Physio C17 - Cerebellum PDF
Document Details
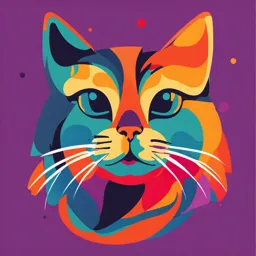
Uploaded by SplendidNovaculite8819
Università degli Studi di Milano Bicocca
Tags
Summary
This document covers the functions of the cerebellum. It details the structure and explains different roles of the cerebellum, including movement, posture, balance, and motor tasks like playing an instrument or riding a bicycle.
Full Transcript
PHYSIO C17 – Cerebellum Movement is produced by complex interactions of the basal nuclei, the cerebellum, and the cerebral cortex. In order to carry out its function, the cerebellum needs to know how the head, trunk, and limbs are oriented at all times. It also needs to know if they are stationary...
PHYSIO C17 – Cerebellum Movement is produced by complex interactions of the basal nuclei, the cerebellum, and the cerebral cortex. In order to carry out its function, the cerebellum needs to know how the head, trunk, and limbs are oriented at all times. It also needs to know if they are stationary (and in what position) or if they are moving (how fast, in which direction, etc.). Since the cerebellum is part of the motor system, it needs to be in formed about the status of each body part with reference to movement at all times so that it can implement its task. The specialized receptors, muscle spindles, and Golgi tendon organs (GTOs), unique to muscles and tendons, respectively, collect and send sensory information about the position and movement of the body’s parts to the cerebellum via the ascending sensory pathways to the cerebellum (which are the dorsal, ventral, and rostral spinocerebellar tracts and the cuneocerebellar tract). 1. Overview of sensory inputs The cerebellum plays major roles in timing of motor activities and in rapid, smooth progression from one muscle movement to the next. It also helps control the intensity of muscle contraction when the muscle load changes and controls the necessary instantaneous inter- play between agonist and antagonist muscle groups. The analysis of the functions of the cerebellum is guided by studies on consequences of lesions (pathology) and anatomical studies. The cerebellum is the conductor behind a specific movement, since it not only determines its speed, but with the aid of projections from the visual and other systems, it also orchestrates its course. The cerebellum has important functions in proprioception, muscle tone, maintenance of posture, balance (equilibrium), and coordination of skilled voluntary movements, as well as in the learning and memory of motor tasks, such as playing a musical instrument and riding a bicycle. All of these functions are performed at the subconscious level. The cerebellum has a constant organization, differently from the telencephalon. Its functions are therefore related to it connective architecture (input and output relationships). The cerebellum is very rich in sensory inputs: somatosensory and special senses - auditory, vestibular, visual etc. It operates at a subconscious level: a lesion of the cerebellum doesn’t compromise the ability to be conscious of the sensory experience (held in the cortex of the telencephalon). What is lost in case of a lesion of the cerebellum (in any region) is speed, accuracy, fluency, coordination, skill (dexterity): The cerebellum is a controller of the precision of the functions. When performing a movement/action, a motor program is needed. The motor program involves a huge portion of the cortex - the parietal and frontal cortex, sensory motor integration and is executed by corticospinal tract by means of 2 ways: - the descending fibers to the spinal cord - the interaction between different cortical areas for integration (to compute the program). The area of the brain essential for the execution of voluntary movement is the primary motor cortex (area 4 of Brodmann, precentral cortex). By lesioning some regions of the precentral cortex, there is a loss of voluntary movement with a poor possibility to recover. There is a huge interaction of the other cortical areas in telling the primary motor cortex what to perform on the spinal cord machinery: the other cortical areas assigned to send autonomous independent contribution action on the spinal cord, talk together and shape the action of the primary motor cortex. The primary motor cortex goes down with the main motor program ,which is the result of the interactions among all the other parts towards N1; this is in continuous exchange with the primary sensory cortex and every part is contributing to the voluntary movement by means of their independent ways to connect to the spinal cord. The motor program, which is something very complex at the level of the cortex, implies not only movement of the prime movers but also organization of the posture, prediction of the relevant sensory contribution and modulation of the sensory income to guide and monitor the ongoing movement. The cerebellum talks with the cortex when it’s time to program a movement, it is interacting with the motor cortex in the definition of the motor program: the cerebellum tells the cortex what feedbacks it expects to have (the time course of the feedback is already programmed, thanks to learning after mistakes made); it compares the rich sensory input generated by movement with that predicted from the internal commands generated