Minerals solidify at different temperatures during cooling. o Can result in partially crystallized magma. Characteristics Composition: Silicate minerals (Oxygen, Silicon, Aluminum, etc.). Crystals: Angular shapes, visible in intrusive rocks. Examples Of Igneous Rocks A. Granite Occurrence: Mountain roots, batholiths, dikes, and sills. Physical Properties: o Hardness: 6-7 on Mohs scale. o Color: White, black, gray, pink, red. o Texture: Coarse-grained, granular. o Density: 2.65 g/cm³. o Porosity: Low (resistant to water/weathering). Uses: Building facades, countertops, flooring, monuments. B. Syenite Occurrence: Plutons, mountain cores, alkaline rock complexes, and sills/dikes. Physical Properties: o Hardness: 5-6 on Mohs scale. o Color: Gray, pink, green. o Texture: Coarse-grained, granular. o Density: 2.6–2.7 g/cm³. o Porosity: Low. Uses: Construction, ornamental stone, aggregate. C. Diorite Occurrence: Subduction zones, volcanic arcs, orogenic belts. Physical Properties: o Hardness: 5-6 on Mohs scale. o Color: Dark gray to black with speckles. o Texture: Coarse-grained, interlocking crystals. o Density: 2.7–3.0 g/cm³. o Porosity: Low. Uses: Road base material, facades. D. Gabbro Occurrence: Oceanic crust, mountain belts, island arcs. Physical Properties: o Hardness: 6-7 on Mohs scale. o Color: Black or dark green. o Texture: Coarse-grained. o Density: 2.9–3.3 g/cm³. o Porosity: Low. Uses: Crushed stone for roads, countertops, flooring. E. Pegmatite Occurrence: Late-stage magma crystallization, fractures along batholiths. Physical Properties: o Hardness: 6-7 on Mohs scale. o Color: Light pink, white, gray. o Texture: Coarse-grained, very large crystals. o Density: 2.6–2.7 g/cm³. o Porosity: Low. Uses: Decorative stone, construction aggregate. F. Dolerite (diabase) Occurrence: Intrusive formations like dikes and sills. Physical Properties: o Hardness: 6-7 on Mohs scale. o Color: Black or dark gray. o Texture: Fine- to medium-grained. o Density: 2.9–3.0 g/cm³. o Porosity: Low. Uses: Road construction, dimension stone. G. Basalt Occurrence: Ocean floors, volcanic regions. Physical Properties: o Hardness: 6 on Mohs scale. o Color: Black or dark gray. o Texture: Fine-grained, compact. o Density: 2.8–3.0 g/cm³. o Porosity: Low. Uses: Road base material, paving stones, railway ballast. II. Sedimentary Rock Definition of Sedimentary Rock Formed by compaction or cementation of sediments. Sediments originate from weathered rocks or dissolved materials. Comprised of sand, silt, plant/animal remains. Key in studying Earth’s history due to layers (strata) and fossils. Types of Sedimentary Rocks 1. Detrital Sedimentary Rocks o Formed from particles of weathered rocks (detritus). o Example: Shale, Sandstone. 2. Chemical Sedimentary Rocks o Derived from dissolved materials solidifying (e.g., salt deposits). o Example: Limestone. Formation Process 1. Weathering o Breakdown of rocks by natural forces. 2. Erosion o Transport by water, wind, waves, or glaciers. 3. Deposition o Sediments settle in rivers, lakes, seas. 4. Lithification o Compaction and cementation of layers over time. Key Features Strata/Bed Layers: Accumulated sedimentary layers. Fossils: Evidence of past life, aiding in Earth’s historical studies. Examples of Sedimentary Rocks 1. Sandstone o Composed of sand-sized grains. o Found in deserts, riverbeds, beaches. o Uses: Building stone, paving, cladding. 2. Limestone o Primarily calcium carbonate (CaCO₃). o Formed from shells/coral in marine settings. o Uses: Cement, building materials, aggregate. 3. Shale o Fine-grained with fissility (splits into thin layers). o Formed from silt and clay particles. o Uses: Bricks, cement, construction fill. 4. Conglomerate o Made of rounded gravel and boulders. o Found in riverbeds, alluvial fans, and coasts. o Uses: Concrete aggregate, decorative stone. 