Oxygen And Carbon Dioxide Transport In Blood (Queen Mary 2023 PDF)
Document Details
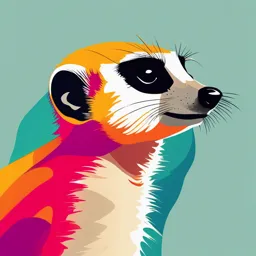
Uploaded by BrilliantVibrance
Queen Mary University of London
Dr Rosalin Bonetta Valentino
Tags
Related
- L1-Structure and Function of Haemoglobin PDF
- Week 1 - YR1 Lecture 1H - Blood Haemoglobin and Oxygen Transport - David Mahns 2019 PDF
- Protein Function, Regulation + O2 Transport PDF
- Respiratory Physiology - Oxygen-Hemoglobin Dissociation Curve Shift & Significance PDF
- Oxygen Saturation Notes PDF
- FSG120 Respiratory System Lecture Three 2024 PDF
Summary
This document contains lecture notes on oxygen and carbon dioxide transport in the blood. It includes learning objectives, the role of the cardiovascular system and explanations of oxidation and reduction.
Full Transcript
Oxygen and carbon dioxide transport in the blood Dr Rosalin Bonetta Valentino Learning Objectives 1. Describe the structure of haemoglobin and explain why it is uniquely suitable for carriage of oxygen in blood. 2. Explain the significance of the subunit structure of haemoglobin and the conseq...
Oxygen and carbon dioxide transport in the blood Dr Rosalin Bonetta Valentino Learning Objectives 1. Describe the structure of haemoglobin and explain why it is uniquely suitable for carriage of oxygen in blood. 2. Explain the significance of the subunit structure of haemoglobin and the consequences of mutations in the haemoglobin genes. 3. Comment on the significance of methaemoglobin in erythrocytes. 4. Draw a fully labelled diagram of an oxygen-haemoglobin dissociation curve. Explain how the curve shifts under the influence of temperature and pH. 5. Explain what is meant by the haematocrit and how it is regulated 6. Explain the ways in which carbon dioxide is transported by the blood. The role of the Cardiovascular System • To supply oxygen and metabolic fuel (eg. glucose) to tissues and take away the waste products of metabolism (eg. CO2). • To maintain defenses against invading micro-organisms. Carriage of oxygen is a problem because oxygen is a powerful oxidising agent; Most organic molecules are damaged by a very high a concentration of O2. Red cells (erythrocytes) are specially designed to carry this dangerous cargo! Oxidation and Reduction • A proper definition of oxidation is a loss of electrons. • Oxidising agents such as molecular oxygen are ‘electron hungry’ so that when they combine with other atoms or molecules they remove electrons from the oxidised molecule. • Oxidation is loss of electrons. It simplifies the electronic structure of the substrate. This decreases the free energy of the system and thus, releases energy, often in the form of heat. • Reduction is the opposite of oxidation: it is a process of gaining electrons. Building more complex molecules from simpler ones often involves reduction. • Reduction normally requires energy. Example of oxidation: Fe2+ Fe3+ + e- Oxidation and Reduction in the body • When oxidation releases a large amount of energy the reaction is generally irreversible. • On the other hand, if the electron transfer only involves a small amount of energy, the reaction may be reversible. Some of the most important oxidation reactions in your body are reversible. • A good example is the reaction between oxidised and reduced nicotinamide adenine dinucleotide (NAD) • This is a key reaction to remember: • This system is involved in many reactions that involve electron transfer in the body. NAD+ + H+ + 2e- NADH Oxidation and Reduction cont… • Living things are essentially ‘reduction engines’; they use energy to build complex (reduced and thus energy rich) molecules from simple (oxidised and thus energy poor) ones. • This process is reversed when complex molecules are ‘burned’ (i.e. oxidised to more simple structures) and release energy. • We ‘burn’ (i.e. remove electrons from) complex molecules like glucose to provide energy to build the complex molecules that make up our body. • The oxidation process requires oxygen as the electron acceptor. • Anaerobic bacteria use other oxidising agents such as sulfate, nitrate (NO3−), sulfur (S), etc. and can live in oxygen-poor environments. Erythrocytes contain the unique molecule… Haemoglobin • To be useful, an oxygen carrier must be able