to produce movement. The cerebellum firstly defines the program, then, during the movement, monitors by means of the sensory income the actual feedback. Lastly, it compares the feedback that is expected for the optimal execution of the movement with what actually goes out. If we’re doing something that is incorrect, it will stop the action and correct it at the level of the cortex or, in some cases, even at the level of the brainstem. It is a real-time control, hence why speed, accuracy, fluency and coordination are lost in case of lesion: it should prevent the error or at least correct it immediately. Differences result in error signals that allow very fast real time on-line feedback to correct movements: the consequence of cerebellar lesion in uncorrected errors of movement. With a lesion of the cerebellum it is not possible/very impaired to learn new movements, skills: the cerebellum is the depository of the computational analysis to be transferred to the cortex to have the expectation. Hence why, when there is a cerebellar lesion, the ability to learn is impaired: the online monitor or the peak forward controller is lost. The cerebellum makes a comparison between what is expected and what is actually ongoing. This analysis can be applied not only to movements but also to other functions of the cortex (e.g., cognitive functions). Learning and errors: some movements are very fast and feedback arrives too late to influence the outcome: the feedback however can still be used to update the feed-forward models used to generate subsequent movements. This underlines the more general role of the cerebellum in motor learning, possibly including some of the more cognitive aspects in the acquisition of motor skill. The integrative function of the cerebellum is still unknown. 2. Layers of the cortex 3 layers in cerebellar cortex: molecular, purkinje and granule cell layer. - Granule and golgi cells are located deep in the cortex (the one bordering the white matter); - bodies of the purkinje cells in mid layer; - the superficial layer contains stellate and basket cells(and fibers of the dendritic trees of the purkinje cells). The purkinje cells are positioned orthogonally with respect to the longitudinal axis. This is repeated for every folium throughout the whole cortex of the cerebellum. The white matter underneath contains the axons of the purkinje cells (out of cortex, efferents) and the axons of all the afferents reaching the cortex. In the image, are detectable the granule cells (in red) and the Golgi cells (in black) in the deep granule or input layer, the Purkinje cell bodies (in blue) in the middle or output layer and the stellate and basket cells (in black) in the superficial molecular or processing layer (together with the parallel fibres from the granule cells). The output from the functional unit is from a deep nuclear cell. This cell is continually under both excitatory and inhibitory influences. The excitatory influences arise from direct connections with afferent fibers that enter the cerebellum from the brain or the periphery. The inhibitory influence arises entirely from the Purkinje cell in the cortex of the cerebellum. The afferent inputs to the cerebellum are mainly of two types, one called the - climbing fiber - mossy fiber. The climbing fibers all originate from the inferior olives of the medulla. There is one climbing fiber for about 5 to 10 Purkinje cells. After sending branches to several deep nuclear cells, the climbing fiber continues all the way to the outer layers of the cerebellar cortex, where it makes about 300 synapses with the soma and dendrites of each Purkinje cell. This climbing fiber is distinguished by the fact that a single impulse in it will always cause a single, prolonged (up to 1 second), peculiar type of action potential in each Pur- kinje cell with which it connects, beginning with a strong spike and followed by a trail of weakening secondary spikes. This action potential is called the complex spike. The mossy fibers are all the other fibers that enter the cerebellum from multiple sources: the higher brain, brain stem, and spinal cord. These fibers also send collaterals to excite the deep nuclear cells. They then proceed to the granule cell layer of the cortex, where they also synapse with hundreds to thousands of granule cells. In turn, the granule cells send extremely small axons, less than 1 micrometer in diameter, up to the molecular layer on the outer surface of the cerebel- lar cortex. Here the axons divide into two branches that extend 1 to 2 millimeters in each direction paral- lel to the folia. Many millions of these parallel nerve fibers exist because there are some 500 to 1000 granule cells for every 1 Purkinje cell. It is into this molecular layer that the dendrites of the Purkinje cells project and 80,000 to 200,000 of the parallel fibers synapse with each Purkinje cell. The mossy fiber input to the Purkinje cell is quite different from the climbing fiber input because the synaptic connections are weak, so large numbers of mossy fibers must be stimulated simultaneously to excite the Purkinje cell. Furthermore, activation usually takes the form of a much weaker, short-duration Purkinje cell action potential called a simple spike, rather than the prolonged complex action potential caused by climbing fiber input. The