5. Breccia o Contains angular clasts cemented together. o Found in landslides, volcanic areas, fault zones. o Uses: Decorative building stone, aggregate. Physical and Chemical Properties 1. Sandstone o Hardness (Mohs): 6-7 o Texture: Medium to coarse-grained o Porosity: Moderate o Reactivity: Inert (mainly quartz), carbonate content reacts with acids o Density: 2.2–2.8 g/cm³ 2. Limestone o Hardness (Mohs): 3-4 o Texture: Fine to medium-grained o Porosity: Moderate to high o Reactivity: Reacts readily with acids due to calcium carbonate composition o Density: 2.3–2.7 g/cm³ 3. Shale o Hardness (Mohs): 2-3 o Texture: Fine-grained and fissile (splits into thin layers) o Porosity: Low o Reactivity: Generally inert unless carbonates are present o Density: 2.4–2.8 g/cm³ 4. Conglomerate o Hardness (Mohs): 6-7 o Texture: Coarse-grained with rounded clasts o Porosity: Low to moderate o Reactivity: Generally inert or silica-based, carbonate components react with acids o Density: 2.5–2.8 g/cm³ 5. Breccia o Hardness (Mohs): 6-7 o Texture: Coarse-grained with angular clasts o Porosity: Low to moderate o Reactivity: Similar to conglomerate; mainly inert, carbonate components react with acids o Density: 2.5–2.8 g/cm³ Uses of Sedimentary Rocks 1. Construction: o Sandstone and limestone in building materials. o Shale for cement, bricks, and tiles. o Breccia and conglomerate as decorative stones. 2. Industrial Applications: o Limestone in cement and aggregate. o Sandstone for paving and flooring. 3. Landscaping and Aesthetics: o Breccia and conglomerate for decorative elements. III. Metamorphic Rock Definition of Metamorphism Metamorphism means "transformation or change in form". It alters the texture, mineralogy, and chemical composition of rocks due to temperature, pressure, and other factors. Sedimentary, igneous, or even other metamorphic rocks can undergo metamorphism as part of the rock cycle. Process of Metamorphic Rock Formation 1. Heat and Pressure: Sedimentary rocks are buried and subjected to intense heat and pressure. 2. Mineral Transformation: Existing minerals break down, and new minerals form. 3. Magma Formation: When exposed to extreme heat and pressure, rocks may melt, forming magma, and restarting the cycle. Characteristics of Metamorphic Rocks Textures: o Foliated: Appears layered or banded due to aligned minerals (e.g., slate, schist, gneiss). o Non-foliated: Uniform in texture without layers (e.g., quartzite, marble). Parent Rocks: Each metamorphic rock has a parent rock (e.g., sandstone → quartzite, limestone → marble). Examples of Metamorphic Rocks 1. Quartzite Origin: Derived from sandstone. Texture: Granular, non-foliated, interlocking quartz grains. Occurrence: Found in mountain-building zones at convergent plate boundaries. Physical Properties: o Hardness: 7 (Mohs scale) o Density: 2.6–2.7 g/cm³ o Color: White, gray, pink, or red. o Porosity: Very low; resistant to water. Uses: o Building stone (exteriors, skyscrapers) o Flooring and roofing o Aggregates for road construction 2. Marble Origin: Derived from limestone under heat and pressure. Texture: Fine to coarse-grained, crystalline. Occurrence: Common at convergent tectonic plate boundaries. Physical Properties: o Hardness: 3-4 (Mohs scale) o Density: 2.7–2.9 g/cm³ o Color: Typically white, but varies with impurities (red, gray, green). o Porosity: Low to moderate. Uses: o Building interiors (luxury floors, columns) o Countertops and tiles o Monuments and sculptures 3. Slate Origin: Formed from shale or mudstone under low-grade metamorphism. Texture: Fine-grained, foliated, with slaty cleavage. Occurrence: Found in sedimentary basins undergoing low-grade metamorphism. Physical Properties: o Hardness: 3-5 (Mohs scale) o Density: 2.7–2.9 g/cm³ o Color: Gray, black, green, purple, or red. o Porosity: Low; highly water-resistant. Uses: o Roofing tiles o Flooring and wall tiles o Paving stones 4. Gneiss Origin: High-grade metamorphism of various rocks. Texture: Coarse-grained, foliated, with alternating light and dark mineral bands. Occurrence: Found at convergent plate boundaries. Physical Properties: o Hardness: 6-7 (Mohs scale) o Density: 2.6–2.9 g/cm³ o Color: Striped or banded appearance. o Porosity: Low. Uses: o Construction aggregate