Case 8: Kidney Anatomy and Physiology PDF
Document Details
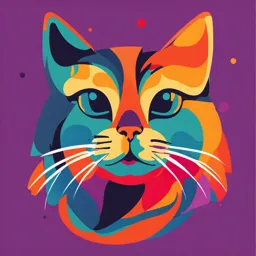
Uploaded by SupportingAutoharp5841
Maastricht University
Tags
Summary
This document provides a detailed description of the anatomy and physiology of the kidneys, including their structure, function, and vascularization. It covers concepts such as the urinary system, nephrons, and filtration processes.
Full Transcript
Case 8 - The kidneys 1. What is the general anatomy of the kidneys? The urinary system is composed of the kidneys and accessory structures (FIG. 19.1a). The study of kidney function is called renal physiology, from the Latin word renes, meaning “kidneys.” The Urinary System Consists of Kidneys, Ur...
Case 8 - The kidneys 1. What is the general anatomy of the kidneys? The urinary system is composed of the kidneys and accessory structures (FIG. 19.1a). The study of kidney function is called renal physiology, from the Latin word renes, meaning “kidneys.” The Urinary System Consists of Kidneys, Ureters, Bladder, and Urethra Urine production begins when water and solutes move from plasma into the hollow tubules (nephrons) that make up the bulk of the paired kidneys. These tubules modify the composition of the fluid as it passes through. The modified fluid, now called urine, leaves the kidney and passes into a smooth muscle tube called a ureter. There are two ureters, one leading from each kidney to the urinary bladder. The bladder expands and fills with urine until, in a reflex called micturition or urination, the bladder contracts and expels urine through a single tube, the urethra. The kidneys are the site of urine formation. They lie on either side of the spine at the level of the 11th and 12th ribs, just above the waist. Although they are below the diaphragm, they are technically outside the abdominal cavity, sandwiched between the membranous peritoneum, which lines the abdomen, and the bones and muscles of the back. Because of their location behind the peritoneal cavity, the kidneys are sometimes described as being retroperitoneal. The concave surface of each kidney faces the spine. The renal blood vessels, nerves, lymphatics, and ureters all emerge from this surface. Renal arteries, which branch off the abdominal aorta, supply blood to the kidneys. Renal veins carry blood from the kidneys to the inferior vena cava. - At any given time, the kidneys receive 20–25% of the cardiac output, even though they constitute only 0.4% of total body weight (4.5–6 ounces each). This high rate of blood flow through the kidneys is critical to renal function. Kidney capsule (renal capsule) The renal capsule consists of three layers of connective tissue or fat that cover your kidneys. It protects your kidneys from injury, increases their stability and connects your kidneys to surrounding tissues. Renal artery The renal artery is a large blood vessel that controls blood flow into your kidneys. For most people at rest, the renal kidneys pump a little over 5 cups (1.2 liters) of blood to your kidneys each minute. Renal cortex The outer layer of your kidney, where the nephrons (blood-filtering units) begin. Contains the renal corpuscles and parts of the nephron responsible for blood filtration. It has a granular appearance due to the presence of numerous glomeruli. Renal medulla The renal medulla is the inner part of your kidney. It contains most of the nephrons with their glomeruli and renal tubules. The renal tubules carry urine to the renal pelvis. Renal papilla These pyramid-shaped structures transfer urine to the ureters. Renal pelvis This funnel-shaped structure collects urine and passes it down two ureters. Urine travels from the ureters to the bladder, where it’s stored. Renal vein This vein is the main blood vessel that carries filtered blood out of your kidneys and back to your heart. Each of your kidneys has a renal vein. In between pyramids → renal underneath space → Pyramids → minor calyces → major calyces → renal pelvis Vascularization Arterial Supply (to the Glomerulus) The abdominal aorta gives off many branches, including the renal arteries. The renal arteries branch off perpendicular to the abdominal aorta, travelling posterior to the renal veins, nerves and the pancreas. Subsequent to branching from the aorta, the renal artery enters the kidney at the hilum, where it divides into anterior and posterior branches. The posterior division goes on to supply the posterior region of the kidney, while the anterior branch (figure 1) divides further to produce apical, anterior superior, anterior inferior and inferior segmental arteries; each supplying their respective segments. At the level of the minor calyces, the branches of the anterior renal arteries further divide into interlobar arteries (figure 2) that course around the borders of the medullary pyramids. At the base of the pyramids, these arteries are referred to as arcuate arteries. Finally, the arteries enter the nephrons (functional units of the kidneys) as the interlobular arteries, where afferent arterioles bring blood to