Cardiac I Study Questions PDF
Document Details
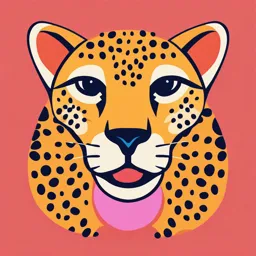
Uploaded by TrustyFantasy
Tags
Summary
This document contains study questions about the cardiovascular system, covering topics such as the path of blood through the heart, heart valves, and the pericardial sac. It's suitable for undergraduate-level study.
Full Transcript
Study Questions Normal Function of the Cardiovascular System 1) Describe in detail (including valves) the path that blood takes as it enters the heart from the Vena cava until its final entrance into the Aorta. Blood first enters the right atrium through superior and inf...
Study Questions Normal Function of the Cardiovascular System 1) Describe in detail (including valves) the path that blood takes as it enters the heart from the Vena cava until its final entrance into the Aorta. Blood first enters the right atrium through superior and inferior vena cava carrying deoxygenated blood, then passes through the right AV valve (Tricuspid Valve) into the right ventricle. From there, it moves through the pulmonary valve into the pulmonary artery and enters the pulmonary circulation. In the pulmonary capillaries, the blood gets oxygenated and returns to the heart through the pulmonary veins. It then flows into the left atrium, passes through the left AV valve into the left ventricle (generates a lot of pressure) and finally moves through the aortic valve into the aorta throughout the body. First Heartbeat (Pulmonary Circulation): Right Atrium → Right Ventricle → Pulmonary Arteries: Deoxygenated blood from the body enters the right atrium, moves into the right ventricle, and is pumped through the pulmonary arteries to the lungs. Gas Exchange in Lungs: Blood is oxygenated in the lungs. Second Heartbeat (Systemic Circulation): Left Atrium → Left Ventricle → Aorta: Oxygenated blood returns to the left atrium, enters the left ventricle, and is pumped into the aorta, delivering oxygen to the rest of the body. 2) Review the structure of the heart valves. Between which two structures do we find valves? Between which two structures in the heart do we NOT find valves? Atrioventricular (AV) valves: These are located between the atria and the ventricles. (a) Tricuspid valve: Between the right atrium and right ventricle. (b) Mitral (bicuspid) valve: Between the left atrium and left ventricle. Semilunar valves: These are located between the ventricles and the arteries that carry blood away from the heart. (c) Pulmonary valve: Between the right ventricle and pulmonary artery. (d) Aortic valve: Between the left ventricle and the aorta. There are no valves between the Veins and atria: Blood flows from the superior and inferior vena cava into the right atrium, and from the pulmonary veins into the left atrium without any valves. 3) Describe the structure and function of the pericardial sac? The Pericardial sac surrounds the heart and has two layers: the Epicardium, which is the inner layer against the heart, and the Pericardium, the outer fibrous layer. Both layers produce fluid that fills the pericardial cavity. The primary function of the pericardial sac is to reduce friction as the heart beats, preventing damage from rubbing against nearby structures in the thoracic cavity. 4) What are the unique structural features of cardiac muscle? Cardiac Muscle is not under voluntary control. Striation: Linear muscle lines along muscle cell. They are intracellular proteins that organize themselves in a particular pattern that’s responsible for generating a force when the muscle contracts. *Skeletal Muscle is also striated muscle* Single Central Nucleus: Most cardiac muscle cells have a single, centrally located nucleus Branching Fibers: Cardiac muscle fibers are branched, allowing them to form a network that enables the heart to contract as a single unit. Intercalated Discs: Junction points between one cardiac cell to the next Gap Junctions: These enable rapid electrical communication between cells (When we have gap junctions between the cells it's referred to as being electrically coupled because what happens to one cell happens to the other) Desmosomes: Protein Anchors. Providing strong mechanical attachments between cells. An example is: Action potential is triggered and then a muscle contraction is triggered it then rapidly spreads in order to contract all at once and act as a single unit) Think one heartbeat! 5) Consider the gap junctions located in the intercalated disks of cardiac muscle. Think about some consequences of selective blockade of those junctions. Action potential would not move through the heart and have isolated contractions would look like defibrillation (more like quivering than contraction) Disrupted Synchronization: The heart's ability to contract as a single, coordinated unit would be impaired. Different regions of the heart might contract at different times, leading to inefficient pumping of blood. Arrhythmias: Irregular heartbeats, or arrhythmias, could develop due to the lack of coordinated electrical signals between cells. Reduced Efficiency: Without proper electrical communication, the heart may not pump blood effectively, reducing cardiac output and potentially leading to cardiac arrest. 