Complex Inheritance of Common Multifactorial Disorders PDF
Document Details
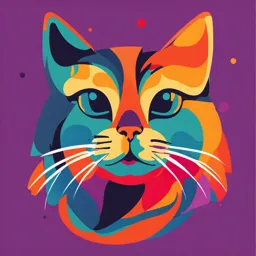
Uploaded by ProblemFreeVibrance4253
Tags
Summary
This document explores the complex inheritance of common, multifactorial disorders. It details how these diseases, like congenital birth defects and cancer, don't follow simple Mendelian patterns, instead arising from complex interactions between genetic variants, environmental factors, and chance. The document also examines different types of traits and familial aggregation.
Full Transcript
# Complex Inheritance of Common Multifactorial Disorders Common diseases such as congenital birth defects, myocardial infarction, cancer, neuropsychiatric disorders, diabetes, and Alzheimer disease cause morbidity and premature mortality in nearly two of every three individuals during their lifetim...
# Complex Inheritance of Common Multifactorial Disorders Common diseases such as congenital birth defects, myocardial infarction, cancer, neuropsychiatric disorders, diabetes, and Alzheimer disease cause morbidity and premature mortality in nearly two of every three individuals during their lifetimes. Many of these diseases "run in families" - cases seem to cluster among the relatives of affected individuals more frequently than in the general population. However, their inheritance generally does not follow one of the Mendelian patterns seen in the single-gene disorders described in Chapter 7. This is because such diseases rarely result simply from inheriting one or two alleles at a single locus, as is the case for dominant and recessive Mendelian disorders. Instead, they are thought to result from complex interactions among a number of genetic variants that alter susceptibility to disease, combined with certain environmental exposures and perhaps chance events as well, all of which acting together may trigger, accelerate, or protect against the disease process. For this reason, these disorders are considered to be multifactorial in origin, and the familial clustering generates a pattern of inheritance that is referred to as complex. The familial clustering and complex inheritance seen with multifactorial disorders can be explained by recognizing that family members share a greater proportion of their genetic information and environmental exposures than individuals chosen at random in the population. ## Qualitative and Quantitative Traits Multifactorial diseases with complex inheritance can be classified either as discrete qualitative traits or as continuous quantitative traits. A qualitative trait is the simpler of the two; a disease, such as lung cancer or rheumatoid arthritis, is either present or absent in an individual. Distinguishing between individuals who either have a disease or not is usually straightforward, but it may sometimes require detailed examination or specialized testing if the manifestations are subtle. In contrast, a quantitative trait is a measurable physiological or biochemical quantity, such as height, blood pressure, serum cholesterol concentration, or body mass index, that varies among different individuals within a population. Although a quantitative trait varies continuously across a range of values, there are certain disease diagnoses, such as short stature, hypertension, hypercholesterolemia, or obesity, that are defined based on whether the value of the trait falls outside the so-called normal range, defined as an arbitrary interval around the population average. ## Familial Aggregation and Correlation The more closely related two individuals are in a family, the more alleles they have in common, inherited from their common ancestors. The most extreme examples of two individuals having alleles in common are identical (monozygotic (MZ)) twins, who have the same alleles at every locus. The next most closely related individuals in a family are first-degree relatives, such as a parent and child or a pair of sibs, including fraternal (dizygotic (DZ)) twins. ### Allele Sharing Among Relatives In a parent-child pair, the child has exactly one allele out of two (50% of alleles) in common with each parent at every locus, that is, the allele the child inherited from that parent. Siblings (including DZ twins) also have 50% of their alleles in common with their other siblings, but this is only on average. This is because a pair of sibs inherits the same two alleles at a locus one fourth of the time, no alleles in common one fourth of the time, and one allele in common one half of the time. At any one locus, therefore, the average number of alleles an individual is expected to share with a sibling given by: ¼ (2 alleles) + ¼ (0 allele) + ½ (1 allele) = 0.5+0+0.5 = 1 allele The more distantly related two members of a family are, the fewer alleles they will have in common, inherited from a common ancestor. ### Familial Aggregation in Qualitative Traits If certain alleles increase the chance of developing a disease, one would expect an affected individual to have a greater-than-expected number of affected relatives compared to what would be predicted from the frequency of the disease in the general population (familial aggregation of disease). This is because the more closely related the family members are to the affected relative, the more they will share the relevant alleles and the greater their chance of also being affected. #### Relative Risk Ratio One way to measure familial aggregation of a disease is by comparing the frequency of the disease in the relatives of an affected proband with its frequency (prevalence) in the general population. The