o Decorative stone for facades, countertops 5. Schist Origin: Progresses from slate to phyllite, then schist, due to regional metamorphism. Texture: Foliated, with visible platy minerals like mica. Occurrence: Found in regions of intense tectonic activity. Physical Properties: o Hardness: 4-5 (Mohs scale) o Density: 2.7–2.9 g/cm³ o Color: Green, gray, or silver, depending on minerals. o Porosity: Low. Uses: o Building stone for walls and foundations o Decorative stone for landscaping Importance of Petrology and Rocks in Civil Engineering Definition Of Petrology Petrology is the study of rocks' texture, structure, mineral composition, and chemical composition. Significance Of Petrology In Civil Engineering Logical Basis: Provides insights into rocks' physical properties like strength and durability. Suitability Assessment: Helps civil engineers assess rocks for specific construction purposes. Diversity of Rocks: Different types of rocks are suitable for various applications— no single rock is ideal for all uses. Examples Of Rock Applications Granite: Its hardness, durability, and absence of weak planes make it suitable for foundations. Limestone (e.g., Cuddapah and Shahabad types): Due to its flat surfaces and easy workability, it is ideal for flooring and roofing. Marble: With its attractive colors and softness, it is often used for face work, statues, and decorative purposes. Sandstone: Its easy workability allows it to be neatly dressed, making it suitable for wall construction. Importance Of Proper Rock Selection Maximizing Utility: Knowing inherent characteristics ensures optimal use of available rocks. Project Success: Correct rock choice contributes to the strength, durability, and aesthetic quality of construction. Structural Geology and Rock Mechanics Attitude of Beds Definition: Refers to the orientation of geological features (e.g., rock layers, faults, folds) within the Earth's crust. Importance: Describes how rock layers are positioned in space, critical for geological and structural analysis. Types of Measurements in Geological Features Strike (Trend): o Direction of the line formed by the intersection of a rock layer or fault with a horizontal plane. Dip (Inclination): o Angle of inclination of a rock layer or fault relative to a horizontal plane. Orientation of Structures Planar Features: o Flat surfaces or features approximated as lying on a plane. Linear Features: o Long, narrow shapes extending in a straight line or along a linear path. Outcrops Definition: Exposed bedrock at the Earth’s surface. Kinds of Outcrops: 1. Igneous Outcrops 2. Sedimentary Outcrops 3. Metamorphic Outcrops Formation of Outcrops: 1. Weathering: Decomposition of rocks, soil, and minerals. 2. Erosion: Displacement of solids by wind, water, or ice. 3. Human Activity: Quarrying, mining, road construction, and excavation. Outcrop Patterns 1. Flat-lying Beds: o Intersections align closely with contour lines, running parallel to them. 2. The Rule of V’s: o Dipping surfaces incised by stream valleys or canyons create V-shaped patterns on maps. 3. Plunging Folds: o Zigzag patterns that change direction at fold hinges. Geologic Maps Definition: Uses lines, symbols, and colors to represent the distribution and nature of rock units in an area. Purpose: o Shows rock types and surficial deposits. o Displays locations of geologic structures (e.g., faults, folds). o Identifies potential natural hazard areas. o Highlights energy resources. Components of Geologic Maps 1. The Map Itself 2. The Map Legend 3. Geologic Cross-section(s) of the Area Types of Geologic Maps 1. Bedrock Maps: Show the distribution of rock types. 2. Surficial Maps: Display surface materials and deposits. 3. Structural Maps: Illustrate the distribution of geological structures. 4. Mineral Maps: Identify locations of mineral deposits. 