the glomerulus to be filtered. It should be noted that these arteries neither anastomose nor have accompanying veins. Interlobar Arteries: - The segmental arteries branch into interlobar arteries, running through the renal columns between the pyramids. Arcuate Arteries: - At the corticomedullary junction, interlobar arteries form arcuate arteries, arching over the base of the pyramids. Interlobular (Cortical Radiate) Arteries: - Arcuate arteries give rise to interlobular arteries, which extend into the cortex. Capillary network As the afferent arterioles enter the glomerulus, they form an intricate network of communicating capillaries. The capillaries are lined by a unique fenestrated epithelium (each space being around 70 - 100 nm wide). The fenestration allows selective passage of smaller particles into the renal tubules and keeps larger blood cells in the vessels. The capillaries leave the glomerulus as efferent arterioles, after which they form capillary beds around the nephron’s loop of Henle. - In cortical nephrons (loop of Henle does not extend deep into the medulla), the capillary beds are called peritubular capillaries; - while in juxtamedullary nephrons (loop of Henle extends deep into the medulla), the capillary beds are called vasa recta. These capillary beds facilitate blood pressure regulation and ionic homeostasis both passively and under hormonal influence. Venous Drainage (from the Capillaries) As the capillaries leave the nephron, they condense to form interlobular veins. Similar to the branches of the renal arteries, the interlobular veins become arcuate veins at the base of the medullary pyramids, then interlobar veins. About five or six interlobar veins join together to form each renal vein. Unlike the branches of the renal arteries, the tributaries of the renal vein communicate with each other. 2. What is the structure of the nephrons? A cross section through a kidney shows that the interior is arranged in two layers: an outer cortex and inner medulla. The layers are formed by the organized arrangement of microscopic tubules called nephrons. - About 80% of the nephrons in a kidney are almost completely contained within the cortex (cortical nephrons) - In cortical nephrons (loop of Henle does not extend deep into the medulla), the capillary beds are called peritubular capillaries; - The other 20% - called juxtamedullary nephrons - dip down into the medulla. The nephron is the functional unit of the kidney. (A functional unit is the smallest structure that can perform all the functions of an organ.) Each of the 1 million nephrons in a kidney is divided into sections, and each section is closely associated with specialized blood vessels. - while in juxtamedullary nephrons (loop of Henle extends deep into the medulla), the capillary beds are called vasa recta. The kidney tubule consists of a single layer of epithelia cells connected together near their apical surface. The apical surfaces are folded into microvilli or other area-increasing folds, and the basal side of the polarized epithelium rests on a basement membrane. The cell-cell junctions are mostly tight but some have selective permeability for ions. The nephron begins with a hollow, ball-like structure called Bowman’s capsule that surrounds the glomerulus. The endothelium of the glomerulus is fused to the epithelium of Bowman’s capsule so that fluid filtering out of the capillaries passes directly into the lumen of the tubule. The combination of glomerulus and Bowman’s capsule is called the renal corpuscle. From Bowman’s capsule, filtered fluid flows into the proximal tubule, then into the loop of Henle, a hairpin-shaped segment that dips down toward the medulla and then back up. The loop of Henle is divided into two limbs, a thin descending limb and an ascending limb with thin and thick segments. The fluid then passes into the distal tubule. The distal tubules of up to eight nephrons drain into a single larger tube called the collecting duct (The distal tubule and its collecting duct together form the distal nephron.) Collecting ducts pass from the cortex through the medulla and drain into the renal pelvis. From the renal pelvis, the filtered and modified fluid, now called urine, flows into the ureter on its way to excretion. The Renal Corpuscle Contains Filtration Barriers Filtration takes place in the renal corpuscle, which consists of the glomerular capillaries surrounded by Bowman’s capsule. Substances leaving the plasma must pass through three filtration barriers before entering the tubule lumen: - the glomerular capillary endothelium - a basement membrane sandwiched in the middle - the epithelium of Bowman’s capsule. The first filtration barrier is the capillary endothelium. Glomerular capillaries are fenestrated capillaries [p. 531] with large pores (fenestra) that allow most components of the plasma to filter through the endothelium. The luminal surface of the capillary and the pores are lined with a lattice-like layer of glycoproteins called the glycocalyx. The negatively charged proteins of the