Chapter 3 Genetic Control of Protein Synthesis, Cell Function, and Cell Reproduction PDF
Document Details
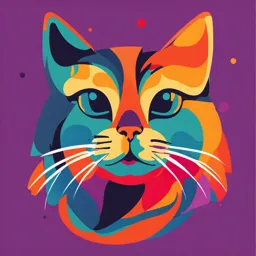
Uploaded by yvesss
Ayura 2027
Tags
Related
- Anthro 1 Spring 24 DNA PDF
- Guyton & Hall 14th ed 3.pdf Genetic Control of Protein Synthesis, Cell Function, and Cell Reproduction PDF
- كتاب الأحياء - الصف الثاني عشر - الفصل الدراسي الأول: الشيفرة الجينية PDF
- Genetic Control of Cell Function PDF
- Microbial Physiology (2002) by Moat, Foster, Spector - PDF
- RNA Processing - Cell Biology - PDF
Summary
This chapter from a biology textbook describes the process of protein synthesis in cells, explaining how genes control cell function and the role of RNA in the process. It includes a diagram illustrating the general schema of gene control.
Full Transcript
CHAPTER 3 Genetic Control of Protein Synthesis, Cell Function, and Cell Reproduction Genes in the Cell Nucleus Control Protein Synthesis (p. 27). The genes control protein synthesis in the cell and in this way control...
CHAPTER 3 Genetic Control of Protein Synthesis, Cell Function, and Cell Reproduction Genes in the Cell Nucleus Control Protein Synthesis (p. 27). The genes control protein synthesis in the cell and in this way control cell function. Proteins play a key role in almost all functions of the cell by serving as enzymes that catalyze the reactions of the cell and as major components of the physical structures of the cell. Each gene is a double-stranded, helical molecule of deoxyribonucleic acid (DNA) that controls formation "G of ribonucleic acid (RNA). The RNA, in turn, spreads throughout the cells to control the formation of a spe- cific protein. The entire process, from transcription of the genetic code in the nucleus to translation of the RNA code and formation of proteins in the cell cytoplasm, is often referred to as gene expression and is shown in Figure 3–1. Because there are about 30,000 genes in each cell, it is possible to form large numbers of different cel- lular proteins. In fact, RNA molecules transcribed from Plasma Nuclear membrane envelope Nucleus DNA Gene (DNA) DNA Transcription transcription RNA RNA formation RNA splicing Translation mRNA Ribosomes RNA transport Protein mRNA formation Translation of messenger RNA Cell Cell structure enzymes Protein Cytosol Cell function Figure 3–1 General schema by which the genes control cell function. 19 20 UNIT I Introduction to Physiology: The Cell and General Physiology the same gene can be processed in different ways by the cell, giving rise to alternate versions of the protein. The total number of different proteins produced by various cell types in humans is estimated to be at least 100,000. Nucleotides Are Organized to Form Two Strands of DNA Loosely Bound to Each Other. Genes are attached in an end- on-end manner in long, double-stranded, helical molecules of DNA that are composed of three basic building blocks: (1) phosphoric acid, (2) deoxyribose (a sugar), and (3) four nitrogenous bases: two purines (adenine and guanine) and two pyrimidines (thymine and cytosine). The first stage in DNA formation is the combina- tion of one molecule of phosphoric acid, one molecule of deoxyribose, and one of four bases to form a nucleo- tide. Four nucleotides can therefore be formed, one from each of the four bases. Multiple nucleotides are bound together to form two strands of DNA, and the two strands are loosely bound to each other. The backbone of each DNA strand is composed of alternating phosphoric acid and deoxyribose molecules. The purine and pyrimidine bases are attached to the side of the deoxyribose molecules, and loose bonds between the purine and pyrimidine bases of the two DNA strands hold them together. The purine base adenine of one strand always bonds with the pyrimidine base thymine of the other strand, whereas guanine always bonds with cytosine. The Genetic Code Consists of Triplets of Bases. Each group of three successive bases in the DNA strand is called a code word. These code words control the sequence of amino acids in the protein to be formed in the cytoplasm. One code word, for example, might be composed of a sequence of adenine, thymine, and guanine, whereas the next code word might have a sequence of cytosine, guanine, and thymine. These two code words have entirely different meanings because their bases are different. The sequence of successive code words of the DNA strand is known as the genetic code. THE DNA CODE IN THE NUCLEUS IS TRANSFERRED TO RNA CODE IN THE CELL CYTOPLASM—THE PROCESS OF TRANSCRIPTION (p. 30) Because DNA is located in the nucleus and many func- tions of the cell are carried out in the cytoplasm, there must be some method by which the genes of the nucleus control the chemical reactions of the cytoplasm. This is achieved through RNA, the formation of which is con- trolled by DNA. During this process the code of DNA is transferred to RNA, a process called transcription. Genetic Control of Protein Synthesis, Cell Function, and Cell 21 Reproduction The RNA diffuses from the nucleus to the nuclear pores into the cytoplasm, where it controls protein synthesis. RNA Is Synthesized in the Nucleus