purkinje cells respond to this input with an axon going out of the cortex and ending in the deep cerebellar nuclei. The only exception is the flocculonodular lobe: in this case the Purkinje cell fibers go outside the cerebellum (without any intermediate station) straight to vestibular nuclei. The vast majority of the computational analysis of the cerebellar cortex exits the cortex and ends in the deep nuclei of the cerebellum: the real output of cerebellum are the deep nuclei (the Purkinje cells are the output of the cerebellar cortex only). Direct stimulation of the deep nuclear cells by both the climbing and the mossy fibers excites them. By contrast, signals arriving from the Purkinje cells inhibit them. Normally, the balance between these two effects is slightly in favor of excitation so that under quiet conditions, output from the deep nuclear cell re- mains relatively constant at a moderate level of continu- ous stimulation. Information analysis The raw information coming from the input is working on the deep cerebellar nuclei exciting the cerebellar cortical circuitry, which in turn will end up on the output, modulating its excitation. This is where the comparison between the raw incoming information and the computation the cortex has done (that results into the modulation of the activity of the output) happens. The deep cerebellar nucleus receives the raw data and the command coming from the computation analysis of the cortex. This analysis corrects mismatches or modulates the activity of the deep cerebellar nuclei that will go to the final target. By knowing the origin of the information (spinal cord, brainstem, cortex) it can be found that this information is given in a raw form to the deep nuclei, the cerebellum verifies that everything matches and if it finds any mismatch it acts on the deep nuclei which in tunr will act on the target in order to correct the mistake. The cortical portion of the cerebellum has a memory of correct and incorrect actions. Indeed, in the cerebellar cortex there is a great expression of the long term potentiation, the synaptic changes that happen. The circuit in the long term is modified. The system projecting to the cerebellum is expecting a confirmation, and indeed this is the cerebellum function. Switching on the device, it is very interesting to see the contribution also of the other cells. The relationship between the granule cells and the mossy fibres: the mossy fibers (in red) enter and get in touch with multiple granule cells (it is a divergent system) the mossy fibers enter, bifurcates parallel to the longitudinal axis and synapse with the series of granule cells via varicosities along the axon of the mossy fibre (the synapse is not the conventional one with the axon branching at the end but it presents varicosities throughout the whole length of the axon performing the synaptic contact). This system of varicosities along the axon length is very effective in long distances. It is also seen in vegetative system, especially when an excitation of multiple tiny muscle fibres is needed.. The mossy fibres excite the granule cells: each mossy fiber contacts hundreds of granule cells (divergence in input). In this type of synapse different cells are involved: the mossy fibres, which contact the granule cells’ dendrites the granule cells the Golgi cells (in yellow), which act on the dendrites of the granule cells as well. Glomerulus The granule cell is receiving an excitatory input from the mossy fibre and another inhibitory input from the Golgi fibers. The whole structure together is called glomerulus and comprises - the dendrites of the granule cell (receiving neuron), - the mossy fiber axon - axon from the Golgi cell. Each granule cell receives 4/5 mossy fibre input via the glomerulus, meaning that more than one varicosity is present and they are very close to each other. These inputs come from the inferior and middle cerebellar peduncles and bring sensory inputs to the cerebellar cortex. Inputs The other entering fibres (afferent) are the climbing fibers: they come from the inferior olivary nucleus (that also gives collaterals to the deep nucleus of the cerebellum). The synaptic input in this case is not divergent: 1 climbing fiber contacts maximum 10 Purkinje cells (local activation). The climbing fibres have an advantage with respect to the mossy fibres: the mossy fibres act on the granule cells which in turn are acting very far in the dendritic tree of the Purkinje cell (located far from the body and therefore far from the axon hillock). Considering the decremental conduction of the postsynaptic potential, it is possible to understand that the contribution of the parallel fibres is committed exactly to a decremental conduction, since it travels a long way to reach the axon hillock. On the other side, the climbing fibres contact the dendrite of the Purkinje cell near its body, closer to the axon hillock (the dispersion of the current is therefore lower). The synaptic input is huge, made by a lot of spines, convergent and local: it constitutes an assurance that the input will be successful on the Purkinje cells. Looking at the architecture on the image, different elements can be detected: the mossy fibers - enter the cortex the glomerulus the parallel fibres - contact many Purkinje cells Purkinje cells granule cells - contact