Microscopy PDF
Document Details
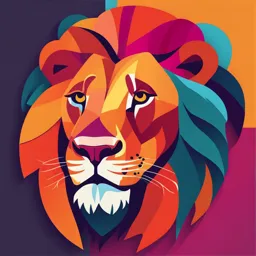
Uploaded by WellMadeStatueOfLiberty9199
School of Nursing and Midwifery, University for Development Studies, Tamale
Dr SKB Bani
Tags
Summary
This document appears to be a set of notes on microscopy, covering topics like types of lenses (concave and convex), properties of lenses and the principles of microscopy.
Full Transcript
. MICROSCOPY Dr SKB Bani 1 MICROSCOPES  Used to view tiny objects that cannot be viewed. with the naked eye.  An ordinary magnifying glass is a simple microscope while  the laboratory microscope is called a compound microscope....
. MICROSCOPY Dr SKB Bani 1 MICROSCOPES  Used to view tiny objects that cannot be viewed. with the naked eye.  An ordinary magnifying glass is a simple microscope while  the laboratory microscope is called a compound microscope. 2 MICROSCOPE  The laboratory microscope has a complex arrangement of lenses.  A lens is a transparent object with one or two curved surfaces.  typically made of glass (or clear plastic in the case of a contact lens). 3. 4. 5 A lens refracts (bends) light and forms an image. An image is a copy of an objected formed by the refraction (or reflection) of visible light. The more curved the surface of a lens is, the more it refracts the light that passes through it. There are two basic types of lenses: concave and convex. The two types of lenses have different shapes, so they bend light and form images in different ways. 6 PROPERTIES OF LENSES Concave Lens 1. is thicker at the edges than it is in the middle. 2. causes rays of light to diverge, or spread apart as they pass through it. 3. Note that the image formed by a concave lens is on the same side of the lens as the object. 7 PROPERTIES OF LENSES Concave Lens 4. The image is also smaller than the object and 5. Image is right-side up. 6. However, it isn’t a real image. It is a virtual image. Your brain interprets it as seeing an image there. 7. The light rays actually pass through the lens to the other side and spread out in all directions (divergent rays). 8 PROPERTIES OF LENSES 9 PROPERTIES OF LENSES Convex lens 1. Is thicker in the middle than at the edges. 2. Causes rays of light to converge at a point called the focus (F). 3. A convex lens forms either a real or virtual image. 4. It depends on how close the object is to the lens relative to the focus. 10 A. Object between lens and focus 11 A. Object between lens and focus 1. Virtual image 2. Same side of the lens as object 3. Image is upright 4. Enlarged 12 B. Object further from the lens than focus 1. Real image forms on the other side of the lens, opposite the object 2. The image in inverted 3. Image may be smaller, larger or same size depending of object’s distance from the lens 4. The further the object, the more reduced the image. 13 CONCAVE CONVEX 1. It is thicker at the edges than the middle. 1. Thicker in the middle than at the edges. 2. Causes rays of light to diverge, or spread apart 2. Causes rays of light to converge at the focus (F). 3. Image formed is on the same side of the lens as the object. 3. A convex lens forms either a real or virtual 4. The image is smaller than the object image, depending on how close the object is to the lens relative to the focus. OBJECT CLOSER THAN FOCUS 1. Image is right-side up. 1. Virtual image 2. However, it isn’t a real image. It is a virtual image. 2. Same side of the lens as object 3. Your brain interprets it as seeing an image there. 3. Image is upright 4. The light rays actually pass through the lens to the other side 4. Enlarged and spread out in all directions (divergent rays). OBJECT FURTHER THAN FOCUS 1. Real image forms on the other side of the lens, opposite the object 2. The image in inverted 3. Image may be smaller, larger or same size depending of object’s distance from the lens 14 4. The further the object, the more reduced the Principal axis = a line passing through the center of the surface of a lens and through the centers of curvature of all segments of the lens. 15 Principal axis = a line passing through the 1. center of the surface of a lens and through the 2. centers of curvature of all segments of the lens. Principal focus = rays parallel to the principal axis entering a convergent lens are refracted and converge at the principal focus. A biconvex lens has Principal Focus on both sides of the lens (depending on the direction of the rays). 16. The distance between the principal focus and the center of the lens is called the focal length. 