Cardiac Physiology PDF
Document Details
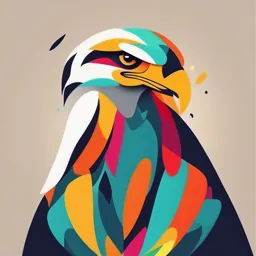
Uploaded by SufficientAnecdote1097
IMC Fachhochschule Krems
Tags
Summary
This document provides an overview of cardiac physiology. It explains the concepts of systole and diastole, essential for understanding the heart's function. It also details the electrical conduction system and its role in coordinating contractions.
Full Transcript
CARDIAC PHYSIOLOGY Systole The contraction of the cardiac muscle tissue in the ventricles is called systole. When the ventricles contract, they force the blood from the chambers into the arteries leaving the heart. The left ventricle empties into the aorta, and the right ven...
CARDIAC PHYSIOLOGY Systole The contraction of the cardiac muscle tissue in the ventricles is called systole. When the ventricles contract, they force the blood from the chambers into the arteries leaving the heart. The left ventricle empties into the aorta, and the right ventricle into the pulmonary artery. The increased pressure due to the contraction of the ventricles is called systolic pressure. Diastole The relaxation of the cardiac muscle tissue in the ventricles is called diastole. When the ventricles relax, they make room to accept the blood from the atria. The decreased pressure due to the relaxation of the ventricles is called diastolic pressure. A heartbeat is a two-part pumping action that takes about a second. As blood collects in the upper chambers (the right and left atrium), the heart's natural pacemaker (the SA node) sends out an electrical signal that causes the atria to contract. This contraction pushes blood through the tricuspid and mitral valves into the resting lower chambers (the right and left ventricles). This part of the two-part pumping phase (the longer of the two) is called the diastole. The second part of the pumping phase begins when the ventricles are full of blood. The electrical signals from the SA node travel along a pathway of cells to the ventricles, causing them to contract. This is called systole. As the tricuspid and mitral valves shut tight to prevent a back flow of blood, the pulmonary and aortic valves are pushed open. While blood is pushed from the right ventricle into the lungs to pick up oxygen, oxygen-rich blood flows from the left ventricle to the heart and other parts of the body. After blood moves into the pulmonary artery and the aorta, the ventricles relax, and the pulmonary and aortic valves close. The lower pressure in the ventricles causes the tricuspid and mitral valves to open, and the cycle begins again. This series of contractions is repeated over and over again, increasing during times of exertion and decreasing while you are at rest. Ventricular systole and diastole – Summary Contraction of the ventricles causes blood to move to the lungs and to the body. Pressure generated by the right ventricle (blood to the lungs) is lower than than pressure generated by the left ventricle (blood to the body). During the period of isometric contraction, ventricular contraction causes an increase in pressure within the ventricles but no movement of blood out of the ventricles. Blood flows out of the ventricles during the period of ejection. Valves of the aorta and pulmonary artery close, ventricles relax, and pressure drops to 0 during the early stages of diastole and the ventricles begin to fill with blood from the caval veins The cells in the cardiac conduction are excitable meaning that they have the ability to respond to an electrochemical stimulus. Electrochemical stimulation makes the cell membrane permeable to the flow of sodium, potassium and calcium ions. The action potential for ventricular muscle is 105mV, meaning that the membrane potential must change from approximately -90mV to approximately +20mV, a difference of about 110mV. Electrical impulses from your heart muscle (the myocardium) cause your heart to contract. This electrical signal begins in the sinoatrial (SA) node, located at the top of the right atrium. The SA node is sometimes called the heart's "natural pacemaker." An electrical impulse from this natural pacemaker travels through the muscle fibers of the atria and ventricles, causing them to contract. Although the SA node sends electrical impulses at a certain rate, your heart rate may still change depending on physical demands, stress, or hormonal factors. The Sinoatrial Node (often called the SA node or sinus node) serves as the natural pacemaker for the heart. Nestled in the upper area of the right atrium, it sends the electrical impulse that triggers each heartbeat. The impulse spreads through the atria, prompting the cardiac muscle tissue to contract in a coordinated wave-like manner. The impulse that originates from the sinoatrial node strikes the Atrioventricular node (or AV node) which is situated in the lower portion of the right atrium. The atrioventricular node in turn sends an impulse through the nerve network to the ventricles, initiating the same wave-like contraction of the ventricles. The electrical network