Case 2b - Nucleic Acids: From DNA to DNA and DNA to RNA PDF
Document Details
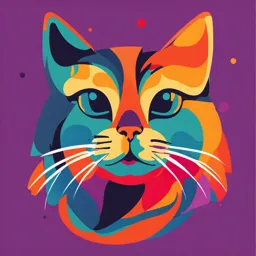
Uploaded by SupportingAutoharp5841
Maastricht University
Tags
Summary
This document provides an overview of nucleic acids, specifically focusing on RNA and the process of DNA transcription in different biological systems. Information includes descriptions of RNA structure and function, along with a comparison of RNA synthesis processes between prokaryotes and eukaryotes.
Full Transcript
Case 2b - Nucleic acids: from DNA to DNA and DNA to RNA 4. What is the function of RNA Primary function of RNA is to create proteins via translation. It carries genetic info that is translated by ribosomes to various proteins needed for cellular processes. 3 main types are: 1. mRNA → Transmits...
Case 2b - Nucleic acids: from DNA to DNA and DNA to RNA 4. What is the function of RNA Primary function of RNA is to create proteins via translation. It carries genetic info that is translated by ribosomes to various proteins needed for cellular processes. 3 main types are: 1. mRNA → Transmits information for protein synthesis - Rapidly degraded by nucleases 2. rRNA → Structure of ribosomal subunits, peptidyl transferase activity - Very stable molecules, majority of cellular RNA 3. tRNA → Brings amino acids to the ribosome - Very stable molecules Other functions: RNA editing, gene regulation, and RNA interference Production of rRNA - Production is in the nucleolus - rRNA transcription affects DNA structure - In a region where the DNA is not compacted as tightly as in the rest of the chromosome → genes responsible for rRNA → continuously transcribed so the region can’t be to compact 5. What is the structure of RNA - RNA contains a ribose = sugar - Uracil instead of thymine → only differ in a methyl group (uracil doesn't have it) → does not partake in base pairing → U+T can pair with A - Typical size: 60–20,000 bases - Could form double helix - Strand can fold back on itself → forms short antiparallel double-helical segments. - RNA molecules contain ‘stems’ and ‘loops’ Stem → base-paired portion Loop → unpaired portion - RNA can pair with DNA → DNA-RNA hybrid → called hybridization - Difference prokaryotes and eukaryotes: mRNA lives about 3 min in prokaryotes so more than 80% of the RNA in e-coli is rRNA and about 3% is mRNA Most human mRNAs live for 1 to 10 hours - In prokaryotes it mRNA can code for many proteins The primary structure of RNA is composed of nucleotides attached by 5’-3’ phosphodiester bonds between ribose sugars. Ribose has the molecular formula C5H10O5 and has a naturally occurring D-ribose form and a less common L-ribose. The base pairing via hydrogen bonds is the basis of RNA's secondary structure. The RNA tertiary structure is the result of RNA folding, which creates a three-dimensional shape consisting of helices and grooves. 6. How is DNA transcribed? Differences prokaryotes and eukaryotes There are significant differences in the steps of transcription in prokaryotes versus eukaryotes. - In prokaryotes (bacteria), transcription occurs in the cytoplasm. Translation of the mRNA into proteins also occurs in the cytoplasm. In eukaryotes, transcription occurs in the cell's nucleus. mRNA then moves to the cytoplasm for translation. - DNA in prokaryotes is much more accessible to RNA polymerase than DNA in eukaryotes. Eukaryotic DNA is wrapped around histones to form nucleosomes. Eukaryotic DNA is packed to form chromatin. While RNA polymerase interacts directly with prokaryotic DNA, other proteins mediate the interaction between RNA polymerase and DNA in eukaryotes. - mRNA produced as a result of transcription is not modified in prokaryotic cells. Eukaryotic cells modify mRNA by RNA splicing, 5' end capping, and addition of a poly A tail. Pre-initiation The first step of transcription is called pre-initiation. - RNA polymerase + cofactors (general transcription factors) bind to DNA and unwind it, creating an initiation bubble. - This space grants RNA polymerase access to a single strand of the DNA molecule. Approximately 14 (17) base pairs are exposed at a time. Initiation - The initiation of transcription in prokaryotes begins with the binding of RNA polymerase to the promoter in DNA. - Transcription initiation is more complex in eukaryotes, where a group of proteins called transcription factors mediates the binding of RNA polymerase and the initiation of transcription. Initiation prokaryotes σ (sigma) subunit RNA polymerase synthesizes RNA from the DNA. Start gene is marked by the promoter → first task of RNA polymerase is finding the promoter. The RNA polymerase of E. coli consists of a core enzyme with the subunit structure α 2 ββ′ω and a σ (sigma) subunit that is only loosely bound to the core enzyme. The σ subunit recognizes the promoter, and the core enzyme synthesizes RNA. Sigma factors are subunits of all bacterial RNA polymerases that are responsible for determining the specificity of promoter DNA binding and efficient initiation of RNA synthesis (transcription). The first sigma factor discovered was the sigma70 (σ70) of the highly studied bacterium Escherichia coli. It was found that the RNA polymerase exists in two forms. A core polymerase (with subunit structure α2ββ′ω) can transcribe DNA into RNA inefficiently and nonspecifically. When the sigma subunit, σ70, is added, it can bind to the core and form a holoenzyme (α2ββ’σω70) that is capable of specific binding at the beginning of genes (promoters) and efficient initiation of transcription. Sigma factor recognizes → -10 box: pribnow box and -35 box upstream - Upstream → in front of start codon - Downstream → after start codon It was hypothesized that multiple sigma factors would be found in E. coli, each capable of directing the core polymerase to transcribe a class of genes. In this way, by regulating the abundance of each active sigma factor, the cell could coordinately regulate groups of genes with common functions. Seven sigma factors are found. Promoters in prokaryotes - The promoters in E. coli have a length of about 60 base pairs, and they look quite different in different genes. - Only two short segments, located about 10 base pairs and 35 base pairs upstream of the transcriptional start site, are similar in all promoters. Even these sequences are variable, although we can define a consensus sequence of the most commonly encountered bases → somewhere between the -10 box and -35 box This diversity is required because genes must be transcribed at different rates. Some are transcribed up to 10 times per minute, but others are transcribed only once every 10 to 20 minutes. The rate of transcriptional initiation depends on the base sequence of the promoter. - In general, the more the promoter resembles the consensus sequence, the higher is the rate of transcription. The RNA polymerase then separates the DNA double helix over a length of about 18 base pairs, starting at a conserved A-T–rich sequence about 10 base pairs upstream of the transcriptional start site. Strand separation is essential because transcription, like DNA replication, requires a single-stranded template. The σ subunit separates from the core enzyme after the formation of the first 5 to 15 phosphodiester bonds. This marks the transition from the initiation phase to the elongation phase of transcription. The core enzyme now moves along the template strand of the gene while synthesizing the RNA transcript at a rate of about 50 nucleotides per second. Control of transcription in eukaryotes Role of topoisomerase - In front of transcription bubble + supercoiling - Before transcription bubble - supercoiling - Topoisomerases will make that the supercoils are removed by cutting the DNA Initiation eukaryotes Eukaryotes use separate enzymes for the synthesis of rRNA, mRNA, and tRNA: 1. RNA polymerase I synthesizes the common precursor of the 5.8S, 18S, and 28S rRNA in the nucleolus, where the ribosomal subunits are assembled. 2. RNA polymerase II synthesizes the mRNA precursors. 3. RNA polymerase III synthesizes small RNAs including tRNAs and the 5S rRNA. Eukaryotic promoters are extremely variable. The core promoter includes 30 to 40 base pairs upstream of the transcriptional start site. It serves as an assembly point for general transcription factors, which are functionally equivalent to the bacterial σ subunit. They are named by the acronym TF, followed by the number of the RNA polymerase with which they work and an identifying letter. The RNA polymerase must bind to the promoter-associated transcription factors before it can start transcription. A more frequently encountered promoter element is the TATA box (consensus TATAAAA), located 25 to 30 base pairs upstream of the transcriptional start site. Fig. 7.10 shows how the general transcription factors assemble on TATA box-containing promoters. - First the TATA-binding protein TBP binds to the TATA box. TBP is only one of about 14 subunits of transcription factor IID (TFIID). The other subunits, known as TBP-associated factors (TAFs), assemble on TBP while TBP is bound to the TATA box. - Other transcription factors add to this complex. - One of them, TFIIH, is composed of 10 subunits which include ATP-dependent helicases and a protein kinase. - Transcription can start when RNA polymerase II binds to the assembled transcription factors. - The helicase components of TFIIH separate the strands during transcription, and its kinase subunit phosphorylates RNA polymerase II on multiple serine and threonine side chains. - The phosphorylated RNA polymerase attracts proteins that are needed during the elongation phase of transcription, as well as those that modify the new mRNA. Only about 10% of human genes possess a TATA box, and there are many alternative ways of assembling a preinitiation complex on core promoters. For example, some of the TAFs bind not only to TBP but also to promoter elements other than the TATA box. DNA is copied in RNA (elongation) - RNA is synthesized 5’ → 3’ - ATP, GTP, CTP, and uridine triphosphate (UTP) are the precursors. - RNA polymerase does not need primer - Starts new chain by placing nucleotide in first position - Template strand of DNA is antiparallel and complementary to RNA - Coding strand is same as RNA (T replacing U) - RNA pol → no proofreading nuclease activity - Error rate is about 1:10,000, more than 1000 times higher than the error rate of poly III Template = antisense = plus = noncoding strand Non-template = sense = minus = coding strand Termination Prokaryotes Rho-factor - A ρ factor (Rho factor) is a bacterial protein involved in the termination of transcription - The main difference between Rho-dependent and Rho-Independent termination is the mechanism of transcription termination. In Rho-dependent termination, the Rho protein is responsible for termination, while the formation of a hairpin loop structure triggers Rho-independent termination. Rho-independent termination - Transcription continues until terminator sequence - Most contain a palindrome → “Madam, I’m Adam.” - When palindrome is transcribed → RNA-transcript forms a stem-loop