17 A ray of light entering a convergent lens at an angle emerges parallel to the incident ray and will pass tru the center of the lens. Another ray entering similarly from the other surface of the lens also passes tru the center of the lens. The point at which these two rays meet is referred to as the optical centre of the lens 18 19 Center of curvature = the center of lens surface on either sides of a lens. For a curve, it equals the radius of the circular arc which best approximates the curve at that point. 20. 21. 22 The law of Reflection When a ray of light strikes a surface, it bounces back with. an angle of equal size and it is said to be reflected. 23  Stray reflections inside the microscope interfere with the path of light rays and. degrade the sharpness of the image The field diaphragm located above the light source is responsible for controlling the width of the light beam (but not its intensity). 24 25 The light source of a microscope without. diaphram illuminates the whole specimen, which may be the source of stray light and excessive heating of the specimen. By closing the field diaphragm, it is possible to limit the beam of light only to the part of the specimen which is actually observed. 26 REFRACTION  The bending of light rays from the ‘normal’ when it passes. from one optical medium to another.  caused by changes in the passage of light when it passes from one medium to another of different optical density.  When light enters a more dense medium, it bends toward the ‘normal line.’  The normal line is the line perpendicular to the surface. 27 When light enters a less dense medium, it bends away from the ‘normal line.’. 28 Snell's law Snell's law states that the ratio of the sines of the. angles of incidence and refraction is equivalent to the ratio of phase velocities in the two media, or equivalent to the reciprocal of the ratio of the indices of refraction 29. Snell's law = 30 Limitation of Lenses caused by light, but arise as a result of 1. Shape of the lens. 2. The presence of different wavelengths in white light These limitations give rise to two defects 1. Spherical aberration 2. Chromatic aberration These two defects are corrected by compounding lenses of varying refractive indices and dispersion abilities into a compound microscope. 31 SPHERICAL ABBERATION  is the indistinctive or fuzzy appearance of images due to nonconvergence of light rays to a common focus.  This occurs when the edge of the lens gives a slightly higher magnification than the center of the lens. 32 SPHERICAL ABBERATION 33 SPHERICAL ABBERATION  This results in the loss of contrast, resolution, clarity, and overall focus. Spherical aberration is the property of lenses that have less than perfect spherical shape.  It increases with an increase in the thickness of the biconvex lens.  It can be corrected by compounding with a biconcave lens that brings the image to sharp focus. 34 CHROMATIC ABERRATION Fuzy appearance of the image due to non-convergence of rays of white light to a common focus. Image is surrounded by a multi- coloured fringe, with the blue light being slightly more magnified than the red. 35 CHROMATIC ABERRATION It is caused by splitting white light into its constituent colours while passing through the biconcave lens, which acts as a prism. as white light passes tru the len, the light of shorter wavelength (wavelength 350nm blue) is refracted more strongly than that of a longer wavelength (wavelength 700mn red), 36 CHROMATIC ABERRATION 37 38 CORRECTION CHROMATIC ABERRATION 39 CORRECTION CHROMATIC ABERRATION 40 CORRECTION CHROMATIC ABERRATION 41 CHROMATIC ABERRATION thus a light of one colour is projected at a greater magnification. than another, resulting in the appearance of coloured fringes in the image of the object. Chromatic aberration in the modern microscope is controlled by proper combination of lenses. Achromatic lenses are corrected for two colours while apochromatic lenses are corrected for 3 colours. 42 CORRECTION CHROMATIC ABERRATION 43 44 45 COMPOUND MICROSCOPE 46 TOTAL MAGNIFICATION OF THE MICROSCOPE In a compound microscope, the magnification is produced by two sets of lenses. The objective (real, magnified, inverted) which is brought into sharp focus by the eye piece lens. The primary image is within the focal length of the eye piece lens. A magnified virtual image is produced by the eye piece lens. 47 PROPERTIES OF LENSES The total magnification of the microscope is thus the magnification of the objective multiplied by the magnification of the eye piece lens. 48 USEFUL MAGNIFICATION: When the magnification results in revealing more details EMPTY MAGNIFICATION: if the magnification of the object fails to bring out further details and loses its sharpness. 