From a DNA Template. During synthesis of RNA, the two strands of the DNA molecule separate, and one of the two strands is used as a template for RNA synthesis. The code triplets in DNA cause the formation of complementary code triplets (called codons) in RNA; these codons then control the sequence of amino acids in a protein to be synthesized later in the cytoplasm. Each DNA strand in each chromosome carries the code for perhaps as many as 2000 to 4000 genes. The basic building blocks of RNA are almost the same as those of DNA except that in RNA, the sugar ribose replaces the sugar deoxyribose and the pyrimi- dine uracil replaces thymine. The basic building blocks of RNA combine to form four nucleotides, exactly as described for the synthesis of DNA. These nucleotides contain the bases adenine, guanine, cytosine, and uracil. The next step in the RNA synthesis is activation of the nucleotides, which occurs through the addition of two phosphate radicals to each nucleotide to form tri- phosphates. These last two phosphates are combined with the nucleotide by high-energy phosphate bonds, which are derived from the adenosine triphosphate (ATP) of the cell. This activation process makes avail- able large quantities of energy, which is used for pro- moting the chemical reactions that add each new RNA nucleotide to the end of the RNA chain. The DNA Strand Is Used as a Template to Assemble the RNA Molecule From Activated Nucleotides. The assembly of the RNA molecule occurs under the influence of the enzyme RNA polymerase as follows: 1. In the DNA strand immediately ahead of the gene that is to be transcribed is a sequence of nucleotides called the promoter. An RNA polymerase recognizes this promoter and attaches to it. 2. The polymerase causes unwinding of two turns of the DNA helix and separation of the unwound portions. 3. The polymerase moves along the DNA strand and begins forming the RNA molecules by binding com- plementary RNA nucleotides to the DNA strand. 4. The successive RNA nucleotides then bind to each other to form an RNA strand. 5. When the RNA polymerase reaches the end of the DNA gene, it encounters a sequence of DNA mole- cules called the chain-terminating sequence, caus- ing the polymerase to break away from the DNA strand. The RNA strand is then released into the nucleoplasm. 22 UNIT I Introduction to Physiology: The Cell and General Physiology The code present in the DNA strand is transmitted in complementary form to the RNA molecule as fol- lows: DNA Base RNA Base Guanine Cytosine Cytosine Guanine Adenine Uracil Thymine Adenine There Are Several Types of RNA. Research on RNA has uncovered many different types of RNA. Some are involved in protein synthesis, whereas others serve gene regulatory functions or are involved in posttranscriptional modification of RNA. The following six types of RNA play independent and different roles in protein synthesis: 1. Precursor messenger RNA (pre-mRNA), a large, im- mature single strand of RNA that is processed in the nucleus to form mature mRNA and includes two different types of segments called introns, which are removed by a process called splicing, and exons, which are retained in the final mRNA 2. Small nuclear RNA (snRNA), which directs the splicing of pre-mRNA to form mRNA 3. mRNA, which carries the genetic code to the cyto- plasm to control the formation of proteins 4. ribosomal RNA, which, along with proteins, forms the ribosomes, the structures in which protein mol- ecules are assembled 5. Transfer RNA (tRNA), which transports activated amino acids to the ribosomes to be used in the as- sembly of the proteins 6. microRNA (miRNA), which are single-stranded RNA molecules of 21 to 23 nucleotides that can regulate gene transcription and translation There are 20 types of tRNA, each of which combines specifically with one of the 20 amino acids and carries this amino acid to the ribosomes, where it is incorporated in the protein molecule. The code in the tRNA that allows it to recognize a specific codon is a triplet of nucleotide bases called an anticodon. During formation of the pro- tein molecule, the three anticodon bases combine loosely by hydrogen bonding with the codon bases of the mRNA. In this way, the various amino acids are lined up along the mRNA chain, thus establishing the proper sequence of amino acids in the protein molecule. Genetic Control of Protein Synthesis, Cell Function, and Cell 23 Reproduction TRANSLATION—SYNTHESIS OF POLYPEPTIDES ON RIBOSOMES FROM GENETIC CODE IN mRNA (p. 33) To manufacture proteins, one end of the mRNA strand enters the ribosome, and then the entire strand threads its way through the ribosome in just over a minute. As it passes through, the ribosome “reads” the genetic code and causes the proper succession of amino acids to bind together to form chemical bonds called peptide linkages. The mRNA does not recognize the different types of amino acids but, instead, recognizes the different types of tRNA. Each type of tRNA molecule carries only one specific type of amino acid that is incorporated into the protein. Thus, as the strand of mRNA passes through the ribo- some, each of its codons attracts to it