serving the ventricles leaves the atrioventricular node through the Right and Left Bundle Branches. These nerve fibers send impulses that cause the cardiac muscle tissue to contract. On the posterior part of the right atrial wall, adjacent to the junction of the superior vena cava and the right atrium, is a specialized conduction system cell area called the sinoatrial node (SA node). It is horseshoe shaped and located just beneath the epicardium. Although the depolarization of the SA node is the first step of the cardiac cycle, it does not produce enough energy to be recorded by the electrocardiograph. The depolarization wave spreads down these specialized cardiac conduction system fibers over the atria by way of internodal and interatrial pathways known as Bachmann's bundle. These specialized fibers transmit impulses six times faster than do ordinary cell-to-cell interconnections. These pathways trigger the contraction of other cells in the atrium more rapidly than if the depolarization wave followed the cell-to-cell interconnections. The atrioventricular node, or AV node, is made up of another cluster of specialized cardiac conduction system cells. The AV node forms a pathway for impulse conduction that bridges between the atria and ventricles. Depolarization of the AV node is relatively slow due to the intrinsic characteristics of its cells. This causes a delay in the transmission of the depolarization wave to the ventricles. The transmission of the wave through the AV node is relatively weak, thus is considered silent on the electrocardiogram. The depolarization wave leaves the AV node and enters a compact tract of cardiac conduction system fibers known as the bundle of HIS. The bundle of His proceeds down the interventricular septum to the inferior border of the membranous septum where it bifurcates forming the right and left bundle branches The right bundle branch spreads the electrical impulse to the right ventricle. The left bundle branch spreads the electrical impulse to the interventricular septum and left ventricle. The left bundle branch divides into two fascicles, an anterior and a posterior one. Electro Cardio Graphy ( ECG/ EKG) Indicates atrial depolarization. The spread of the impulse from the S-A node through the two atria. A fraction of a second after the P- wave begins, the atria contract. The P-wave is associated with atrial depolarization. Normal P-waves last from 0.06 to 0.11 seconds and should not exceed 2.0-2.5 mm in height. Dysfunctions of the sinoatrial node result in the observance of abnormalities in the P-wave; (i.e., longer, wider or absent), when viewed on an ECG (EKG). The P-R interval represents conduction of electricity from the sinoatrial node to the atrioventricular node. It also represents atrial depolarization through the AV node. The P-R interval is measured from the beginning of a P-wave to the beginning of the QRS complex. Normal lengths of time for the P-R interval range from 0.12- Begins as a downward deflection, continues as a large, upright, triangular wave and ends as a downward wave at its base. Represents ventricular depolarization- the spread of the electrical impulse through the ventricles. Shortly after the QRS wave begins, the ventricles undergo contraction. The QRS complex is the result of ventricular depolarization through the Bundle Branches and Purkinje fibers. The length of the normal QRS complex should be no longer than 0.10 seconds. The S-T segment represents the ventricles in an active contraction state but with no electrical activity occurring. The S-T segment is measured from the end of the QRS complex to the beginning of the T-wave. The T- wave represents ventricular repolarization and is normally upright. Stroke Volume Stroke volume is directly related to the force of ventricular contraction. The demands placed upon the body will directly affect cardiac output in two ways. Cardiac output will increase if the blood volume within the body is increased or if the heart beats faster. Cardiac output will decrease if either of these factors decrease. A normal, healthy heart ejects about 60% of the blood volume present in the ventricle. Stroke volume represents the difference between end diastolic volume (EDV), the amount of blood collected in a ventricle during diastole, and end systolic volume (ESV), the amount of blood remaining in the ventricle after contraction. These volumes are used to determine normal cardiac output. Cardiac Output Cardiac output is the amount of blood that passes an arbitrary point in the circulatory system in a given time. It is the amount of blood pumped by each ventricle per unit of time. In a normal person at rest cardiac output is approximately 5,000 ml/min (5 l/min). Cardiac output is defined as the amount of blood that is ejected by one ventricle in a one minute period of time.Since the volume of blood passing through the left ventricle must equal the volume passing through the right ventricle in a normal person without any shunts, cardiac output can be determined from either