49 MICROSCOPE USE AND CARE Always grip the microscope by the arm and put your hand beneath its base. Hold the scope upright at all times. 50 MICROSCOPE USE AND CARE ONLY the 100X (oil immersion) objective uses oil. DO NOT get oil on any of the other objectives; the oil will ruin them, and you’ll not be able to focus at those powers. 51 MICROSCOPE USE AND CARE  Large specimens, should be examined at low power only. They are too big to magnify further, and efforts to do so will break the slide and can damage the objective. 52 MICROSCOPE USE AND CARE When you put the scope away, remember to 1. Remove the slide and return it to its proper place. 2. Clean any oil off the 100X objective. Clean the other objectives too. 3. Turn off the light. 53 MICROSCOPE USE AND CARE When you put the scope away, remember to 4. Loop the cord up on itself , secure it, and don’t let it dangle freely. 5. Replace the cover. 6. Do not tamper with any of the components of the microscope. 54 MICROSCOPE USE AND CARE 7. Always be certain that the low-power objective is in place before putting the microscope away. 8. Always unplug the electrical cord by pulling on the plug, not the cord. 9. Never look through the microscope while rapidly reducing the distance between the objective lens and the slide. 55 MICROSCOPE USE AND CARE Always carry the microscope with two hands. Keep the microscope at least 6 inches from the edge of the lab table, and keep the excess electrical cord on the table top. Do not touch the glass lens with your fingers. Clean the lenses with lens paper only, and wipe the lenses before and after each use. Always wipe the oil from the oil-immersion objective with lens paper before putting the microscope away. 56 MICROSCOPE USE AND CARE 57 MICROSCOPE Magnifying power of Objective lens 1. Low power objective, Marked x10, 16mm, 2/3inch Coded yellow ring).  Used for rapid scanning of the field. 58 Magnifying power of Objective lens 2. High dry Objective: Marked x40, 4mm, 1/16inch, Coded with a blue ring, Usually the highest dry (without immersion oil) magnification. 59 Magnifying power of Objective lens 3. Oil immersion objective, Highest magnification in the ordinary microscope. Coded x100, 2mm, 1/12inch, white ring. Always oil immersed. 60 MICROSCOPE 61 MICROSCOPE 62 MICROSCOPE Magnifying power of Objective lens Cedar wood oil which has similar optical density as glass, hence similar refractive index as glass. Is placed between the lens and object to prevent refraction of light. It enables the light to pass tru the glass, tru the oil and to the lens glass as though it had passed tru glass all the ways. 63 MICROSCOPE Resolving power of the microscope The limit of useful magnification is set by the RP of the microscope The resolving power of a mic obj is the ability to reveal closely adjacent points as separate and distinct. Quantitatively, it is the capacity to distinguish two neighbouring points as separate entities. 64 MICROSCOPE Resolving power of the microscope Depends largely on the amount of light entering the obj and the refractive index of the medium btw the obj and the object. The presence of oil between the obj and the object conserves much of the light which would have otherwise have been lost tru refraction 65 Resolving power of the microscope  the ability to reveal closely adjacent structural details as being actually separate and distinct.  tells you the minimum distance between two points that can be seen as distinct by the objective lens. If it is less, the points will be seen as one.  The increase in magnifying power is always linked to an increase in resolving power.  The higher the resolving power of an objective, the closer can be the fine lines or small dots in the specimen which the objective can separate in the image. Resolving power of the microscope  The resolving power of an objective is dependent on what is known as the numerical aperture (NA) of the objective.  The numerical aperture is a designation of the amount of light entering the objective from the microscope field,  i.e. the cone of light collected by the front lens of the objective (an index or measurement of the resolving power). MICROSCOPE Resolving Power (RP) = 0.61*λ NA λ = wavelength NA = Numerical Aperture When further magnification fails to show the two points as distinct, it is called Empty magnification. 68 Numerical Aperture It is dependent on the diameter of the lens and the focal length of the lens. Focal length has an inverse relationship with numerical aperture (NA), meaning that as focal length decreases, numerical aperture increases: The following are the usual numerical apertures of commonly used objectives. 