relative risk ratio, λr, (where the subscript "r" refers to relatives) is defined as: λr = Prevalence of the disease in the relatives of an affected person / Prevalence of the disease in the general population The value of λr, as a measure of familial aggregation depends both on how frequently a disease is found to have recurred in a relative of an affected individual (the numerator) and on the population prevalence (the denominator); the larger λr, is, the greater is the familial aggregation. The population prevalence enters into the calculation because the more common a disease is, the greater is the likelihood that aggregation may be just a coincidence based on drawing alleles from the overall gene pool rather than a result of sharing the alleles that predispose to disease because of familial inheritance. A value of λr = 1 indicates that a relative is no more likely to develop the disease than is any individual in the population, whereas a value greater than 1 indicates that a relative is more likely to develop the disease. #### Family History Case-Control Studies Another approach to assessing familial aggregation is the case-control study, in which patients with a disease (the cases) are compared with suitably chosen individuals without the disease (the controls), with respect to family history of disease (as well as other factors, such as environmental exposures, occupation, geographical location, parity, and previous illnesses). To assess a possible genetic contribution to familial aggregation of a disease, the frequency with which the disease is found in the extended families of the cases (positive family history) is compared with the frequency of positive family history among suitable controls, matched for age and ethnicity, but who do not have the disease. ### Familial Correlation The tendency for the values of a physiological measurement to be more similar among relatives than it is in the general population is measured by determining the degree of correlation of particular physiological quantities among relatives. The coefficient of correlation (symbolized by the letter r) is a statistical measure of correlation applied to a pair of measurements, such as, for example, a child’s serum cholesterol level and that of a parent. Accordingly, a positive correlation would exist between the cholesterol measurements in a group of patients and the cholesterol levels in their relatives, if it is found that the higher a patient's level, the proportionally higher is the level in the patient's relatives. ### Heritability The concept of heritability of a quantitative trait (symbolized as H²) was developed in an attempt to determine how much the genetic differences between individuals in a population contribute to variability of that trait in the population. H² is defined as the fraction of the total phenotypic variance of a quantitative trait that is due to allelic variation in the broadest sense, regardless of the mechanism by which the various alleles affect the phenotype. The higher the heritability, the greater is the contribution of genetic differences among people to the variability of the trait in the population. The value of H² varies from 0, if genotype contributes nothing to the total phenotypic variance in a population, to 1, if genotype is totally responsible for the phenotypic variance in that population. ## Examples of Common Multifactorial Diseases with a Genetic Contribution This section and the next, we turn to considering examples of several common conditions that illustrate general concepts of multifactorial disorders and their complex inheritance. ### Multifactorial Congenital Malformations Many common congenital malformations, occurring as isolated defects and not as part of a syndrome, are multifactorial and demonstrate complex inheritance. Among these, congenital heart malformations are some of the most common and serve to illustrate the current state of understanding of other categories of congenital malformation. Congenital heart defects (CHDs) occur at a frequency of approximately 4 to 8 per 1000 births. They are a heterogeneous group, caused in some cases by single-gene or chromosomal mechanisms and in others by exposure to teratogens, such as rubella infection or maternal diabetes. ### Neuropsychiatric Disorders Mental illnesses are some of the most common and perplexing of human diseases, affecting 4% of the human population worldwide. The annual cost in medical care and social services exceeds $150 billion in the United States alone. Among the most severe of the mental illnesses are schizophrenia and bipolar disease (manic-depressive illness). #### Schizophrenia Schizophrenia affects 1% of the world's population. It is a devastating psychiatric illness, with onset commonly in late adolescence or young adulthood, and is characterized by abnormalities in thought, emotion, and social relationships, often associated with delusional thinking and disordered mood. A genetic contribution to schizophrenia is supported by both twin and family aggregation studies. MZ concordance in schizophrenia is estimated to be 40% to 60%; DZ concordance is 10% to 16%. #### Bipolar Disease <start_of_image>FREQUENCY OF HLA ALLELES The human leukocyte antigen (HLA) system can be confusing at first because the nomenclature used to define and describe different HLA alleles has undergone a fundamental change with the advent of widespread DNA sequencing of the major histocompatibility complex (MHC). According to the older system of HI A nomenclature, the different alleles were distinguished from one another