the Purkinje cells directly climbing fibers In the glomerulus, the granule cell dendrite receives from the mossy fibre (the membrane of the Golgi cell is embracing the varicosities of the mossy fibre) and acts on the Purkinje cells. The Golgi axon is acting (through an inhibition input) on the granule cell dendrite. The Golgi cell axon, running up to the molecular layer, is excited in turn by the parallel fibres. These events form an inhibitory loop. The aim of the inhibitory loop can be considered as a “refresh of the screen”. For instance, taking in consideration the fast proprioceptive information carried by the mossy fibres, the granule cell is continuously excited by the action potentials running through these fibres (they enter from the spinocerebellar tracts into the cerebellum). This system permits not to have a tonic excitation of the granule cells: as soon as the granule cells fire, the impulse will surely reach the Purkinje cells but the granule cells have to be “refreshed back” to membrane potential in order to allow the next stimulus in. Indeed, by continuously activating the granule cell without refreshing it there will be a saturation of the firing of the cell which won’t allow it to sense the successive stimulus. The granule cell must be “refreshed” in order to be sensitive to the later incoming stimulus. This constitutes a sort of autogenic inhibition and it happens for any type of information incoming. Spikes There is another feature that distinguishes between the mossy and climbing fibres: not only they differ for their position and organization, but they also have a different result. The parallel fibres input to the Purkinje cells is very convergent: each Purkinje cell receives inputs from 200,000 - 1 million parallel fibres. Indeed, the molecular layer is full of “wires” running longitudinally. This is a fast line: looking at the firing rate, it performs an excitatory action of up to 100 Hz, meaning that the fibres are talking very fast as a high frequency line of transmission (simple spikes). The input of the climbing fibers is very selective: each purkinje cell receives input from only one climbing fibre; the one fibre makes multiple synaptic contacts all over the Purkinje cell dendritic tree. The climbing fibre input is also excitatory, but has a very low frequency (~ 1-3Hz). Looking at the power of the synapse, it presents a peculiar reaction: it produces a profound Ca++ mediated depolarisation of the membrane and high frequency discharge ‘complex spikes’. the spika indicates a depolarization followed by a fibrillation and eventually it calms down: it is a sort of a burst. The climbing fibres come from the olivary nucleus, they are very powerful and act locally, they “talk slowly but with a loud volume” The mossy fibers arrive and convey their input. They are firing very fast, which makes sense with the frequency of discharge of the parallel fibers. There is an interaction with the deep nuclei. The climbing fibers originate from the inferior olivary nucleus. It is very powerful and local. It discharges at a low but powerful rate. Combining them together, the mossy fibers will switch on and off with the Purkinje cells at a high volume and the climbing will be more refined when “talking” to the Purkinje cells. Many Purkinje cells will receive inputs from the parallel fibers but only two Purkinje cells will receive the climbing fibers (hence why they are local and precise). 3. Synchronization of complex spiles in Purkinje cells. Experiment: when studying a neuron, it is necessary to understand its architecture and then its activity. It is then necessary to correlate the activity of the neuron with a significant parameter correlated with the discharge. This will allow the discharge to serve a function. After placing electrodes at the olivary nucleus of the rat, the rat performs the action of licking. The results are that as the rat is licking some cells show synchronous activation at one time (1) and during the action (2). Other cells do not activate during synchronous activation. This shows that the olivary nucleus does not follow the movement (velocity, onset, offset, …). It is just active during the movement (like in basal ganglia). It is therefore not possible to separate and localize cells depending on the parameter of the movement. It I sonly possible to define cells that are active and inactive during synchronous movement. Looking at how these cells are sensitive to peripheral afferents, they are not. This is due to the fact that the frequency of discharge of Purkinje cells is of 1-3 Hz. It seems that the during the movement, the inferior olivary nucleus is “talking” to many groups of Purkinje cells that are “interested” by this movement. It is “waking them up”. It acts as a power supply to increase the excitability of the group of Purkinje cells, that then will be able to control the movement. The inferior olivary nucleus modulates the responsiveness of the target, the Purkinje cells, in respect to their response to parallel fibers. The “waking up” of a certain group of cells is essential for the refinement of output for the movement. The non-urgency of the signal compared to the 4. Efferent output from cerebellum The Purkinje cell cortical outputs result from: - The interaction with afferent fibers - The interaction with interneurons - The interaction with other Purkinje cells - Membrane properties The deep nuclei output results from: - The interaction with afferent fibers - The interaction with average cortical outputs via Purkinje cells - Membrane properties The stellate and basket cells are very refined interneurons. They interact with Purkinje cells. They allow the output to be more precise. In the image above on the right Spinocerebrallar and other pre-cerebellar nuclei income in the cerebellum with mossy fibers. The granule cells give parallel fibers. They are actor on many cells, they are diverging. The inferior olive is divided in two shows the local, very precise and non-diverging effect. The Purkinje cells, blue and green, receive from the respective climbing fibers. The circuit is divergent not only on the longitudinal axis but also in the orthogonal axis. The mossy fibers enter and are able to excite different rows of cells. The red line present are the cells that receive the most inputs. The gray and light gray receive partial inputs. All the rows are excited with different degrees of intensity. This allows the input to diverge in rows but also to enlarge it. This causes a problem due to the somatotopic organisation of the cerebellum. The divergence and enlargement of the signal causes it to be blurred, which impeads the formation of a sharp response. The stellate and basket cells: the basket cells are located with their body on the row with the most excited body. The dendrites of the basket cells are in the “red” row. There are rows of basket cells that follow the corresponding excited row. This causes the basket cells to be parallelly excited with the Purkinje cells. The excitation of the basket cells will have an inhibitory effect on the neighbouring rows. This is a lateral inhibition. The red line will therefore emerge even more, in addition to the higher volume of inputs thanks to the refined job of the basket cells. The inputs mossy + climbing fibres) are very strictly organised to target specific Purkinje cells outputs. They are organised in cortical microzones. Simple cell activity occurs at a high frequency and encodes movement parameters, such as force and direction Climbing fibre activity (complex spikes) occurs at low frequency (~ 1 Hz) and appear to signal events such as errors Here the mossy fiber arribes at the granule cell and also gives off collaterals to the deep cerebellar neurons. The granule cells activates the parallel fibers. The parallel fibers excite the Golgi cells which inhibits the granule cell. It also excites the Purkinje cells and the basket cells. The basket inhibits the neighboring Purkinje cells. This allows the refinement of the input. The Purkinje cells acts as an inhibitor on the deep cerebellar neurons. The deep cerebellar neurons receives from the mossy fibers (collaterals) and the inferior olivary nucleus. The deep cerebellar nucleus then inhibits the inferior olivary nucleus (a loop is formed). The activity of the inferior olive is modulated on the basis of the errors occurring in the system. Further focusing of the input-output is achieved by basket cells inhibiting ‘off- beam’ Purkinje cells. Parallel fibres may co-ordinate activity in groups of Purkinje cells for complex acts such as walking. 5. Cerebellar functional regions are their projections to deep nuclei. The organization of the cerebellum under the form of a computational model translates on the basis of the analysis of the inputs arriving and the outputs departing (what’s the function of this system?). Who is receiving? The 3 deep nuclei in cerebellum are the fastigial, interpositus (composed of 2 main parts) and dentate nuclei. The cerebellum is subdivided in zones based on the anatomical features (anterior lobe, posterior lobe, flocculonodular lobe), the evolutionary division (archeocerebellum, paleocerebellum, neocerebellum) or the functional division (cerebrocerebellum, spinocerebellum and vestibulocerebellum). They are superimposable. The cerebellum is divided in - the flocculonodular lobe, - the medial zone (the vermis and the paravermis), - the intermediate zone (proximal to the vermis) - the lateral zone. There are 3 nuclei for 4 lobes. Parallelly, looking at the connections from the cortex of the cerebellum to the deep nuclei, it is possible to see that each part of the cortex has a reference nucleus. - lateral zone connects with the dentate nucleus, - intermediate is with the interpositus nuclei, - medial zone connects with the fastigial nuclei - floccularnodular zone doesn’t have a deep nucleus. The vermis receives from different types of information: - the vestibular nuclei - acoustic information - visual information - somatosensory information from the trunk and proximal muscles ( axial and proximal muscles). It processes the information through the fastidious nucleus and projects to the brainstem (vestibular nuclei, …) and to interneurons of the spinal cord. It is rich in the spinal cord. The medial portion of the intermediate zone receives from: - somatosensory receptors of the limb (distal proprioception) - the motor cortex The information is processes through the interpositus and projects towards the red nucleus of the brainstem and neurons of lamina 8. It a satellite of the corticospinal tracts. The lateral portion receives from the associative cortex (cortex