10 X objective ----------- NA 0.25 40 X objective ----------- NA 0.65 100 X (immersion oil) objective ------- NA 1.25 MICROSCOPE Numerical Aperture (NA)  measure of how much light can be collected by an optical system such as the microscope lens.  The NA of a microscope objective is the measure of its ability to gather light and to resolve fine specimen detail of the object (or specimen).  Image-forming light waves pass through the specimen and enter the objective. 70 MICROSCOPE Resolving Power (RP) = 0.61*λ NA λ = wavelength NA = Numerical Aperture 71 72 73 74 75 76 MICROSCOPE  The use of a wrong working distance can damage the front lens of the obj especially in high power objectives.  to reduce such damages, SPRING LOADED OBJECTIVES are used.  In modern mics, the objectives are par-focal. When an objective is used to focus on object, the object is still in focus, and a little touch on the fine adjustment when another obj is swung into position.  Prevents the possibility of damaging the objective lens when changing from a low power obj to a high power obj during focussing.  Also prevents the lens of the objective from being scratched. 77 MICROSCOPE What is the working distance of an objective?  Microscope objectives are generally designed with a short free working distance,  which is defined as the distance from the front lens element of the objective to the closest surface of the coverslip when the specimen is in sharp focus 78 Microscopy Filters MICROSCOPE  increase contrast and resolution  block ambient light  removing harmful ultraviolet or infrared light,  to selectively transmit only wanted wavelengths.  adjust colour balance of light to give the best visual effect.  produce monochromatic light when necessary  absorb heat from the high intensity lamps  transmit light of a selected intensity  protect the eyes from injury by ultra violet rays. 79 Microscopy Filters 80 SOURCES OF ILLUMINATION MICROSCOPE 1. Day light Direct day light injurious, better to use reflected day light. Day light is sufficient for use in mono-ocular mics but too weak in oil emersion objective. 2. Electric light 60wats electric bulb, provides adequate illumination 3. Battery In the absence of electricity, a 6volts mic lamp can be connected to a 6volts battery. 4. Mirror Mics without a built-in source illumination can have a mirror mounted below the condenser and iris diaphragm 81 BRIGHT FIELD MICROSCOPY 82 BRIGHT FIELD MICROSCOPE Bright-field microscopy is the  simplest of a range of techniques used for illumination of samples in light microscopes  and its simplicity makes it a popular technique.  The typical appearance of a bright-field microscopy image is a dark sample on a bright background, hence the name.  It has a direct light source that illuminates the object to make it visible tru the microscope. 83 BRIGHT FIELD MICROSCOPE Amplitude specimens possess color and are able to decrease the brightness of the passing light all on their own. These specimens are best observed using bright- field microscopy. Pigmented structures (such as chloroplasts) and specimens that are selectively stained are examples. 84 BRIGHT FIELD MICROSCOPE 85 BRIGHT FIELD MICROSCOPE  Widely used for stained or naturally pigmented or highly contrasted specimens mounted on a glass microscope slide.  The specimen is illuminated from below and observed from above.  The specimen appears bright, but DARKER THAN THE BRIGHT BACKGROUND. 86 BRIGHT FIELD MICROSCOPE Principle of BFM  A specimen is placed on the stage of the microscope and incandescent light from the microscope's light source is aimed at a lens from beneath the specimen.  The objective magnifies the light and transmits it to an eyepiece and into the user's eyes. 87 BRIGHT FIELD MICROSCOPE Limitations of BFM 1. Many objects such as microorganisms and cells are transparent, giving very poor contrast with bright light and are not very visible unless they are stained with a coloured dye. 2. Since many staining techniques lead to the death of the specimen, it is impossible to use BFM to view living organisms. 88 BRIGHT FIELD MICROSCOPE 89 DARK-FIELD MICROSCOPE 90 DARK-FIELD MICROSCOPE PRINCIPLE OF DFM  Light microscope equipped with a special condenser and objective which brightly illuminate the object against a dark background.  Condenser has a special blacked-out area, which prevents light coming from the light source from entering the microscope objective, making the scope field appear dark.  The path of light is directed in such a way that it passes tru the outer edge of the condenser at a wide angle. 