to bind oxygen reversibly. • Haemoglobin is a unique molecule because it can combine rapidly and reversibly with oxygen without becoming oxidised. It is found in erythrocytes, the red blood cells. • Normal erythrocytes are biconcave disks about 7 µm in diameter and about 2 µm thick. • A typical erythrocyte haemoglobin molecules. contains about 270 million • Mature red cells have no nuclei or mitochondria! • This may be because mitochondria are easily damaged by the high oxygen levels found in erythrocytes. Microcytic vs Macrocytic Anaemia • The sizes of red cells can vary in several common illnesses: • For example, if a red blood cell is smaller than usual - this occurs in microcytic anaemia. • If a red blood cell is larger than normal - this is macrocytic anaemia. Immature erythrocytes: Reticulocytes Just before and after leaving the bone marrow, immature red cells are known as reticulocytes. These comprise about 1-2% of circulating red blood cells in a normal person (but are a larger proportion after haemorrhage). Reticulocytes change into mature red cells in about a day after entering circulation. They are called reticulocytes because of a reticular (mesh-like) network of ribosomal RNA that becomes visible under a microscope with certain stains such as new methylene blue. Because of their lack of nuclei and organelles, the mature red blood cells cannot divide or repair themselves and so cannot survive for long. They are a kind of ‘cellular zombie’ Glucose transporters in Erythrocytes: GLUT1 • Because they have no mitochondria, the mature red cells cannot use any of the oxygen they transport; however, they do require small amounts of ATP to maintain the sodium pumps in their cell membranes and for other ion pumping operations. • Red blood cells produce ATP by glycolysis → This involves conversion of glucose to pyruvate followed by conversion of pyruvate to lactic acid. It is less efficient than aerobic metabolism. • Thus, red cells naturally have a low pH. • Red blood cells have a different glucose uptake system (Glut1) to other cells in the body: Glut1 works by facilitated diffusion and this uptake is not regulated by insulin. GLUT1 Transmembrane surface display PDB code: 4PYP Erythrocytes and oxidative damage • Red blood cells contain antioxidants (eg. Vitamin C) to protect them against oxidative damage (also taken up from the blood by Glut1). However, they are progressively damaged by the oxygen they carry. • The haemoglobin is gradually converted to methaemoglobin (oxidised haemoglobin). • The lack of repair ability means that the red cells have a limited lifetime; typically about 120 days. Summary: Erythrocyte life cycle • The ageing changes in erythrocyte its plasma undergoes membrane, making it susceptible to recognition by phagocytes and subsequent phagocytosis in the spleen, liver and bone marrow. The structure of Haemoglobin • Human haemoglobin is made up of four subunits, each with a haeme prosthetic group attached. • The four polypeptide chains are bound to each other by salt bridges, hydrogen bonds, and hydrophobic interactions. The exact way the subunits fit together is vital for proper oxygen transport. The three dimensional folding of the subunits creates ‘steric hindrance’ so the oxygen molecule cannot get physically close enough to the iron to remove the electron. Iron in the haemoglobin of Erythrocytes…is Hexavalent! • The ability to transport oxygen without being oxidised depends on the ability of the iron atom being hexavalent (form six bonds with surrounding atoms). • In ferrous iron (Fe2+), the atom has 6 unpaired ‘d’ orbital electrons (Fe is a transition metal) and can therefore form bonds with 6 electrons from other atoms. • 4 of the d orbital electrons are in a plane and 2 protrude above and below the plane. One orbital above plane Fe2+ One orbital below plane The structure of the Haeme group The four iron electrons in the plane are held by four covalent bonds to nitrogen atoms in a molecule called a porphyrin ring. The porphyrin ring together with its ferrous iron (Fe2+) centre is called a haeme group. Haeme group Porphyrin ring Structure of the Haeme cont… • A histidine group underneath the porphyrin ring binds a fifth iron electron. • This leaves one electron which can react with