a specific tRNA that, in turn, delivers a specific amino acid. This amino acid then combines with the preceding amino acids to form a peptide linkage, and this sequence continues to build until an entire protein molecule is formed. At this point, a chain-terminating (or “stop”) codon appears and indicates completion of the process, and the protein is released into the cytoplasm or through the membrane of the endoplasmic reticulum to the interior. CONTROL OF GENE FUNCTION AND BIOCHEMICAL ACTIVITY IN CELLS (p. 35) The genes control the function of each cell by determin- ing the relative proportion of various types of enzymes and structural proteins that are formed. Regulation of gene expression covers the entire process from tran- scription of the genetic code in the nucleus to the for- mation of proteins in the cytoplasm. The Promoter Controls Gene Expression. Cellular protein synthesis starts with transcription of DNA into RNA, a process controlled by regulatory elements in the promoter of a gene. In eukaryotes, including mammals, the basal promoter consists of a sequence of seven bases (TATAAAA) called the TATA box, which is the binding site for the TATA-binding protein and several other important transcription factors that are collectively referred to as the transcription factor IID complex. In addition to the transcription factor IID complex, this region is where transcription factor IIB binds to both the DNA and RNA polymerase 2 to facilitate transcription of the DNA into RNA. This basal promoter is found in all protein coding genes, 24 UNIT I Introduction to Physiology: The Cell and General Physiology and the polymerase must bind with this basal promoter before it can begin traveling along the DNA strand to synthesize RNA. The upstream promoter is located further upstream from the transcription start site and contains several binding sites for positive or negative transcription factors that can effect transcription through interactions with proteins bound to the basal promoter. The structure and transcription factor binding sites in the upstream promoter vary from gene to gene to give rise to the different expression patterns of genes in different tissues. Transcription of genes in eukaryotes is also influ- enced by enhancers, which are regions of DNA that can bind transcription factors. Enhancers can be located far from the gene they act on or even on a different chro- mosome. Although enhancers may be located a great distance away from their target gene, they may be rela- tively close when DNA is coiled in the nucleus. It is esti- mated that there are 110,000 gene enhancer sequences in the human genome. Control of the Promoter Through Negative Feedback by the Cell Product. When the cell produces a critical amount of substance, it causes negative feedback inhibition of the promoter that is responsible for its synthesis. This inhibition can be accomplished by causing a regulatory repressor protein to bind at the repressor operator or a regulatory activator protein to break this bond. In either case, the promoter becomes inhibited. Other mechanisms are available for control of tran- scription by the promoter, including the following: 1. A promoter may be controlled by transcription fac- tors located elsewhere in the genome. 2. In some instances, the same regulatory protein func- tions as an activator for one promoter and as a re- pressor for another, allowing different promoters to be controlled at the same time by the same regula- tory protein. 3. The nuclear DNA is packaged in specific structural units, the chromosomes. Within each chromosome, the DNA is wound around small proteins called his- tones, which are held together tightly in a compacted state with other proteins. As long as DNA is in this compacted state, it cannot function to form RNA. Multiple mechanisms exist, however, that can cause selected areas of the chromosomes to become de- compacted, allowing RNA transcription. Even then, specific transcription factors control the actual rate of transcription by the promoter in the chromo- some. Genetic Control of Protein Synthesis, Cell Function, and Cell 25 Reproduction THE DNA–GENETIC SYSTEM CONTROLS CELL REPRODUCTION (p. 37) The genes and their regulatory mechanisms determine not only the growth characteristics of cells but also when and whether these cells divide to form new cells. In this way, the genetic system controls each stage of the development of the human from the single-cell fertil- ized ovum to the whole functioning body. Most cells of the body, with the exception of mature red blood cells, striated muscle cells, and neurons, are capable of reproducing other cells of their own type. Ordinarily, as sufficient nutrients are available, each cell increases in size until it divides via mitosis to form two new cells. Different cells of the body have different life cycle periods that vary from as short as 10 hours for highly stimulated bone marrow cells to the entire life- time of the human body for nerve cells. Cell Reproduction Begins With Replication of DNA. Mitosis can take place only after all of the DNA in the chromosomes has been replicated. The DNA is duplicated only once, so the net result is two exact replicates of all DNA. These replicates then become the DNA of the two daughter cells that will be formed at mitosis. The replication of DNA is similar to the way RNA is transcribed from DNA, except for a few important differences: 1. Both strands of the DNA are replicated, not just one of them. 2. Both strands of the DNA helix are replicated from end to end rather than small portions of them, as oc- curs during the transcription of RNA by genes. 