glycocalyx help repel negatively charged plasma proteins, and the endothelial pores are small enough to prevent blood cells from leaving the capillary. The second filtration barrier is the basement membrane, the acellular layer of extracellular matrix that separates the capillary endothelium from the epithelium of Bowman’s capsule (Fig. 19.5d). The basement membrane consists of negatively charged glycoproteins, collagen, and other proteins, and it too acts like a coarse sieve, excluding most plasma proteins from the fluid that filters through it. The third filtration barrier is the epithelium of Bowman’s capsule, consisting of specialized cells called podocytes (podos, foot) that surround each glomerular capillary (Fig. 19.5c). Podocytes have long cytoplasmic extensions called foot processes that extend from the main cell body (Fig. 19.5a, b). Foot processes wrap around the glomerular capillaries and interlace with one another, leaving narrow filtration slits closed by a slit diaphragm. The slit diaphragm contains several structural unique proteins, including nephrin and podocin, that seem to form a two-layer sieve. Glomerular mesangial cells lie between and around the glomerular capillaries, creating a support structure for the tuft of capillaries (Fig. 19.5c). - Mesangial cells influence filtration by altering the surface area of the filtration slits. - In addition, mesangial cells secrete cytokines associated with immune and inflammatory processes. 3. What is the general function of the kidneys? Main function → mass balance (water and ions Filtration and secretion − waste products (urea, creatinine, uric acid, bilirubin...) − toxins Homeostatic regulation − electrolytes, acid-base, osmolarity, volume, blood pressure Hormone production − Epo (erythropoietin), calcitriol, renin Gluconeogenesis Kidneys control excretion of - Water osmotic pressure of body fluid - NaCl volume of extracellular fluid - Acid acid-base balance of the body - Potassium K+ equilibrium - Urea nitrogen equilibrium Blood Pressure Regulation When low blood pressure → glomerular PH drops → GFR decreases Adrenaline Adrenaline, secreted by the adrenal medulla during stress or physical activity, directly influences blood pressure. It enhances sympathetic nervous system activity, causing: - Vasoconstriction of blood vessels, increasing vascular resistance and blood pressure. - Increased cardiac output by raising heart rate and stroke volume. - Stimulation of renal arterioles, which supports the release of renin and activates the RAAS, amplifying blood pressure regulation mechanisms. Angiotensin The kidneys regulate blood pressure through the renin-angiotensin-aldosterone system (RAAS): - Renin secretion: In response to low blood pressure, the juxtaglomerular cells of the kidneys release renin. - RAAS activation: Renin converts angiotensinogen (from the liver) into angiotensin I, which is then converted to angiotensin II by ACE (angiotensin-converting enzyme). - Angiotensin II causes vasoconstriction and stimulates aldosterone release, increasing sodium and water reabsorption, thus raising blood pressure. - Long-term control: The kidneys adjust blood volume by altering the excretion of sodium and water. Regulation of Water and Electrolyte Composition - The kidneys maintain fluid balance by adjusting water excretion based on body needs, mediated by antidiuretic hormone (ADH). - They regulate electrolyte concentrations (e.g., sodium, potassium, calcium) by controlling reabsorption or excretion in the nephron. - Sodium and potassium balance is influenced by aldosterone, which promotes sodium reabsorption and potassium excretion. Endocrine Regulation Erythropoietin (EPO): The kidneys produce EPO in response to low oxygen levels in the blood (hypoxia), stimulating the bone marrow to produce more red blood cells. Vitamin D Activation: The kidneys convert inactive vitamin D into its active form (calcitriol), which is crucial for calcium absorption in the intestines and bone health. → promoties calcium absorption in intestinal lumen Renin: As part of the RAAS, renin secretion indirectly regulates vascular resistance and blood volume. Gluconeogenesis The kidneys contribute to glucose homeostasis, especially during prolonged fasting or stress. They perform gluconeogenesis, synthesizing glucose from non-carbohydrate precursors such as amino acids and glycerol, providing energy when blood glucose levels are low. little more 4. What is the function of the nephrons? Kidneys Filter, Reabsorb, and Secrete Three basic processes take place in the nephron: filtration, reabsorption, and secretion (FIG. 19.2). Filtration is the movement of fluid from blood into the lumen of the nephron. Filtration takes place only in the renal corpuscle, where the walls of glomerular capillaries and Bowman’s capsule are modified to allow bulk flow of fluid. Once the filtered fluid, called filtrate, passes into the lumen of the nephron, it becomes part of the body’s external environment, just as substances in the lumen of the intestinal tract are part of the