that develops a preference for secondary sensory areas computation of the higher cognitive functions). The associative cortex is formed of the prefrontal cortex, the posterior parietal and the temporal lobe. These areas are dedicated to the computational analysis of different sources of sensory stimuli and the emergence of higher order functions, such as executive functions (prefrontal lobe – the ability to decide what to do and the desire to do something else ; the Stroop test assesses these functions: the patient reads a word referring to a color (blue), but the ink of the word is in a color different than blue. The patient is asked to name the ink, not the word). The prefrontal lobe also allows us to be socially adequate (patients aren’t able to inhibit instinctive stimuli). It allows the passage from the homunculus representation of the body by the brain to the one resembling reality. This part of the cerebellum receives from the associative cortex and controls the CST, the premotor, motor and parietal cortices. It does not receive sensory inputs but is an evolution of the cerebellum and corresponds to the neocortex. It is the most evolutionarily developed regions compared to the macaque. The information is processed by the dentate nuclei. The cerebellum is not only a way to control the movement, the ability to use sensory inputs to move are the source of the cognitive life. Association cortexes are based on sensory inputs, causing poor cognitive functions. Looking a cerebello-lesioned patients, cognitive issues arise depending on the location of the lesion. 6. Flocculonodular zone - vestibulocerebellum It is the most ancient portion. It does not have a nucleus: the Purkinje cells exit directly to the vestibular nuclei. It is an independent structure: the information enters with no intermediate step and exits with no intermediate step. The flocculonodular node received form the vestibule, vestibular nuclei and visual afferents (superior colliculus and striate cortex). This node is able to interact with the vestibulospinal tracts as it is a satellite of them. It acts on the medial and lateral vestibulospinal tracts. It is able to control this system. The vestibulospinal tract is able to control head and neck movements, ocular movements (lesion of the cerebellum can alter the VOR or nystagmus), epiaxial muscle movement (for posture) and proximal limb muscle movement. The vestibulocerebellum controls the balance between stance and gait. The integration of vestibular inputs with visual inputs allows this cerebellar region to control ocular movements and coordinated head-ocular movements. 7. Spinocerebellar (vermis) It receives afferent inputs from the cuneo-cerebellar tract (proporioception C7-above districts ; head and neck), the labyrinth, auditory inputs and visual inputs. It outputs to the fastigial nucleus and then to the spinal cord, the reticular formation (reticulo-spinal), the vestibular nuclei, the VL thalamic nucleus and the motor cortex. The vermis, given its action of the vestibular nuclei and the motor cortex, controls mainly the head and neck movements and the proximal limb muscles. It is similar to the vestibulocerebellum, but the vestibulocerebellar tracts coordinates the posture during locomotion and stance, whereas the spinocerebellar/vermis (here) controls posture during voluntary movement. The output of these two tracts is different, not only on the vestibular nuclei but also on the motor cortex, which goes into the VL thalamic nuclei. Due to these connections, it is part of the motor program since postural adjustments are necessary during movement. It is needed to integrate the program with the posture. 8. Spinocerebellum – intermediate pars of the hemispheres Once the body is set for motion, the motion itself has to be controlled at the distal limbs by the spinocerebellum at the level of the intermediate pars. It receives afferent inputs form the spinocerebellar tract (proprioception C8-below), as the limbs inform on the gravity forces, the acceleration, the posture during movement, and the primary motor cortex, the executive part of the CST. It will output towards the interpositus nucleus and then the red nucleus (pars magnocellularis, VL thalamic nuclei and the motor cortex. It allows the control of distal movement. The spinocerebellum control the: - Posture during movement - The mouth-head-neck movement - Distal fine movement execution (movement itself). It is somatotopically organized. 9. Cerebrocerebellum It receives afferent inputs from the posterior parietal cortex, the motor cortex and the premotor cortex all via the pontine nuclei. It will output towards the dentate nucleus and then, through the pontine nuclei, towards the red nucleus (pars parvicellularis, the second part of the red nucleus that doesn’t interact with the spinal cord but with the cortex for the program of movement), the VL thalamic nuclei and the premotor cortex. Recieves and talks the cortex: corticocortico loop to the cerebellum. It controls cortical motor programs (the timing, planning, sequence of motion, …) and motor learning motor (writes the programs). It is associated with the associative areas and is therefore involved in congnition, as the sequence is not only a sequence of actions, but computation of a neurological function. 