5. Geologic Hazard Maps: Highlight areas prone to hazards like landslides and seismic activity. Importance of Geologic Maps Geological Understanding: Helps geologists develop a theoretical understanding of geology and geologic history. Practical Applications: Essential for zoning, engineering, hazard assessment, and resource development (e.g., sediments, groundwater, fossil fuels, and minerals). Importance In Engineering and Construction Hazard Assessment: Identifies geologic hazards (e.g., landslides, sinkholes, seismic activity) for designing safe and effective infrastructure. Study of Structures I. Folds Definition: Result from the bending of originally planar sedimentary strata during deformation. Types of Folds: 1. Anticlines: Upward-arching folds. 2. Synclines: Downward-arching folds. 3. Monoclines: A single, large step in the rock layer. 4. Chevron: Sharp, angular folds. 5. Recumbent: Almost horizontal folds. 6. Isoclinal: Parallel layers in a fold. 7. Plunging: Folds with tilted axes. 8. Dome: Circular, upward bulge. 9. Basin: Circular, downward bulge. 10. Ptygmatic: Highly folded, irregularly shaped folds. 11. Slump: Folds formed by gravitational sliding. II. Faults Definition: Fractures or zones where rock blocks move relative to each other. Types of Faults: 1. Normal Fault: One block moves down relative to the other. 2. Strike-slip Fault: Blocks move horizontally. 3. Reverse (Thrust) Fault: One block moves up relative to the other. 4. Oblique-slip Fault: Combination of vertical and horizontal movement. III. Joints Definition: Fractures in rock bodies resulting from tensile and compressive stresses. Formation of Joints: 1. Contraction during rock formation. 2. Expansion and contraction due to temperature and pressure changes. 3. Crustal disturbance from tectonic forces. Types of Joints According to Classification: o Spatial Relationship: 1. Nonsystematic Joint: Randomly oriented. 2. Systematic Joint: Organized and patterned. o Geometry: 1. Strike Joint: Runs parallel to the strike. 2. Dip Joint: Runs parallel to the dip. 3. Oblique Joint: Diagonal orientation. o Genesis: 1. Tension Joint: Formed by tensile stress. 2. Shear Joint: Formed by shear stress. 3. Compression Joint: Formed by compressive stress. Physical Properties of a Rock Mineral Composition, Structure, and Texture: Essential in determining the overall characteristics and classification of rocks. Density, Void Ratio, and Porosity: Influence the rock’s ability to store and transmit fluids and gases. Permeability: Determines how easily fluids can pass through the rock. Electrical and Thermal Properties: Important for assessing rock behavior under different environmental and engineering conditions. Specific Gravity and Unit Weight: Used in calculations for construction and geological assessments. Moisture Content and Degree of Saturation: Affect the rock's physical and mechanical behavior. Swelling, Anisotropy, and Durability: Critical for understanding how rocks respond to environmental changes and stresses. Mechanical Properties of Rocks Strength: Refers to the ability of the rock to withstand stress without breaking. Deformability: The capacity of the rock to change shape under stress. Hardness: Indicates the resistance of the rock to indentation and abrasion. Elasticity: The ability of the rock to return to its original shape after deformation. Plasticity: The ability to deform permanently without breaking. Dynamic Properties of Rocks Modulus, Damping, and Wave Velocity: Important for geotechnical engineering, influencing seismic design, foundation analysis, and slope stability. Applications Modulus of Deformation – Young’s Modulus: Reflects the stiffness of an earth material and its resistance to elastic deformation under load. Modulus of Rigidity – Shear Modulus: Describes the elastic response when a shear force is applied, resulting in lateral deformation. Bulk Modulus: Measures a substance's ability to withstand changes in volume under uniform compression. Importance of Structural Geology and Rock Mechanics Incorporating structural geology and rock mechanics into civil engineering enhances the safety, efficiency, and sustainability of construction projects. This integration leads to better infrastructure and reduced risks for the community by ensuring that construction practices account for geological conditions and rock behavior. Dynamic Properties of Rocks Dynamic properties describe how rocks behave under changing conditions, such as those caused by seismic waves or explosions. 