other molecules. • In haemoglobin, oxygen forms a weak reversible bond with the sixth ferrous electron in the haeme group. • The bond is weak as the oxygen molecule cannot get close enough to the iron to fully remove the electron due to steric hindrance from other parts of the haemoglobin molecule. Weak reversible bond Steric hindrance affects oxygen bonding • The result is that the oxygen is attracted to the iron but does not get close enough to oxidise it. • Oxygen bonding is critically dependent on the interlocking of the haemoglobin subunits and the partial pressure of oxygen in solution. • When the partial pressure is high (lungs), the oxygen binds and we have oxyhaemoglobin. • In the tissues, the oxygen dissociates to form deoxyhaemoglobin, which is transported back to the lungs for reoxygenation. Steric Hindrance from folded proteins Iron atom Space-filling model of haeme Oxygen Binding in Haemoglobin • Oxygen binding at the four haeme sites in haemoglobin does not happen simultaneously. • Once the first haeme binds oxygen, it introduces small changes in the structure of the corresponding protein chain. • These changes nudge the neighbouring chains into a different shape, making them bind oxygen more easily. • Thus, it is difficult to add the first oxygen molecule, but binding the second, third and fourth oxygen molecules gets progressively easier and easier. • If the steric hindrance is not quite right oxygen DOES oxidise the iron to its ferric state. • Haemoglobin with ferric iron is called methaemoglobin and cannot carry oxygen (the ferric iron in methaemoglobin no longer has the 6th electron to attract the oxygen molecule). Formation of methaemoglobin is one reason why red cells have a limited lifetime; they gradually accumulate methaemoglobin and then cannot carry oxygen (some repair can be done by the enzyme methaemoglobin reductase). • The steric hindrance that allows reversible bonding of oxygen to haeme iron but not actual oxidation of the iron is so finely balanced but gradual formation of methaemoglobin is unavoidable. • In newly formed released red cells, most of the methaemoglobin formed is converted back to haemoglobin by the NADH-dependent enzyme methaemoglobin reductase found inside the cell. • However, as the cells age, the amount of methaemoglobin increases. Methaemoglobinemia • Sampling venous blood shows that normally 1-2% of people's haemoglobin is methaemoglobin. • A higher percentage than this can be genetic or caused by exposure to various chemicals and is known as methaemoglobinemia. • One of the signals that the red cell should be removed from the circulation is thought to be a rise in the level of methaemoglobin in the cell, which causes markers on the surface of the red cell to change. • This change is detected by cells in the liver and spleen which remove the exhausted red cells. A Case of Methaemoglobinemia • There are a few rare individuals born with a congenital deficiency of methaemoglobin reductase. • There is a relatively high incidence of this trait among Alaskan Inuit and among some families in Appalachia. • These individuals may go through life with as much as half of their total haemoglobin in the form of methaemoglobin. As it turns out, however, they can manage oxygen transport to tissues quite well. • They compensate for the defect by making more red blood cells than normal individuals (polycythemia). • Thus, the total oxygen carrying capacity of the blood is increased. Sickle cell anaemia • The single mutation in β-globin chain of haemoglobin that causes sickle cell anaemia is E6V. • Sickle cell anaemia is caused by a single mutation in haemoglobin which causes a change in the shape of the RBC from biconcave to sickle deformation of the RBC. • The distorted cells are fragile and often rupture, leading to loss of haemoglobin. • This may seem like a terrible thing, but in one circumstance, it is actually an advantage. • The parasites that cause the tropical disease malaria, which spend part of their life cycle inside red blood cells, cannot live in the sickle cells. • Thus people with sickle cell haemoglobin are somewhat resistant to malaria. Learning Objectives 1. Describe the structure of haemoglobin and explain why it is uniquely suitable for carriage of