91 DARK-FIELD MICROSCOPE  In its path, the light strikes the object plane. The light from the object enters the objective, only light that is scattered by objects on the slide reaches the eye.  Instead of coming up through the specimen, the light is reflected by the specimen on the slide.  making the object appear bright against a dark background. 92 DARK-FIELD MICROSCOPE 93 DARK-FIELD MICROSCOPE 94 DARK-FIELD MICROSCOPE 95 DARK-FIELD MICROSCOPE Application of DFM 1. Demonstration of Treponema Pallidum in chancre fluid, the motility of which cannot be seen in ordinary light microscope. 2.Detection of leptospira in urine 3. Demonstration of Borrelia in blood 4.Demostration of vibrio cholera and Campylobacter species in stool 96 DARK-FIELD MICROSCOPE 97 DARK-FIELD MICROSCOPE 98 DARK-FIELD MICROSCOPE 99 DARK-FIELD MICROSCOPE Limitation of DFM 1. Difficult setting, centering and focussing the dark field condenser 2. Using slides and cover slips that are not completely clean make viewing of objects difficult due to interference from particles 3. Difficult in examining too dense preparations, bcos particle absorbs more light instead of scattering. 4. The presence of air bubbles in the immersion oil makes viewing difficult 5. Insufficient oil contact between the slide and the condenser makes viewing difficult. 100 FLUORESCENCE MICROSCOPE 101 FLUORESCENCE MICROSCOPE Principle A substance is fluorescent when it is able to absorb light of shorter wavelength and energy (blue light) and emit light of longer wavelength and lesser energy (green, red etc light). Application of this phenomenon is the basis of fluorescence microscopy. Fluorescence is adopted in the lab to identify antibodies, microorganisms and many other substances. In practice, MICROBES ARE STAINED with a FLUORESCENT DYE and then ILLUMINATED WITH BLUE LIGHT. The dye absorbs blue light (shorter wavelength) AND EMITS GREEN LIGHT (longer wavelength). 102 FLUORESCENCE MICROSCOPE In a fluorescence microscope, a high intensity mercury arc lamp is used as the light source. It emits white light, which is passed through an ‘exciter filter’. It allows only the blue component of the white light (the white light consists of seven colors, which in the decreasing order of wavelength are violet, indigo, blue, green, yellow, orange and red) to pass through it and blocks out all other color component A DICHROIC MIRROR, which REFLECTS BLUE LIGHT, but ALLOWS GREEN LIGHT TO PASS TRU IT is used on the path of the BLUE LIGHT. The mirror is fixed at such an angle that the blue light is REFLECTED DOWNWARD TO THE SPECIMEN. 103 FLUORESCENCE MICROSCOPE  Certain portions of the specimen retain the dye, while others do not.  The portions, which retain the fluorescent dye, absorb blue light and emit green light.  The emitted green light goes upward and passes through the dichroic mirror.  It reflects back blue light, if any, and allows only green light to pass through it. 104 FLUORESCENCE MICROSCOPE  Then, the light reaches a ‘barrier filter’. It allows green light to pass to the eye and blocks out any residual blue light from the specimen, which might not have been completely reflected by the dichroic mirror.  Thus, the eye perceives the stained portions of the specimen as glowing green object against a jet-black background, whereas the unstained portions of the specimen remain invisible.  When fluorescence is used to detect ag-ab rxn, it is called immunofluorescence. 105 FLUORESCENCE MICROSCOPE In FM, the background is dark because there is no visible light, and only the specimen (cells, microorganism etc) stained with fluorochrome appears bright. 106 107 108 109 FLUORESCENCE MICROSCOPE The specimen is previously stained with a fluorescent dye known as fluorochromes, such as 1. acridine orange NO, 2. acridine yellow, 3. acriflavine, 4. thioflavine S, 5. thioflavine T or 6. titan yellow G. 7. fluorescin isothiocyanate (FIT) 8. auramine fluorencent dye 110 FLUORESCENCE MICROSCOPE 111 FLUORESCENCE MICROSCOPE 1. Detect AFBs in sputum or csf when stained with auramine fluorencent dye 2.Examination of acridine-orange stained specimens for the detection of Trichomonas Vaginalis, intracellular gonococci and other parasites and microoganisms 3.FM is used in immunodiagnostics in both direct and indirect antibody techniques. Very useful in monoclonal reagents because of its high specificity. 112 FLUORESCENCE MICROSCOPE  Advantages Fluorescence microscopy One essential advantage of fluorescence microscopy, however, is the presence of fluorescent molecules themselves. Although a structure is too small to be resolved in a light microscope, the emission of light remains visible, making it possible to resolve in the fm.. 113 FLUORESCENCE MICROSCOPE  Fluorophores lose their ability to fluoresce as they are illuminated. The process is photobleaching.  