external environment [Fig. 1.2, p. 40]. For this reason, anything that filters into the nephron is destined for excretion, removal in the urine, unless it is reabsorbed into the body. After filtrate leaves Bowman’s capsule, it is modified by reabsorption and secretion. Reabsorption is the process of moving substances in the filtrate from the lumen of the tubule back into the blood flowing through peritubular capillaries. Secretion selectively removes molecules from the blood and adds them to the filtrate in the tubule lumen. Although secretion and glomerular filtration both move substances from blood into the tubule, secretion is a more selective process that usually uses membrane proteins to move molecules across the tubule epithelium. Filtration The filtration of plasma into the kidney tubule is the first step in urine formation. This relatively nonspecific process creates a filtrate whose composition is like that of plasma minus most of the plasma proteins. Under normal conditions, blood cells remain in the capillary, so that the filtrate is composed of water and dissolved solutes. When you visualize plasma filtering out of the glomerular capillaries, it is easy to imagine that all the plasma in the capillary moves into Bowman’s capsule. However, filtration of all the plasma would leave behind a sludge of blood cells and proteins that could not flow out of the glomerulus. - Instead, only about one-fifth of the plasma that flows through the kidneys filters into the nephrons. - The remaining four-fifths of the plasma, along with most plasma proteins and blood cells, flows into the peritubular capillaries. - The percentage of renal plasma flow that filters into the tubule is called the filtration fraction. Capillary Pressure Causes Filtration The three pressures that influence glomerular filtration - capillary blood pressure, capillary colloid osmotic pressure, and capsule fluid pressure - are summarized in FIGURE 19.6a. 1. The hydrostatic pressure (PH) of blood flowing through the glomerular capillaries forces fluid through the leaky endothelium. Capillary blood pressure averages 55 mm Hg and favors filtration into Bowman’s capsule. Although pressure decreases as blood moves through the capillaries, it remains higher than the opposing pressures. Consequently, filtration takes place along nearly the entire length of the glomerular capillaries. - Efferent arteriole is smaller than afferent → causes pressure in bowman's capsule 2. The colloid osmotic pressure (p) inside glomerular capillaries is higher than that of the fluid in Bowman’s capsule. This pressure gradient is due to the presence of proteins in the plasma. The osmotic pressure gradient averages 30 mm Hg and favors fluid movement back into the capillaries. 3. Bowman’s capsule is an enclosed space (unlike the interstitial fluid), and so the presence of fluid in the capsule creates a hydrostatic fluid pressure (Pfluid) that opposes fluid movement into the capsule. Fluid filtering out of the capillaries must displace the fluid already in the capsule lumen. Hydrostatic fluid pressure in the capsule averages 15 mm Hg, opposing filtration. The net driving force is 10 mm Hg in the direction favoring filtration. This pressure may not seem very high, but when combined with the very leaky nature of the fenestrated glomerular capillaries, it results in rapid fluid filtration into the tubules. The volume of fluid that filters into Bowman’s capsule per unit time is the glomerular filtration rate (GFR). Average GFR is 125 mL/min, or 180 L/day, an incredible rate considering that total plasma volume is only about 3 liters. This rate means that the kidneys filter the entire plasma volume 60 times a day, or 2.5 times every hour. If most of the filtrate were not reabsorbed during its passage through the nephron, we would run out of plasma in only 24 minutes of filtration! GFR is influenced by two factors: - the net filtration pressure - the filtration coefficient Filtration pressure is determined primarily by renal blood flow and blood pressure. The filtration coefficient has two components: (1) the surface area of the glomerular capillaries available for filtration (2) the permeability of the filtration slits In this respect, glomerular filtration is similar to gas exchange at the alveoli, where the rate of gas exchange depends on partial pressure differences, the surface area of the alveoli, and the permeability of the alveolar-capillary diffusion barrier. Where does secretion and reabsorption take place? reabsorption → everywhere except bowman's capsule Factors that affect the GFR Are factors that affect Kf or Starling forces, such as: - afferent arteriolar resistance: PGC - efferent arteriolar resistance: PGC - renal arteriolar pressure: PGC - plasma protein concentration: πGC - obstruction of urinary pathways: PBC - reduction in the number of nephrons: Kf Afferent arteriole determines filtration rate → vasoconstriction → less flow → less GFR Efferent arteriole determines → vasoconstriction → increased filtration 5. Autoregulation of GFR