1. Modulus of Deformation (Young’s Modulus) o Reflects the stiffness of a material or resistance to elastic deformation under load. o Named after British scientist Thomas Young, who first described it in 1807. o Represents the elastic properties of a solid under tension or compression in one direction. 𝜎 o Formula: 𝐸 = 𝜖 where: ▪ 𝐸 = Young's Modulus (Modulus of Elasticity) ▪ 𝜎 = Stress ▪ 𝜖 = Strain o Higher Young's Modulus means a stiffer material. o Evaluation: Performed using a Tensile Testing Machine to measure tensile strength. 2. Modulus of Rigidity (Shear Modulus) o Measures the material's response to shear stress, resulting in lateral deformation. o Indicates how a material deforms under forces pushing in opposite directions. 𝜏 o Formula: 𝐺 = 𝛾 where: ▪ 𝐺 = Shear Modulus (Modulus of Rigidity) ▪ 𝜏 = Shear Stress ▪ 𝛾 = Shear Strain o Higher shear modulus means more rigidity. 3. Bulk Modulus o Measures the ability of a substance to withstand changes in volume under uniform compression. 𝑉 o Formula: 𝐾 = −𝜌( ) ∆𝑉 where: ▪ 𝐾 = Bulk Modulus ▪ 𝜌 = Pressure ▪ 𝑉 = Initial Volume ▪ ∆𝑉 = Change in Volume o A substance that is difficult to compress has a high bulk modulus and low compressibility. 4. Poisson’s Ratio o Defined as the ratio of lateral strain to axial strain for uniaxial stress. o Named after physicist Siméon Poisson. o Indicates the lateral expansion of a material under compression. 𝜖𝑙𝑎𝑡𝑒𝑟𝑎𝑙 o Formula: 𝑣 = 𝜖 𝑎𝑥𝑖𝑎𝑙 where: ▪ 𝑣 = Poisson’s Ratio ▪ 𝜖𝑙𝑎𝑡𝑒𝑟𝑎𝑙 = Lateral Strain ▪ 𝜖𝑎𝑥𝑖𝑎𝑙 l = Axial Strain o Values typically range between 0.0 and 0.5. High Poisson’s ratio implies high stiffness. Waves and Wave Properties Definition of a Wave: A wave is a disturbance that transmits energy from one place to another, without the transfer of matter. Properties of a Wave: Crest: Maximum point of displacement. Trough: Lowest point in a cycle. Amplitude: Distance from the resting position to the maximum displacement. Wavelength: Distance between two consecutive points like crest to crest. Types of Waves: 1. Longitudinal Waves: Particle movement is parallel to the direction of wave propagation (e.g., P-waves). 2. Transverse Waves: Particle movement is perpendicular to the direction of wave propagation (e.g., S-waves). 3. Seismic Waves: Generated by earthquakes or explosions, traveling within or along the earth's surface. o Body Waves: ▪ P-waves (Primary): Fastest, move through solids and liquids. ▪ S-waves (Secondary): Slower than P-waves, move only through solids. o Surface Waves: ▪ Love waves: Fastest surface waves, move side to side. ▪ Rayleigh waves: Roll along the ground, moving up and down. Wave Velocity: Formula: 𝑣 = 𝑓𝜆 where: o 𝑣 = velocity o 𝑓 = frequency o 𝜆 = wavelength Factors Influencing Wave Velocity: Wavelength: Longer wavelengths result in higher velocity. Frequency: Higher frequency increases wave velocity. Medium: o Density: Higher density slows wave velocity. o Elasticity: More elastic means faster wave velocity. Temperature: Warmer conditions lead to faster wave velocity. Elasticity and Hooke’s Law Elasticity: The ability of a material to return to its original shape after being stretched or compressed. Elastic Deformation vs. Plastic Deformation: Elastic Deformation: Temporary and fully recoverable when the force is removed. Plastic Deformation: Permanent change when the material is stretched beyond its elastic limit. Hooke’s Law: States that within the elastic limit, stress is directly proportional to strain. Formula: 𝜎 = 𝐸𝜖 where: o 𝜎 = Stress o 𝐸 = Elastic Modulus o 𝜖 = Strain Moduli of Elasticity 1. Static Modulus of Elasticity: Measures material stiffness under static load conditions. Important for structural deflection calculations. 