ventricle. Mathematically, cardiac output is determined by multiplying stroke volume for one beat (the amount of blood ejected by a ventricle) times the heart rate (beats per minute). Stroke volume is the difference between the end diastolic volume and end systolic volume. (the amount of blood pumped by the heart per beat) Cardiac Output = Stroke Volume x Heart Rate Resting college student mL/beat x 72 beats/min. = 5040 mL/min or 5 L/min. Exercising college student mL/beat x 120 beats/min = 24000 mL/min or 24 L/min. EXTRINSIC REGULATION OF THE HEART Nerval ( sympathetic & parasympathetic ) R. Humoral R.: Vasodilating or –constricting agents, ions, and blood gas ) Blood pressure Body temperature Sympathetic Control Known as the "fight or flight" mechanism the sympathetic nervous system originates in the thoracic and lumbar regions of the spinal cord. Most postsynaptic sympathetic nerve endings release adrenaline or norepinephrine. Sympathetic stimulation of the heart has two effects: (1) it increases the heart rate (250 bpm with maximal stimulation); and (2) it increases the force of contraction which decreases the duration of systolic contraction and allows more time for filling during diastole. The innervation of the small arteries and arterioles allows sympathetic stimulation to increase the resistance thereby changing the rate of blood flow through the tissues. The innervation of the large vessels (particularly of the veins) makes it possible for sympathetic stimulation to change the volume of these vessels and alter the volume of the peripheral circulatory system. This leads to an increase in vascular resistance and therefore blood pressure. Parasympathetic Control The parasympathetic system consists of the vagus nerve which originates in the medulla of the brain and branches to the heart and digestive organs. Parasympathetic stimulation decreases heart rate and the force of cardiac muscle contraction. Under parasympathetic stimulation, heart rate can go as low as 20-30 bpm. Postsynaptic nerve endings of the parasympathetic system release acetylcholine. Humoral Regulation of Circulation Humoral regulation refers to control of circulation by substances already in the bloodstream. Some substances are formed by special glands and then transported by the blood throughout the body. Others are formed in local tissue areas and cause only local circulatory effects. 1. Vasoconstricting Agents Epinephrine and norepinephrineare secreted by the adrenal medulla when the sympathetic nervous system is stimulated. All vascular beds of the body are affected by these vasoconstricting agents. Another, angiotensin, is one of the most powerful vasoconstricting agents. A decrease in arterial pressure will cause the kidneys to secrete renin, which in turn acts to split away angiotensin from its precursor, angiotensinogen. Vasopressin(also called anti-diuretic hormone) formed in the hypothalamus, is a very powerful vasoconstrictor but also helps the body regulate fluid volume by controlling water reabsorption in the renal tubules. 2. Vasodilating Agents Bradykinin can form anywhere in the circulatory system. It arises from plasma or tissue fluids and is a very powerful arteriolar dilator. It also increases capillary permeability which can lead to peripheral edema. Serotonin is present in the intestines and platelets. It can have either a vasodilating or vasoconstricting effect depending on the conditions or the area of circulation. Histamine can be found in virtually every tissue of the body and is derived from mast cells in the tissues and basophils in the blood. It is a powerful vasodilator of arterioles and also increases capillary porosity, allowing leakage of both fluids and plasma proteins into the surrounding tissues causing local edema. It is responsible for allergic reactions. Prostaglandins (primarily vasodilators) are also found in almost every tissue of the body. 3. Effect of pH, CO2 and O2 Chemoreceptors in the brain and carotids monitor blood CO2, O2, and pH levels. In response to increased CO2, decreased pH, or decreased O2, autonomic nervous system reflexes increase sympathetic stimulation and decrease parasympathetic stimulation. 4. Effect of Extracellular Ion Concentration An increase or decrease in extracellular K+ decreases heart rate. Increased extracellular Ca2+ increases the force of contraction of the heart and decreases the heart rate. Decreased Ca2+ levels produce the opposite effect. 4. Effect of Blood Pressure Baroreceptors (sensory receptors are in the walls of certain large arteries i.e.Internal carotids and the aorta)--function to measure blood pressure. In response to an increase in blood pressure, the baroreceptor reflexes decrease sympathetic stimulation and increase parasympathetic stimulation. An increase in right atrial pressure also causes the cardioregulatory center to increase the heart rate through a reflex called the Bainbridge Reflex. 5. Effect of Body Temperature Heart rate increases when body temperature increases and decreases when bodytemperature decreases.