Photobleaching occurs as the fluorescent molecules accumulate chemical damage from the electrons excited during fluorescence. 114 FLUORESCENCE MICROSCOPE Limitations of FM  Photobleaching can severely limit the time over which a sample can be observed by fluorescent microscopy.  Several techniques exist to reduce photobleaching such as the use of 1. more robust fluorophores, 2.by minimizing illumination, or by 3. using photoprotective scavenger chemicals.  Fluorescence microscopy with fluorescent reporter proteins has enabled analysis of live cells by fluorescence microscopy, however cells are susceptible to phototoxicity, particularly with short wavelength light. 115 FLUORESCENCE MICROSCOPE  Furthermore, fluorescent molecules have a tendency to generate reactive chemical species when under illumination which enhances the phototoxic effect.  Unlike transmitted and reflected light microscopy techniques fluorescence microscopy only allows observation of the specific structures which have been labeled for fluorescence.  For example, observing a tissue sample prepared with a fluorescent DNA stain by fluorescent microscopy only reveals the organization of the DNA within the cells and reveals nothing else about the cell morphologies 116 PHASE CONTRAST MICROSCOPY 117 PHASE CONTRAST MICROSCOPY  An advantage of this method is the ability to study cell structure without dyes or killing the cell or organism. This makes it possible to study the cell in situ.  The phase contrast is a modified type of light microscopy that enhances the contrasts between the different structures of the object because of their thickness or optical densities.  In bright-field microscopy, the unstained object in the specimen are visible because of the amplitude of light rays passing tru them, producing a contrast with the surrounding medium. 118 PHASE CONTRAST MICROSCOPY  When a light ray passes tru a part of the specimen with lower density, a very small amount of light is absorbed and the emerging ray of light will have a comparatively high amplitude or intensity.  Another ray of light passing tru a denser part of the specimen will emerge with a comparatively lower amplitude or intensity because more light is absorbed. This part of the specimen will appear darker.  These differences in intensity/amplitude are subtle and the human eye is not sensitive enough to see the minute differences.  Phase contrast converts optical densities or refractive indices into amplitude changes which are easily visible to the eyes. 119 PHASE CONTRAST MICROSCOPY  A wave of light is made of rays travelling in a straight line. When two such waves travel together, they are said to be in ‘phase.’  Such a ray will appear bright to the observer. However, if one the rays is held up or made to change path, they will no more travel together and they are said to interfere with each other, differing in the intensity.  In PCM, a special condenser and objective controls that illuminates in a way that accentuate the differences in optical densities. 120 PHASE CONTRAST MICROSCOPY  It causes light to travel different routes tru various parts of the specimen. This results in an image with differing degree of darkness and brightness, collectively called contrast.  In effect, the phase contrast technique employs an optical mechanism to translate minute variations in phase into corresponding changes in amplitude, which can be visualized as differences in image contrast.  One of the major ADVANTAGES of phase contrast microscopy is that living cells can be examined in their natural state without previously being killed, fixed and stained.  As a result, the dynamics of ongoing biological processes can be observed and recorded in high contrast with sharp clarity of minute specimen detail 121 PHASE CONTRAST MICROSCOPY 122 BFM VRS PCM MICROSCOPE Specimens that do not possess much color but a different refractive index that the surrounding mounting medium can be referred to as PHASE SPECIMENS as they cause a phase-shift in of the light. The unaided human eye is not capable of detecting this phase shift. This phase shift is then converted into a brightness difference by the optics of the phase-contrast microscope. Bacteria are a good example here. They are nearly completely transparent but nevertheless appear darker or brighter (depending on the optics) than the background. 