6) Describe and explain the ionic basis of the action potential in the autorhythmic cells in the heart. Autorhythmic cells (self-generating) are 1% of cells in the heart they are responsible for initiating and coordinating the heartbeat. These cells generate spontaneous action potentials that trigger the contraction of cardiac muscle. They affect the 99% of the contractile muscle cells. 1. Theres No Resting State (-60mV) because of sodium leak channels: No voltage-gated ion channels are open yet. 2. Slow Depolarization (Pacemaker Potential): Leak Sodium Channels: Slowly allow sodium ions (Na+) into the cell, gradually increasing the membrane potential (slow depolarization). Transient Calcium Channels (T-type): Open briefly as the membrane potential gets close to threshold, allowing a small influx of calcium ions (Ca++) to push the cell towards the threshold. 3. Rapid Depolarization: Long-lasting Calcium Channels (L-type): Once the threshold is reached, these channels open, causing a fast influx of calcium ions (Ca++), leading to a sharp rise (rapid depolarization) in membrane potential. 4. Repolarization: Potassium Channels: At the peak of the action potential, calcium channels close and potassium channels open. Potassium ions (K+) leave the cell, restoring the negative membrane potential (repolarization). This process repeats, setting the pace for the heart's rhythmic contractions. 7) Describe and explain the ionic basis of the action potential in the cardiac muscle cells? Needs stimulation and has a “Plateau phase” another difference is that the action potential is much wider. There’s no hyperpolarization phase. 1. Phase 4: Resting Membrane Potential o The cardiac muscle cell maintains a stable, negative membrane potential at rest. 2. Phase 0: Rapid Depolarization o Upon stimulation Sodium Channels Open: Sodium ions (Na+) rapidly enter the cell, causing a sharp increase in membrane potential (depolarization). 3. Phase 1: (Blip) Early Repolarization o Immediately following the rapid depolarization, Sodium Channels Close: The membrane potential drops slightly after sodium channels close. 4. Phase 2: Plateau Phase o Calcium Channels Open: Calcium ions (Ca++) enter the cell. o Potassium Channels Open: Potassium ions (K+) leave the cell. o The opposite movement of calcium and the of potassium create plateau that maintains a sustained depolarization o Significance of the Plateau Phase: Cardiac cells are electrically coupled to each other. The purpose for the cells to fire action potentials at the same time resulting in them contracting at the same time. Having a wider/longer action potential gives neighboring cells enough time for this action potential to spread throughout the heart. 5. Phase 3: Repolarization o Calcium Channels Close: The calcium influx stops. o Potassium Channels Fully Open: Potassium ions continue to leave the cell, restoring the negative membrane potential (repolarization). 8) Where are the autorhythmic cells located in the heart? What is the direction of flow of the action potential? 3 Areas where cells are located 1. Sinoatrial (SA) Node: This is in the right atrium near the superior vena cava and acts as the heart's natural pacemaker. 2. Atrioventricular (AV) Node: Located at the junction between the atria and the above the ventricles, near the interatrial septum (Center of the heart). 3. Left and right Bundle of His (Intraventricular septum): This arises from the AV node and branches into the left and right bundle branches, running along the interventricular septum. Purkinje Fibers: These fibers extend from the bundle branches and spread throughout the ventricular muscle. Direction of the action potential flow: 1. SA Node Initiation: The action potential originates in the SA node because it’s the fastest and spreads across the atria, causing atrial contraction. Spreads to three main directions simultaneously: To the left atrium via the interatrial pathway (fibers that connect the SA node to the left atrium). To the right atrium and the rest of the atrial mass, leading to full atrial contraction. To the AV node (atrioventricular node) via the intranodal pathway (fibers that connect the SA node to the AV node). 2. AV Node Delay: The signal reaches the AV node, where it is delayed for 100 milliseconds to allow the atria to fully contract and allow for maximal ventricular filling. 3. Bundle of His: After the AV node fires, the signal spreads through the rest of the ventricles through the Bundle of His and splits into the left and right bundle branches. 4. Purkinje Fibers: Finally, the action potential spreads through the Purkinje fibers, causing the ventricles to contract almost simultaneously. 9) Why is the Sinoatrial node considered the pacemaker of the heart? What area of the heart would become the pacemaker if the SA node stopped functioning? The sinoatrial (SA) node is considered the pacemaker of the heart because it has the fastest natural firing rate among all the autorhythmic cell groups in the heart. SA node spontaneously generates action potentials at a higher frequency (70-80 beats per minute) than the atrioventricular (AV) node (40-60 beats per minute) or the Bundle of His and Purkinje fibers (20-40 beats per minute) If the SA node stops functioning, the AV node would take over as the pacemaker of the heart because it’s the second fastest. 