oxygen in blood. ✓ 2. Explain the significance of the subunit structure of haemoglobin and the consequences of mutations in the haemoglobin genes. ✓ 3. Comment on the significance of methaemoglobin in erythrocytes. ✓ 4. Draw a fully labelled diagram of an oxygen-haemoglobin dissociation curve. Explain how the curve shifts under the influence of temperature and pH. 5. Explain what is meant by the haematocrit and how it is regulated 6. Explain the ways in which carbon dioxide is transported by the blood. Haemoglobin forms There are several different forms of the haemoglobin subunit (globin), each with a slightly different amino acid sequence. All subunits contain the haeme group, but the amount of steric hindrance varies depending on the particular mix of subunits in a particular molecule. • Adult haemoglobin is normally made up of two ⍺ subunits and two β subunits. • This is haemoglobin A, (⍺2β2). Other subunits can exist, for example the Υ subunit. • In the foetus and newborn there is foetal haemoglobin, with two Υ subunits (⍺2Υ2). • Foetal haemoglobin has a higher affinity for oxygen than the adult haemoglobin, and thus, can remove it from the placental blood. Oxygen Binding and Unloading • 2,3 DPG (2-3 diphosphoglycerate) is a small separate molecule bound loosely to the haemoglobin molecule. • When beta subunits start to deoxygenate, it binds to them more tightly, moves into the centre of the haemoglobin and increases the rate of oxygen release. • Thus, it enhances the ability of RBCs to release oxygen in hypoxic tissues. % Hb saturation • The proportion of haemoglobin that is bound to oxygen is called percent saturation and written as %Hb saturation or often for arterial blood as SaO2 (arterial oxygen saturation). This can be easily measured with a pulse oximeter. • Healthy individuals at sea level usually show oxygen saturation values between 96% and 99%, and should be above 94%. • An SaO2 value below 90% is hypoxaemia. Note that oxygen saturation does not directly reflect tissue oxygenation, as the ability to unload the oxygen in the respiring tissues determines the oxygen delivery. • Oxygen unloading is described by the Oxygen-haemoglobin dissociation curve. Cooperative Binding of Haemoglobin Oxygen dissociation curves show the relationship between oxygen levels (as partial pressure) and haemoglobin saturation. • Because binding potential changes with each additional O2 molecule, the saturation of haemoglobin is not linear. The oxygen/haemoglobin dissociation curve in Adults • The progressive binding of oxygen to the four subunits of haemoglobin is the reason for the characteristic shape of this curve. • You need to remember that this curve is ‘s’ shaped, flat at high pO2 and steep at medium and low pO2. Flat upper part of curve means that Hb is more than 90% saturated by oxygen over a wide range of pO2 in the lungs from 70 mm Hg to > 100 mm Hg. Steep middle part of curve means that Hb releases large amounts of oxygen for a small decrease in pO2 over the range 20 - 40 mm Hg. Effect of temperature on dissociation curve • Heavily metabolising tissue heats up; conversely, slowly metabolising tissue is colder than normal. • Heat moves the Hb curve to the right, thus unloading more oxygen at any given partial pressure. • Cold moves it sharply to the left. A cold limb may become hypoxic and feel fatigued even if well perfused. Hotter: unloads oxygen Effect of pH on dissociation curve • Heavily metabolising tissue generates more CO2 and thus becomes more acidic. • A lower pH also moves the curve to the right. • The effects of heat and acid are additive. • This pH-driven shift is called the BOHR SHIFT. MORE ACID: unloads oxygen • Myoglobin (Mb) is a form of haemoglobin found in muscle. It is a single subunit. • It has a greater affinity for oxygen than haemoglobin; therefore O2 is transferred to MbO2 as blood passes through muscle capillaries. • Mb, thus, forms a ‘buffer store’ of oxygen in muscle. Clinical note: When myoglobin is released from damaged muscle tissue the process is called rhabdomyolysis. The released myoglobin is filtered by the kidneys but is toxic to the renal tubular epithelium and so may cause acute renal failure. Learning Objectives 1. Describe the structure of haemoglobin and explain why