and renal perfusion GFR Is Relatively Constant Blood pressure provides the hydrostatic pressure that drives glomerular filtration. Therefore, it might seem reasonable to assume that if blood pressure increased, GFR would increase, and if blood pressure fell, GFR would decrease. That is not usually the case, however. Instead, GFR is remarkably constant over a wide range of blood pressures. As long as mean arterial blood pressure remains between 80 mm Hg and 180 mm Hg, GFR averages 180 L/day (Fig. 19.6b). GFR is controlled primarily by regulation of blood flow through the renal arterioles. If the overall resistance of the renal arterioles increases, renal blood flow decreases, and blood is diverted to other organs [Fig. 15.13, p. 528]. The effect of increased resistance on GFR, however, depends on where the resistance change takes place. - If resistance increases in the afferent arteriole (Fig. 19.6d), hydrostatic pressure decreases on the glomerular side of the constriction. This translates into a decrease in GFR. - If resistance increases in the efferent arteriole, blood “dams up” in front of the constriction, and hydrostatic pressure in the glomerular capillaries increases (Fig. 19.6e). Increased glomerular pressure increases GFR. The opposite changes occur with decreased resistance in the afferent or efferent arterioles. Most regulation occurs at the afferent arteriole. Autoregulation of glomerular filtration rate is a local control process in which the kidney maintains a relatively constant GFR in the face of normal fluctuations in blood pressure. One important function of GFR autoregulation is to protect the filtration barriers from high blood pressures that might damage them. The myogenic response is the intrinsic ability of vascular smooth muscle to respond to pressure changes. Tubuloglomerular feedback is a paracrine signaling mechanism through which changes in fluid flow through the loop of Henle influence GFR. Myogenic Response The myogenic response of afferent arterioles is similar to autoregulation in other systemic arterioles. - Smooth muscle in the arteriole wall stretches because of increased blood pressure - stretch-sensitive ion channels open - the muscle cells depolarize - Depolarization opens voltage-gated Ca 2+ channels - the vascular smooth muscle contracts. Vasoconstriction increases resistance to flow, and so blood flow through the arteriole diminishes. The decrease in blood flow decreases filtration pressure in the glomerulus. If blood pressure decreases, the tonic level of arteriolar contraction disappears, and the arteriole becomes maximally dilated. However, vasodilation is not as effective at maintaining GFR as vasoconstriction because normally the afferent arteriole is fairly relaxed. Consequently, when mean blood pressure drops below 80 mm Hg, GFR decreases. This decrease is adaptive in the sense that if less plasma is filtered, the potential for fluid loss in the urine is decreased. In other words, a decrease in GFR helps the body conserve blood volume. 6. Anatomy and function of juxtaglomerular apparatus Notice in Figure how the nephron twists and folds back on itself so that the final part of the ascending limb of the loop of Henle passes between the afferent and efferent arterioles. This region is known as the juxtaglomerular apparatus. The proximity of the ascending limb and the arterioles allows paracrine communication between the two structures, a key feature of kidney autoregulation. Tubuloglomerular Feedback Tubuloglomerular feedback is a local control pathway in which fluid flow through the tubule influences GFR. The twisted configuration of the nephron, as shown in FIGURE, causes the final portion of the thick ascending limb of the loop of Henle to pass between the afferent and efferent arterioles. The tubule and arteriolar walls are modified in the regions where they contact each other and together form the juxtaglomerular apparatus. The modified portion of the tubule epithelium is a plaque of cells called the macula densa (Fig. 19.7b). The adjacent wall of the afferent arteriole has specialized smooth muscle cells called granular cells (also known as juxtaglomerular cells or JG cells). The granular cells secrete renin, an enzyme involved in salt and water balance as well as paracrine signal molecules. - NaCl delivery past the macula densa increases as a result of increased GFR - the macula densa cells send a paracrine message to the neighboring afferent arteriole - JG cells → secrete renin → angiotensin 1 → angiotensin 2 → bind to receptors - The afferent arteriole constricts - increasing resistance and decreasing GFR Experimental evidence indicates that the macula densa cells transport NaCl, and that increases in salt transport initiate tubuloglomerular feedback. Flow can also be sensed in renal tubule cells by the primary cilia [p. 104], which are located on the apical surface facing the lumen. The renal primary cilia are known to act as flow sensors as well as signal transducers for normal development. extra juxtaglomerular mesangial cells