3. The principal enzymes for replication of DNA are a complex of several enzymes called DNA polymerase, which is comparable to RNA polymerase. 4. Each newly formed DNA strand remains attached by loose hydrogen bonding to the original DNA strand that is used as its template. Two DNA helixes that are formed, therefore, are duplicates of each other and are still coiled together. 5. The two new helixes become uncoiled by the action of enzymes that periodically cut each helix along its entire length, rotate each segment sufficiently to cause separation, and then resplice the helix. DNA Strands Are “Repaired” and “Proofread.” During the time between the replication of DNA and the beginning of mitosis, there is a period of “proofreading” and “repair” of the DNA strands. Whenever inappropriate DNA nucleotides have been matched up with the nucleotides 26 UNIT I Introduction to Physiology: The Cell and General Physiology of the original template strand, special enzymes cut out the defective areas and replace them with the appropriate complementary nucleotides. Because of proofreading and repair, the transcription process rarely makes a mistake. When a mistake is made, however, it is called a mutation. Entire Chromosomes Are Replicated. The DNA helixes of the nucleus are each packaged as a single chromosome. The human cell contains 46 chromosomes arranged in 23 pairs. In addition to the DNA in the chromosome, there is a large amount of protein composed mainly of histones, around which small segments of each DNA helix are coiled. During mitosis, the successive coils are packed against each other, allowing the long DNA molecule to be packaged in a coiled and folded arrangement. Replication of the chromosomes in their entirety occurs soon after replication of the DNA helixes. The two newly formed chromosomes remain temporarily attached to each other at a point called the centromere, which is located near their center. These duplicated but still-attached chromosomes are called chromatids. Mitosis Is the Process by Which the Cell Splits Into Two New Daughter Cells. Two pairs of centrioles, which are small structures that lie close to one pole of the nucleus, begin to move apart from each other. This movement is caused by successive polymerization of protein microtubules growing outward from each pair of centrioles. As the tubules grow, they push one pair of centrioles toward one pole of the cell and the other toward the opposite pole. At the same time, other microtubules grow radially away from each of the centriole pairs, forming a spiny star called the aster at each end of the cell. The complex of microtubules extending between the centriole pairs is called the spindle, and the entire set of microtubules plus the pairs of centrioles is called the mitotic apparatus. Mitosis then proceeds through several phases. Prophase is the beginning of mitosis. While the spindle is forming, the chromosomes of the nucleus become condensed into well-defined chromosomes. Prometaphase is the stage at which the growing mi- crotubular spines of the aster puncture and fragment the nuclear envelope. At the same time, the microtu- bules from the aster become attached to the chroma- tids at the centromere, where the paired chromatids are still bound to each other. Metaphase is the stage at which the two asters of the mitotic apparatus are pushed farther and farther Genetic Control of Protein Synthesis, Cell Function, and Cell 27 Reproduction apart by additional growth of the mitotic spindle. Simultaneously, the chromatids are pulled tightly by the attached microtubules to the center of the cell, lining up to form the equatorial plate of the mitotic spindle. Anaphase is the stage at which the two chromatids of each chromosome are pulled apart at the centro- mere. Thus all 46 pairs of chromosomes are separat- ed, forming two sets of 46 daughter chromosomes. Telophase is the stage at which the two sets of daugh- ter chromosomes are pulled completely apart. Then the mitotic apparatus dissolves, and a new nuclear membrane develops around each set of chromo- somes. Cell Differentiation Allows Different Cells of the Body to Perform Different Functions. As a human develops from a fertilized ovum, the ovum divides repeatedly until trillions of cells are formed. The new cells gradually differentiate from each other, with certain cells having different genetic characteristics from other cells. This differentiation process occurs as a result of inactivation of certain genes and activation of others during successive stages of cell division. This process of differentiation leads to the ability of different cells in the body to perform different functions.