it is uniquely suitable for carriage of oxygen in blood. ✓ 2. Explain the significance of the subunit structure of haemoglobin and the consequences of mutations in the haemoglobin genes. ✓ 3. Comment on the significance of methaemoglobin in erythrocytes. ✓ 4. Draw a fully labelled diagram of an oxygen-haemoglobin dissociation curve. Explain how the curve shifts under the influence of temperature and pH. ✓ 5. Explain what is meant by the haematocrit and how it is regulated 6. Explain the ways in which carbon dioxide is transported by the blood. What is the haematocrit? The amount of oxygen carried depends on the haematocrit (percentage of blood which is red blood cells: normally about 45%). Erythropoetin The haematocrit is controlled by a hormone erythropoetin (EPO), which is continually released from interstitial cells in the kidney; when the kidney is hypoxic, erythropoetin secretion is increased. Thus this is a negative feedback loop. There is some evidence that erythropoetin secretion is inhibited by a rise in pulmonary arterial pressure. • Synthetic Erythropoietin is available as a therapeutic agent. • It is used in treating anaemia resulting from chronic kidney disease, from the treatment of cancer (chemotherapy & radiation), and from other critical illnesses (heart failure). Red blood cell production in bone marrow Normal haematocrit = 45% Haematocrit falls Renal hypoxia EPO increase Stimulation Learning Objectives 1. Describe the structure of haemoglobin and explain why it is uniquely suitable for carriage of oxygen in blood. ✓ 2. Explain the significance of the subunit structure of haemoglobin and the consequences of mutations in the haemoglobin genes. ✓ 3. Comment on the significance of methaemoglobin in erythrocytes. ✓ 4. Draw a fully labelled diagram of an oxygen-haemoglobin dissociation curve. Explain how the curve shifts under the influence of temperature and pH. ✓ 5. Explain what is meant by the haematocrit and how it is regulated. ✓ 6. Explain the ways in which carbon dioxide is transported by the blood. CO2 transport by blood • Red blood cells also carry carbon dioxide, a major metabolic product, back to the lungs. • Although CO2 is very soluble in water, this solubility is not enough to carry all the CO2 generated by the body back to the lungs. • The red blood cells convert the CO2 to bicarbonate via an enzyme found inside the cell, carbonic anhydrase (CA). • Most of the bicarbonate that is formed is expelled into the plasma and carried in the venous blood to the lungs. • As the bicarbonate diffuses out, chloride ions diffuse into the red cell to maintain electrical neutrality. CO2 CA HCO3- Cl- In RBCs: HCO3- out HCO3- Cl- • In the lungs, bicarbonate re-enters the red cells and is converted back to CO2 and then released into the alveoli. • Chloride leaves the red cell to balance the electrical charge of the bicarbonate entering the cell. CO2 CA HCO-3 Cl- In lungs: CO2 & chloride out HCO-3 Cl- • CO2 is also carried to the lungs in the form of carbaminohaemoglobin. • CO2 can bind to the oxyhaemoglobin and displace oxygen in acid conditions. HbCO2 • This transport however, is not as important as the transport of bicarbonate. Low pH CO2 HbO2 O2 Oxygen CO2 • In the lungs, the high partial pressure of oxygen and high pH displaces the CO2 from the haemoglobin and oxyhaemoglobin is reformed. HbCO2 High pH HbO2 Summary diagram: Gas exchange in tissues Oxygen release and carbon dioxide pickup at the tissues In the lungs: O2 loaded/CO2 unloaded Most CO2 is carried back to the lungs in the form of bicarbonate, not as dissolved gas. Oxygen pickup and carbon dioxide release in the lungs Learning Objectives 1. Describe the structure of haemoglobin and explain why it is uniquely suitable for carriage of oxygen in blood. ✓ 1. Explain the significance of the subunit structure of haemoglobin and the consequences of mutations in the haemoglobin genes. ✓ 2. Comment on the significance of methaemoglobin in erythrocytes. ✓ 3. Draw a fully labelled diagram of an oxygen-haemoglobin dissociation curve. Explain how the curve shifts under the influence of temperature and pH. ✓ 4. Explain what is meant by the haematocrit and how it is regulated. ✓ 5. Explain the ways in which carbon dioxide is transported by the blood. ✓