123 BFM VRS PCM MICROSCOPE Amplitude specimens possess color and are able to decrease the brightness of the passing light all on their own. These specimens are best observed using bright- field microscopy. Pigmented structures (such as chloroplasts) and specimens that are selectively stained are examples. Many specimens are a combination of these two. Here the choice of the right kind of microscope is important in order to see that what one wants to see. If you want to enhance contrast based on colour, then the BFM is ideal, but if base on optical densities, them the PCM is ideal. Phase contrast microscopes will optically darken certain structures to the extent that it is not possible to see the natural color of the structure. In this case it is probably better to use bright field microscopy. Stained bacteria, for example, should be observed in bright field. 124 PHASE CONTRAST MICROSCOPY Applications of PCM 1. Examine unstained bacteria, e.g. cholera vibrios in specimens and cultures 2. Examining wet preparations like urine, vaginal swabs etc 3. Examining stool preparations for trophozoite of amoeba eg Amoebae in faecal preparations 4. Examining filarial in blood and other body fluids Trypanosomes in blood, cerebrospinal fluid 5. Detection of parasites in lymph gland fluid ; Promastigotes of leishmanial parasites in culture fluid; 6. Trichomonas species in direct smears and cultures; 7. Urine sediments. 125 PHASE CONTRAST MICROSCOPY  Transparent microorganisms suspended in a fluid may be difficult and sometimes impossible to see.  Phase contrast makes it possible for these to be seen. 127 ELECTRON MICROSCOPY 133 ELECTRON MICROSCOPY 134 The electromagnetic spectrum © 2016 Paul Billiet ODWS 135 ELECTRON MICROSCOPY  Maximum resolution with a light microscope which uses light with wavelengths between 300-700nm to illuminate the object is limited to 0.2µm or 200nm.  Viruses and other fine structures cannot be seen with the light microscope.  A beam of electrons having a wavelength of 0.05nm is used, the resolution is increased dramatically by 2000times (0.1nm). PRINCIPLE OF ELECTRON MICROSCOPY  In an electron mic, the beam of electrons is converged by a circular magnetic field. The EM use electromagnetic lenses. The convergence of an electron beam produces an image.  The image and object distances are related to the focal length of the lens in exactly the same as in the light optics.  The electron microscope is therefore constructed on the same optical principles as in the light microscope and the same formula can be used to correlate the magnification and focal distances. 137 PRINCIPLE OF ELECTRON MICROSCOPY The electron beam is derived from a heated tungsten filament which is surrounded by a metal cylinder known as the Whenalt cap. This cap serves to shape the electron beam. Just beyond is the whenalt cap which is the anode which has an aperture tru which the electron beam passes. A large voltage is applied between the cathode and the anode. This gives the electrons their high velocity. The wl of the electrons is inversely proportional to the velocity of the electrons, therefore the electrons have short wls (0.1- 0.5Å/0.01-0.05nm). 138 PRINCIPLE OF ELECTRON MICROSCOPY  The electron beam first passes tru the condenser lens. As in the LM, this condenser lens help focus the electrons unto the specimen.  The lens of the EM consist of circular electromagnetic fields.  The magnetic lens of an EM can have different powers (focal length and magnification) depending on the amount of current flow tru the electrical coils. 139 PRINCIPLE OF ELECTRON MICROSCOPY In the EM, the lenses are rigidly fixed (variable in the LM), but the lens are movable with respect of the object so that the image can be focused and proper conditions of illumination obtained. In the EM, focal lengths are variable by changing lens currents. Thus the illumination of the object is achieved by varying the current in the condenser lens. 140 PRINCIPLE OF ELECTRON MICROSCOPY 141 Principle of Electron microscopy 142 PRINCIPLE OF ELECTRON MICROSCOPY  The imaging system of the electron microscope has two lenses; the objective and the projector lenses.  A third intermediate lens, may be present between the two. This gives 3 levels of magnification and makes it possible to achieve higher magnification in a reasonable amount of space. 