serologically. However, as the genes responsible for encoding the class I and class II MHC chains were identified and sequenced, single HLA alleles initially defined serologically were shown to consist of multiple alleles defined by different DNA sequence variants even within the same scrological allele. The 100 serological specificities at HLA-A, B, C, DR, DQ, and DP loci now comprise more than 1300 alleles defined at the DNA sequence level! ## Modifier Genes in Mendelian Disorders As discussed in Chapter 7, allelic variation at a single locus can explain variation in the phenotype in many single-gene disorders. However, even for well-characterized Mendelian disorders known to be due to defects in a single gene, variation at other gene loci may impact some aspect of the phenotype, illustrating features therefore of complex inheritance. ### Examples of Multifactorial Traits for Which Specific Genetic and Environmental Factors are Known Up to this point, we have described some of the epidemiological approaches involving family and twin studies that are used to assess the extent to which there may be a genetic contribution to a complex trait. It is important to realize, however, that studies of familial aggregation, disease concordance, or heritability do not specify how many loci there are, which loci and alleles are involved, or how a particular genotype and set of environmental influences interact to cause a disease or to determine the value of a particular physiological measurement. ### Digenic Inheritance The next level of complexity is a disorder determined by the additive effect of the genotypes at two or more loci. One clear example of such a disease phenotype has been found in a few families of patients with a form of retinal degeneration called retinitis pigmentosa (RP). Affected individuals in these families are heterozygous for mutant alleles at two different loci (double heterozygotes). One locus encodes the photoreceptor membrane protein peripherin and the other encodes a related photoreceptor membrane protein called Rom1. Heterozygotes for only one or the other of these mutations in these families are unaffected. Thus the RP in this family is caused by the simplest form of multigenic inheritance, inheritance due to the effect of mutant alleles at two loci, without any known environmental factors that influence disease occurrence or severity. ### Gene-Environment Interactions in Venous Thrombosis Another example of gene-gene interaction predisposing to disease is found in the group of conditions referred to as hypercoagulability states, in which venous or arterial clots form inappropriately and cause life-threatening complications of thrombophilia. With hypercoagulability, however, there is a third factor, an environmental influence that in the presence of the predisposing genetic factors, increases the risk for disease even more. ### Multiple Coding and Noncoding Elements in Hirschsprung Disease A more complicated set of interacting genetic factors has been described in the pathogenesis of a developmental abnormality of the enteric nervous system in the gut known as Hirschsprung disease (HSCR). In HSCR, there is complete absence of some or all of the intrinsic ganglion cells in the myenteric and submucosal plexuses of the colon. An aganglionic colon is incapable of peristalsis, resulting in severe constipation, symptoms of intestinal obstruction, and massive dilatation of the colon (megacolon) proximal to the aganglionic segment. #### Type 1 Diabetes Mellitus A common complex disease for which some of the underlying genetic architecture is being delineated is diabetes mellitus. Diabetes occurs in two major forms: type 1 (T1D) (sometimes referred to as insulin-dependent; IDDM) and type 2 (T2D) (sometimes referred to as non-insulin-dependent; NIDDM), representing approximately 10% and 88% of all cases, respectively. Familial aggregation is seen in both types of diabetes, but in any given family, usually only TID or T2D is present. They differ in typical onset age, MZ twin concordance, and association with particular genetic variants at particular loci. #### Alzheimer Disease Alzheimer disease (AD) is a fatal neurodegenerative disease that affects 1% to 2% of the United States population. It is the most common cause of dementia in older adults and is responsible for more than half of all cases of dementia. As with other dementias, patients experience a chronic, progressive loss of memory and other cognitive functions, associated with loss of certain types of cortical neurons. Age, sex, and family history are the most significant risk factors for AD. Once a person reaches 65 years of age, the risk for any dementia, and AD in particular, increases substantially with age and female sex. ## The Challenge of Multifactorial Disease With Complex Inheritance The greatest challenge facing medical genetics and genomic medicine going forward is unraveling the complex interactions between the variants at multiple loci and the relevant environmental factors that underlie the susceptibility to common multifactorial disease. This area of research is the central focus of the field of population-based genetic epidemiology (to be discussed more fully in Chapter 10). The field is developing rapidly, and it is clear that the genetic contribution to many more complex diseases in humans will be elucidated in the coming years. Such understanding will, in time, allow the development of novel preventive and therapeutic measures for the common disorders that cause such significant morbidity and mortality in the general population.