𝜎 Formula: 𝐸 = 𝜖 2. Dynamic Modulus of Elasticity: Measures stiffness under rapidly changing or cyclic loads. Critical for evaluating materials under dynamic conditions, such as earthquakes. 𝑝𝑣 2 Formula: 𝐸𝑑 = 1−𝑣2 Difference Between Static and Dynamic Moduli: Static Moduli: Influenced by temperature and microstructure; typically, lower. Dynamic Moduli: Higher, as they measure resistance under rapid loading. Grouting Definition: The injection of pumpable materials into soil or rock to modify physical characteristics. Importance of Grouting: Provides strong bonds, prevents cracking, and improves aesthetics. Enhances waterproofing and moisture protection. Seals joints and fills gaps to prevent water infiltration. Uses of Grouting: Tile Installation: Creates a smooth, water-resistant surface. Concrete Repair and Waterproofing: Seals cracks and voids to restore structural integrity. Foundation and Soil Stabilization: Improves load-bearing capacity and prevents settlement. Dam and Underground Construction: Prevents water infiltration and stabilizes structures. Jointing: Creates watertight connections between precast concrete. Grouting Tools and Equipment: 1. Grout Pump 2. Grout Mixer 3. Injection Equipment 4. Drill 5. Electric Trowel Types of Grouts: Cementitious Grouts: Mixture of cement and water; used in repairs. Chemical Grouting: Chemical reactions solidify or expand grout. Resin Grouts: Epoxy or polyurethane; used for sealing. Microfine Cement Grouts: Fills small cracks. Silicate Grouts: Strengthens soils under foundations. Types of Grouting Techniques: 1. Permeation Grouting: Fills soil pores without disturbing structure. 2. Compaction Grouting: Consolidates soil by injecting soil-cement grout. 3. Jet Grouting: Uses high-velocity jets to mix soil with grout. 4. Rock/Fissure Grouting: Fills rock fractures without creating new ones. 5. Compensation Grouting: Forms lenses within soil to stabilize it. Grouting Process: 1. Preparation: Assess, select grout, prepare equipment. 2. Mixing: Prepare the grout mixture. 3. Application: Inject grout, monitor flow. 4. Finishing: Seal surface and clean tools. 5. Curing: Allow curing and inspect. 6. Post-application: Final touches and monitor performance. Advantages of Grouting: Soil stabilization, structural support, water control, and leakage mitigation. Disadvantages of Grouting: Environmental hazards, potential for shrinkage, limited durability, and health risks. Geological and Geophysical Investigation in Civil Engineering Introduction A. Geological Investigation Definition: Evaluates ground conditions of a site by studying rocks, soil, and groundwater for the safety and stability of construction projects. Objectives: o Foundation Design: Identify foundation conditions and soil-bearing capacity. o Site Stability: Assess landslides, erosion, and seismic risks. o Material Identification: Classify soil and rock types for construction and excavation. o Groundwater Conditions: Identify the location and impact of the water table. o Environmental Impact: Evaluate geological factors affecting the environment. Importance: o Risk Mitigation: Prevent structural failures from geohazards. o Cost Control: Reduce design revisions and construction delays. o Sustainability: Ensure long-term project resilience and minimal environmental impact. B. Geophysical Investigation Definition: Non-invasive exploration of subsurface conditions using physical measurements. Objectives: o Subsurface Profiling: Map underground layers and features. o Hazard Identification: Detect cavities, fault lines, and unstable ground. o Non-Invasive Exploration: Assess areas without drilling. o Groundwater Detection: Locate water sources and assess water levels. o Material Properties: Measure density, conductivity, and seismic velocity. Advantages: o Non-Invasive: Cost-effective and quick. o Large Area Coverage: Rapid assessment of expansive regions. o Early Hazard Detection: Identify unseen dangers. Limitations: o Indirect Data: Verification needed with direct methods. o Complex Interpretation: Requires expertise to avoid misinterpretation. o Depth Limitation: Some methods are less effective in certain soils. Site Investigation Procedure: 1. Preliminary Investigation: o Purpose: Gather initial site data. o Tasks: Review maps, surveys, previous reports, and perform an initial site visit. 