10. Peduncles in cerebellar connections 11. The gain of sensory processing in the cerebellum depends on the motor task The image below is a functional MRI recording in the cerebellum with respect to a controlled condition (movement) the areas receiving more oxygen (more activated) with particular tasks. In this case, one task touches passively with tip of the fingers. The other task asks to recognize an object in a socket. In both cases the tip of the fingers is stimulated. Looking at the cerebellum, a difference of activation occurs when a sensory discrimination is asked. During sensory discrimination, there is a lateral activation. It is usually rare to see the cerebellum off. 12. Motor learning Wearing lenses that impair the visual field and the patient is asked to point on a precise line. Physiologically, the patient will have a certain minimal degree of error. When a patient with a lens, the field is deviated: initially, the patient will point completely off, but in seconds, it will be corrected. Taking the lenses off, the compensation will still be on, causing another compensation to occur to return to physiological conditions. A patient with a lesion to cerebellum will never be able to compensate and learn (because you can’t learn). 13. Neurological deficits The symptoms and signs to a cerebellar lesion are - Ataxia (lack of coordination): it can be applied to o gait, o dysmetria (error in movement amplitude – cannot reach the target). The activity of the muscle is w/o the correct speed to reach the target o dysdiadochokinesia (errors in executing rapid alternating movements): does not happen with someone with spinocerebellar problems o movement decomposition (disrupted temporal sequence of movements composing an action): timing of the action is impaired causing a decomposition of the movement o dysartia (slow and disarticulated speech) Ataxia can take different names depending of the impaired motor function. - Hypotonia (diminished resistance to passive limb displacement): - Pendolar reflexes: lack of control of agonist/antagonist activation – the stretch of agonist or antagonist muscle go beyond their time. - Action-intention tremor: series of erroneous correction in the range movement due to the failure of adaptive control. It is different Parkinson’s disease because PD causes tremor at rest. The tremor only occurs during movement. Vesibulocerebellar lesions are characterised by a: - Lack of balance - Gait ataxia - Falling down Spinocerebellar lesions are dependent on whether they are in the vermis or the paravermis. They are characterised by: - Hypotonia - Dysmetria - Action tremor - Speech deficits - Pendolar reflexes Cerebrocerebellar lesions are characterised by: - Decomposition of movement - Impaired reaction times - Impaired direction of movement A cooling dentate nucleus causes an impaired motor program, resulting in an inactivation of the movement. Usually there is an activation of the biceps and triceps during a reflex. When there is an impairment with the dentate nucleus, the movement goes beyond the normal time (see in blue). This cooling does not occur in voluntary nucleus. 14.Non-motor functions of the cerebellum The outputs of the cerebellar loops provide this structure with the anatomical substrate to influence the control of movement and also cognition. Neuroimaging and neurophysiological data supply compelling support for this view. The range of tasks associated with cerebellar activation is remarkable and includes tasks designed to assess attention, executive control, language, working memory, learning, pain, emotion and addiction. 15. Clinical point - Freidrich ataxia Freideich ataxia is named after the german doctor nikolaus friedreich who described the disorder in 1863. FRDA is a genetic conditions that damages nerve tissue in the area of the brain responsible for the coordination, muscle movement and some sensory functions (cerebellum and spinal cord). Uncoordinated movement (ataxia) worsen over time. Usually the conditions worsens begins between the ages of 8-15, but it can occasionally begin at birth. FRDA affects the SN involved in the muscle control and coordination but may also affect heart function. The age of appearance of the symptoms usually varies but is usually before 25. They may appear as early as 2 and as late as 30-40 years. The genetic pattern determines the severity of the condition. Initial weakness and unsteadiness of the legs results in difficulties in standing and waling. An affected child might trip requently over low obstacles. The difficulty of walking is usually followed by uncoordinated movements (ataxia) of the arms and hands causing difficulties in writing and other manipulative tasks. Other symptoms that appear early are loss of knee and ankle tendon reflexes and speech difficulties. Abnormal muscle control and tone leads to problems such as scoliosis and foot deformities (high arched feet). Arm weakness can then develop later on. Loss of muscke control forces the passage to a wheelchair. There is no treatment to slow the progression of the disease. The main treatment is to support the patient through psychological support, walking aids, wheelchair, physical and speech therapy. Orthopedic interventions for scoliosis and high arched foot can be necessary. Treatment of associated cardiac disease and diabetes may improve quality of life.