10) What is the purpose of the AV nodal delay? The AV nodal delay allows the atria to contract and fully empty their blood into the ventricles before the ventricles begin to contract. If the ventricles contracted simultaneously with the atria, they wouldn't have the opportunity to fill completely, reducing heart's pumping efficiency. This ensures that the ventricles receive the maximum amount of blood before they contract, optimizing the amount of blood ejected with each heartbeat. 11) If you were to cut off all parasympathetic and sympathetic innervation to the heart, what would heart rate be? Explain why? If all parasympathetic and sympathetic innervation to the heart were cut off, the heart rate would be determined by the intrinsic firing rate of the sinoatrial (SA) node. This is because the SA node, located in the right atrium, has the fastest natural firing rate among all the autorhythmic cell groups in the heart. Without any external input from the nervous system, the SA node would continue to spontaneously generate action potentials at its inherent rate of approximately 70- 80 beats per minute. Even though the heart receives input from both the sympathetic and parasympathetic branches of the autonomic nervous system, these inputs primarily serve to modulate the intrinsic heart rate set by the SA node. 12) Explain what electrical events happening in the heart generate the waveforms seen in a 12 lead EKG. P Wave: The P wave represents atrial depolarization, which occurs when the SA node generates an action potential. This signal spreads through the atria, leading to atrial contraction, pushing blood into the ventricles. PR Interval: The PR interval captures the time it takes for the electrical impulse to travel from the SA node, through the atria, and to the AV node. The delay at the AV node (AV nodal delay) allows the ventricles to fill with blood before they contract. QRS Complex: The QRS complex represents ventricular depolarization. This is the electrical signal spreading rapidly through the ventricles (via the Bundle of His and Purkinje fibers) causing ventricular contraction (ventricular systole), which pumps blood to the lungs (right ventricle) and the body (left ventricle). ST Segment: The ST segment is the flat part of the EKG after the QRS complex, representing the time during which the ventricles are still depolarized and contracting (sustained contraction to eject blood). It indicates the plateau phase of the action potential in the cardiac muscle cells. T Wave: The T wave represents ventricular repolarization, where the electrical signal returns the ventricular muscle to its resting state, allowing the ventricles to relax (ventricular diastole) and refill with blood. TP Interval: This interval occurs between the end of the T wave and the start of the next P wave. It represents diastole, where both the atria and ventricles are filling with blood. 13) If you were to look at a series heart cycles on an EKG, the distance between which two waveforms would decrease if heart rate increased? The distance between any two points will decrease if the HR increases. Example the distance between R to R decreases if the Heart rate increases. 14) Review all the electrical and mechanical events of the cardia cycle. 1. Atrial Systole (Electrical: P Wave) Electrical Event: The SA node fires, causing atrial depolarization, which corresponds to the P wave on the EKG. Mechanical Event: Atria contract (atrial systole), pushing blood into the ventricles. The AV valves (tricuspid and mitral) are open, and blood flows from the atria into the ventricles. Ventricular Volume: Ventricular volume increases as they fill, reaching End Diastolic Volume (EDV). 2. Isovolumetric Contraction (Electrical: QRS Complex) Electrical Event: Ventricular depolarization, corresponding to the QRS complex. Mechanical Event: The ventricles begin to contract, causing the AV valves (tricuspid and mitral) to close (S1 heart sound, "lub"). No blood is ejected because the pressure has not yet exceeded the aortic or pulmonary artery pressures. All valves are closed during this phase. Ventricular Volume: Ventricular volume remains constant because all valves are closed. 3. Ventricular Ejection (Mechanical Systole) Electrical Event: Ventricular depolarization continues; repolarization begins after the QRS complex. Mechanical Event: Once ventricular pressure exceeds the pressure in the aorta and pulmonary artery, the aortic and pulmonary valves open, and blood is ejected from the ventricles. This is the ventricular ejection phase. Ventricular Volume: Ventricular volume decreases as blood is pumped out. This volume is called Stroke Volume (SV), and what remains is the End-Systolic Volume (ESV). 4. Isovolumetric Relaxation (Electrical: T Wave) Electrical Event: Ventricular repolarization, corresponding to the T wave. Mechanical Event: As the ventricles relax, the pressure in the ventricles falls below the pressure in the aorta and pulmonary artery, causing the aortic and pulmonary valves to close (S2 heart sound, "dub"). No blood enters the ventricles, and all valves are closed. Ventricular Volume: Volume remains constant because all valves are closed. 