2. Field Investigation: o Geologic Reconnaissance and Mapping: Identify and document geological features. o Geophysical Measurements: ▪ Seismic Reflection: Measures seismic wave velocity. ▪ Electrical Resistivity: Assesses ground resistance to electrical current. ▪ Ground Penetrating Radar (GPR): Detects subsurface changes and voids. o Drilling and Sampling: ▪ Boreholes: Obtain core samples. ▪ Standard Penetration Test (SPT): Assesses soil strength. ▪ Cone Penetration Test (CPT): Evaluates soil properties. 3. Final Investigation: o Laboratory Testing: Analyze soil, rock, and water samples. o Data Analysis: Integrate field data for detailed models. o Monitoring: Track changes over time for long-term site stability. Geological Methods 1. Surface Explorations: o Topographic Maps: Create maps to represent terrain features. o Aerial Surveys: Use cameras in low-flying planes for photography. o Photogeology: Map vast areas using satellite images. o Instruments/Equipment: ▪ LIDAR: Creates detailed 3D maps. ▪ Large Format Cameras: Provide high-resolution imagery. ▪ Photogrammetry Systems: Reconstruct 3D models. ▪ UAVs: Capture data from remote or hazardous locations. o Hydrogeological Surveys: Record drainage patterns and water levels. o Instruments: ▪ Groundwater Detector: Maps water sources. 2. Subsurface Explorations: o Direct Method: Use drilling, trial pits, tunnels, etc., to examine underground materials. o Indirect Method: Use geophysical techniques for subsurface assessment. 3. Tests in Geologic Methods: o Standard Penetration Test: Collects samples and measures penetration resistance. o Permeability Test: Measures water flow rate through soil. o Vane Shear Test: Estimates undrained shear strength. o Penetrometer Test: Measures resistance to penetration. Exploration Techniques 1. Geophysical Surveys: Steps: 1. Setup: Define geoscience objectives and gather prior information. 2. Properties: Identify physical properties (e.g., density, conductivity). 3.Data: Collect high-quality, cost-effective data. 4.Processing: Create maps or 3D images. 5.Interpretation: Analyze results for geological insights. 6.Synthesis: Correlate findings with prior data and assess their completeness. 2. Geologic Mapping: Purpose: Represent the distribution and types of rock and soil. Steps: 1. Test Drilling: Locate formations and confirm presence. 2. Preparing a Geological Map: Plot rock units, structures, and age relationships. 3. Reporting: Ensure accurate data collection for reliable reports. 3. Geochemical Survey: Purpose: Study geological samples for construction suitability and mineralization. Steps: 1. Research Methods: Choose suitable techniques. 2. Use Multiple Methods: Integrate different techniques for accuracy. 3. Based on Experience: Apply knowledge from past investigations. 4. Conduct Calibration: Ensure reliable results. 5. Integrate Data: Combine findings with geological and geophysical data. 4. Desktop Survey: Purpose: Initial exploration using existing records and reports. Tasks: Review maps, reports, and other relevant documents before fieldwork. Geological and Geophysical Investigation Overview Geological Investigation: Analyzes rock and soil types, properties, and composition to assess stability, potential landslides, subsidence, and groundwater conditions. Determines suitability for construction. Geophysical Investigation: Uses physical principles (e.g., wave velocities, resistivity) to assess subsurface conditions without direct sampling. It complements geological investigations by saving time and money and aiding in identifying contaminated areas and gaps between boreholes. Geophysical Methods Non-Seismic Methods 1. Gravity Survey o Principle: Measures variations in the Earth’s gravitational field. o Applications: Mineral exploration, subsurface mapping, geological structure detection. 