143 PRINCIPLE OF ELECTRON MICROSCOPY  The numerical aperture of the EM is very small  The scanning transmission electron microscope has achieved better than 50 pm (10-12) resolution in annular dark-field imaging mode and magnifications of up to about 10,000,000x  whereas most light microscopes are limited by diffraction to about 200nm (10-9)resolution and useful magnifications below 2000x. 144 PRINCIPLE OF ELECTRON MICROSCOPY  The objective is placed with its focal point close to the object. Intermediate images are formed between each lens system. The projector throws its image on to the fluorescent screen or photographic plate to make permanent record.  The entire imaging system is usually referred to as the scope column and its constructed upside down compared to the light microscope. The electron source and condenser are places above the image formed below.  The column is very rigidly constructed and maintained at very high vacuum since air molecules would deflect the electrons beam. The specimen is introduced into the object plane through an air lock system. Therefore, it is not possible to examine living materials in the electron microscope. 145 Principle of Electron microscopy  Electron optics are essentially similar to light optics. One important difference, however is that, the formation of the image is due to scattering of the electrons by the molecules of the specimen.  This scattering solely depend on the mass densities. Elements of high atomic weight such as lead or uranium cause marked electron scatter and appear dense in the electron image.  The lighter elements such as carbon, oxygen and nitrogen cause less electron scatter and give poor contrast. 146 Principle of Electron microscopy  Most biological stains depend on the absorption of light of certain wavelengths of light due to their molecular structure and are composed mainly of carbon nitrogen and hydrogen atoms.  Since these are all of the low weight, they have very little electron scattering power and are not generally useful as stains for electron microscope.  Unstained tissues have poor contrast in electron microscopy. They may be stained by a variety of metal salts.  Most of such stains are relatively non specific , eg, lead citrate, lead hydroxide and uranyl acetate. The sections are supported on thin plastic grids. 147 Principle of Electron microscopy DISADVANTAGE  It is necessary to examine the objects in vacuum because the gas molecules in the air can scatter electrons and distort the image.  Therefore, only dead and dried objects can be examined in the electron microscope, which may lead to considerable distortion in the object (imagine dried rbc).  There are several techniques available in electron microscopy, including staining methods with heavy metals and radioactive substances.  The method used depends on the type of microscopy and the purpose of the examination. 148 This is the inner surface of a trachea which I captured under the Scanning Electron Microscope (SEM). The cilia, 149 which are each about 1/4 of a micron in diameter, keep your air passages clean. X8000 magnification. MICROMETRY refers to the measurement of dimensions of the desired microorganisms under a microscope using two micro-scales known as 'micrometers’. At first, the diameter of the microscopic field must be established with the help of these 2 micrometers namely ocular micrometer and stage micrometer Measurement of microscopic objects using a calibrated eyepiece micrometer. The unit of measurement is the µm. The eyepiece micrometer is calibrated for each microscope objective at which the measurement is required. The eyepiece micrometer is calibrated using a scale engraved on a micrometer glass slide. 150 MICROMETRY Calibrating the eyepiece micrometer 1. Unscrew the upper lens of a 10x eyepiece and insert the eyepiece micrometer disc, with engraved side facing downward. 2. Place the stage graticule slide on the stage. Rotate the right objective into position and bring both the eyepiece scale and the stage scale into clear focus. 3. Adjust the field until the 0-line of the eyepiece scale aligns with the 0-line of the stage calibration scale. 4. Look along the scales and note where the stage scale perfectly align with the eyepiece division. 151 MICROMETRY Calibrating the eyepiece micrometer 5. Measure the distance between the 0-line and where the alignment occurs on the graticule. The measurement of the calibration scale are usually 1mm=1000µm. 6. Count the number of division on the eyepiece from 0-line to where the alignment occurs. 7. Calculate the measurement of 1 division on the eyepiece scale in µm 8. Subsequently measure the organism with the ocular scale, count the divisions and multiply by the measurement of one division on the eyepiece scale in µm 152 MICROMETRY 153 STAGE MICROMETER 154 STAGE MICROMETER 155 STAGE MICROMETER 156 157 STAGE MICROMETER 158 MICROMETRY 159 MICROMETRY 160 MICROMETRY 161