5. Passive Ventricular Filling (Electrical: TP Interval) Electrical Event: No major electrical activity is seen during this phase (the TP interval on the EKG). Mechanical Event: The pressure in the atria exceeds that in the ventricles, causing the AV valves (tricuspid and mitral) to open. Blood flows passively from the atria into the ventricles, filling them. (no heart sounds) Ventricular Volume: Ventricular volume increases during this phase as the ventricles refill. The end-diastolic volume (EDV) is reached just before the next contraction. 15) What are the only times during the cardiac cycle that ventricular volume is not changing? The only times during the cardiac cycle when ventricular volume does not change are during the isovolumetric contraction and isovolumetric relaxation phases. 1) Isovolumetric Contraction When: Occurs after atrial contraction and after the AV valves close (marked by S1). What happens: The ventricles are contracting, and pressure is rising, but the aortic and pulmonary valves remain closed because the ventricular pressure hasn't yet surpassed that of the aorta and pulmonary artery. Constant volume: Since all valves are closed, no blood enters or leaves the ventricles, resulting in a constant ventricular volume. 2) Isovolumetric Relaxation When: Occurs after ventricular ejection and after the semilunar valves close (marked by S2). What happens: The ventricles are relaxing, and pressure is decreasing, but the AV valves remain closed because ventricular pressure is still higher than atrial pressure. Constant volume: With all valves closed, no blood moves in or out of the ventricles, maintaining a constant ventricular volume. Importance of These Phases: Pressure Build-up: Isovolumetric contraction allows the ventricles to build enough pressure to eventually open the semilunar valves and eject blood. Prevention of Backflow: Both phases ensure that blood doesn't flow back into the atria or the ventricles during pressure changes, maintaining the unidirectional flow of blood through the heart. 16) Describe all the electrical and mechanical events of the cardiac cycle that correspond in time with the QRS complex (and just after, 30-40 msec, the QRS complex) on the EKG? QRS represents ventricular depolarization (electrical signal that tells ventricles to contract) beginning of ventricular systole Left ventricular pressure increases exceeding the atria => AV valves shut First heart sound seen due to closure of both AV valves Isovolumetric Contraction: Ventricles are contracting and building up pressure but ALL the valves are still closed. Remember we are trying to increase ventricular pressure to exceed aortic pressure in order for the valves to open. 30-40msec After the QRS complex. Left ventricular pressure exceeds aortic pressure (marking the end of isovolumetric contraction) This opens the Semilunar valves (aortic /pulmonic) to eject blood into the Aorta 17) Describe the pressure differences during Isovolumetric contraction between: (a) The ventricles> and the atria – This pressure is greater than atrial pressure (Pressure forces AV valves to close) (b) The ventricles and the < arteries - Arterial pressure is higher than the ventricles (This is because the ventricles are still building up pressure to overcome the pressure in the arteries) 18) What is the required change that allows the ventricles to move from Isovolumetric contraction to the ejection phase? Ventricular pressure needs to goes above aortic pressure which results in the Aortic valve opening and ejection of the blood. 19) Think of the mechanical events that take place during the second heart sound and answer the following. The second heart sound (S2) occurs during the cardiac cycle when the ventricles are relaxing What is happening in terms of aortic vs. ventricular pressure? Aortic Pressure exceeds ventricular pressure. What is happening electrically in terms of the EKG? Middle (Peak) of the T Wave. Represents ventricular repolarization, the electrical recovery of the ventricles as they prepare for the next heartbeat What is happening in terms of the heart valves? Closure of the semilunar valves (aortic and pulmonic) These valves close to prevent the backflow of blood from the aorta and pulmonary artery back into the ventricles as the ventricles relax. The closure of the aortic valve creates a pressure wave that travels back up the aorta, causing a small bump in aortic pressure known as the dicrotic notch 20) If a patient had an alteration in his second heart sound (ex. the sound was muffled or involved an abnormal whistle), this would most likely indicate a problem with what structure in the heart? Aortic Valve– The closure of this valve is what makes the louder in the second sound because of the pressure 21) A patient with hypertension has a systolic blood pressure of 140mmHg and a diastolic blood pressure of 95mmHg. How high must left ventricular pressure reach in order to end isovolumetric contraction in this patient (give a number value)? 95mmHG. Point where Ventricular pressure exceeds Aortic resting pressure marks the end of isovolumetric contraction.