structure → internal base pairing → RNA-polymerase dissociates from template and releases RNA The terminator region typically contains an inverted repetitive pattern, immediately followed by an Adenine-rich region (AAAA). The inverted repeat sequence region contains two areas that complement each other. This complementarity allows hydrogen bonding to form a hairpin loop structure. This structure halts the activity of RNA polymerase. A U-rich region follows this. In the U-rich regions, weak interactions exist between the U bases of the transcript and the A bases of the template. These weak Adenine-Uracil interactions cause the RNA transcript and DNA template to separate, destabilizing them. As a result, the transcript disconnects from the transcription site. - Doesn’t need energy Rho-dependent termination It is one of the two mechanisms used by prokaryotes to terminate transcription. The Rho factor protein, which has helicase activity, plays a crucial role in this process. The Rho protein binds to the RNA transcript and moves along with the RNA polymerase in a 5′-3′ direction. This movement facilitates the breaking of hydrogen bonds between the DNA template and the RNA transcript. As the Rho factor approaches the transcription bubble, it separates the DNA/RNA hybrid, releasing the transcript from the bubble and terminating the transcription. Eukaryotic termination At the end of mRNA sequence → termination sequences → proteins bind to these sequences (CPSF and CstF) → form loop 2 enzymes bind (CF1 and CF2) → cleave strand - Addition of polyA-tail is done by polyA polymerase (PAP) - PAP will start the synthesis of polyA-tail → stabilized by polyA binding proteins (PAB) - Will add 50-250 adenines to end of strand (3’ site) - On the 3’ site of where CF1 and CF2 cleaved the mRNA the polyA-tail was added - The other site → exonucleases will attach and degrade the rest of the mRNA still being synthesized → the degradation happens quicker than synthesis of mRNA so exonucleases will catch up - The 5’ cap binds with additional proteins to the polyA-tail → forms a loop Modifying RNA after transcription - Chemically modifying after transcription → post transcriptional processing In prokaryotes mRNA rarely processed: - It is translated as soon as it is synthesized, leaving no time for posttranscriptional modifications. In fact, ribosomes attach to the 5 ′ end of bacterial mRNA and start translation long before the synthesis of the mRNA has been completed In eukaryotes mRNA is processed extensively: ~ Removal of introns, exons stay ~ Ends of mRNA → modified to protect from nucleases + to guide mRNA into cytoplasm and to ribosomes - Post transcriptional processing occurs during transcription - Initiated by proteins that are recruited by phosphorylated RNA polymerase - It begins almost immediately after start of transcription with the covalent attachment of a modified guanine nucleotide to the 5ʹ end of the mRNA on the free 5’ site → guanine is placed in opposite direction → so 5’ site binds to 5’ site - This cap binds proteins that protect the mRNA from 5ʹ-exonucleases, guide the mRNA through the nuclear pore complex into the cytosol, mediate its binding to the ribosome, and regulate the start of translation - Next introns are removed → by spliceosomes - The spliceosome contains five small RNAs (U1, U2, U4, U5, and U6) with lengths between 106 and 185 nucleotides. These associate with proteins to form small nuclear ribonucleoprotein particles (snRNPs “snurps”). Overall, about 50 proteins are involved in splicing. - Alternative splicing → cutting the exons in different ways → 1 gene can code for more proteins - Finally, a polyadenylation signal (consensus AAUAAA) in the last exon recruits an endonuclease that cleaves the RNA about 20 nucleotides downstream. - This cut marks the end of the last exon, and a poly A tail of about 200 nucleotides is added enzymatically to the newly created 3ʹ end → ribonuclease degradation protection - The poly A tail binds multiple copies of a poly A binding protein (PABP), which retards the action of 3ʹ-exonucleases and allows the mRNA to survive for many hours or even a few days. - Only the histone mRNAs have no poly A tails, and consequently their half-lives are only a few minutes. Histones are synthesized only during S phase of the cell cycle when the DNA is replicated, and their synthesis must be switched off quickly once DNA replication is completed. Pre-mRNA 5’ prime cap → 5’ UTR (untranslated part) → start codon → introns → exons → introns → exons → stop codon → 3’ prime UTR → poly A tail mRNA 5’ prime cap → 5’ UTR (untranslated part) → start codon → coding segments → stop codon → 3’ prime UTR → poly A tail 7. The difference between DNA and RNA - Both carry genetic information - DNA double stranded structure → provides stability and protection - RNA single stranded structure → more chemically reactive and prone to being broken down by enzymes - The O-H bond in the ribose of RNA makes the molecule more reactive, compared with DNA. RNA is not stable under alkaline conditions, plus the large grooves in the molecule make it susceptible to enzyme attack. RNA is constantly produced, used, degraded, and recycled. - DNA is stable under alkaline conditions, while RNA is not stable. - DNA stores + transfers genetic info - RNA codes for amino acids + messenger between ribosome and DNA to make proteins - DNA is susceptible to UV damage. - Compared with DNA, RNA is relatively resistant to UV damage. - Size difference: DNA Often > 10^6 base pairs ; RNA 60–20,000 bases - Dntps DNA