2. Magnetic Survey o Principle: Measures variations in the Earth's magnetic field due to magnetic properties of materials. o Applications: Mapping geological structures, mineral exploration, locating buried objects. 3. Electrical Resistivity Survey o Principle: Measures the sub surface’s ability to conduct electrical current. o Applications: Groundwater studies, environmental investigations, archaeological surveys. 4. Ground Penetrating Radar (GPR) o Principle: Uses radar pulses to detect and image subsurface interfaces. o Applications: Environmental and archaeological studies, subsurface imaging. 5. Electromagnetic (EM) Methods o Principle: Measures how the subsurface responds to electromagnetic fields. o Applications: Groundwater studies, mineral exploration, mapping conductive structures. 6. Remote Sensing o Principle: Collects data from satellites or aerial platforms. o Applications: Geological mapping, land cover classification, environmental monitoring. Seismic Methods 1. Seismic Reflection o Principle: Seismic waves are sent into the subsurface and analyzed for reflections. o Applications: Oil and gas exploration, subsurface mapping, engineering studies. 2. Seismic Refraction o Principle: Analyzes seismic waves refracted at subsurface boundaries. o Applications: Engineering, groundwater studies, shallow investigations. 3. Surface-Wave Methods o Principle: Measures characteristics of surface wave propagation. o Applications: Shallow imaging, site characterization, geotechnical studies. 4. Downhole Seismic Methods o Principle: Seismic sensors are deployed in boreholes for detailed subsurface information. o Applications: Reservoir characterization, geological studies, monitoring subsurface changes. 5. Cross hole Seismic Methods o Principle: Seismic sources and receivers are placed in different boreholes. o Applications: Geotechnical investigations, subsurface material characterization. Seismic vs. Electrical Methods Seismic Methods: Measure wave-fields generated by seismic waves, such as those from earthquakes. Electrical Methods: Detect electric current flow, measuring potentials, currents, and electromagnetic fields. Direct Penetration Techniques Test Pits: Dug to assess soil conditions before construction. Average depth: 3-15 ft, max 20 ft. Core Boring: Extracts cores of material for detailed analysis of rock and mineral composition. Auger Boring: Uses helical augers to remove soil, suited for non-rocky terrains. Wash Boring: Uses water to remove soil, quick and cost-effective; best for non- gravel soils. Core Logging: Analyzes core samples to determine mineral composition, aiding in mining project decisions. Importance of Geological Conditions in Construction Essential Factors for Dams, Tunnels, Buildings, and Road Cuttings: 1. Stable Foundation o Ensures even weight distribution, reducing settlement and damage risks. Improves structure durability and safety. 2. Competent Rock o Strong, stable rock (igneous, metamorphic) supports structures effectively. 3. Minimal Faults and Fractures o Reduces the risk of landslides and structural damage. 4. Water Permeability o Dams: Require impermeable materials to prevent leakage. o Tunnels: May benefit from permeable materials for drainage. o Buildings and Road Cuttings: High permeability can lead to erosion and damage; low permeability can affect stability. 5. Seismic Stability o Essential for regions prone to earthquakes. Ensures structures can withstand seismic forces to avoid collapse. 6. Slope Stability o Prevents landslides and erosion, particularly important for steep terrains. o Requires stable slopes and adequate drainage systems. 7. Groundwater Conditions o High groundwater levels can cause erosion and settlement; low levels can affect stability and subsidence. o Proper groundwater control is necessary for construction safety and cost efficiency. 8. Soil Conditions o Bearing Capacity: Ensures soil supports the structure without excessive settling. o Moisture Content & Erosion: Influences stability and potential for structural damage.