Synapses: Membrane Excitability PDF
Document Details
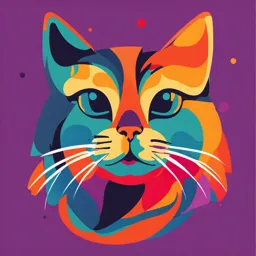
Uploaded by SplendidNovaculite8819
Università degli Studi di Milano Bicocca
Tags
Related
- Biological Foundations of Mental Health Notes - King's College London
- Lecture 10 - Nervous Tissue - Tagged PDF
- Neurology Foundations (OCCTH 583) PDF
- Task 2 - Neurons PDF
- Midterm 1: Introduction To Nervous System PDF
- Biopsychology, Global Edition, 11th Ed - Neural Conduction and Synaptic Transmission PDF
Summary
This document provides an overview of membrane excitability, focusing on the mechanisms of synaptic transmission. It discusses various types of synapses, highlighting the differences between electrical and chemical synapses. The role of calcium ions in neurotransmitter release and the process of exocytosis are also discussed.
Full Transcript
PHYSIO #4 – MEMBRANE EXCITABILITY: SYNAPSES The axon potential can be established at the terminal portion of the axon or at the axon hillock when the minimal intensity is reached, the so called rheobase. The absolute refractoriness is a window of time that cannot be decreased. There are neurons th...
PHYSIO #4 – MEMBRANE EXCITABILITY: SYNAPSES The axon potential can be established at the terminal portion of the axon or at the axon hillock when the minimal intensity is reached, the so called rheobase. The absolute refractoriness is a window of time that cannot be decreased. There are neurons that can discharge with a faster or slower range, so they are not the same in terms of membrane potential and properties: neurons of different sites of the brain have their own feature in frequency of discharge. At a certain point the neuron bumps in the absolute refractoriness. 1. Signals to communicate The origin of action potential is possible on the axon hillock or at the end of the axon. The classical neuron has the soma, dendrites, and a long axon with the synaptic terminal. The action potential is used as a messenger through which the neurons communicate. Communicating without dissipation is essential to communicate in long distances. The graded potential will never reach the target, therefore the evolution of the digital signal with a smart conduction is essential. e.g., sensory action potential conveys to the brain, the sensory neuron receives the information and the other branch of the pseudounipolar neuron will enter the spinal cord or the brainstem of CNS. That part of the axon will end with a synaptic terminal to communicate a signal. The action potential is therefore a signal to communicate. 2. Modalities of IC cellular signaling There are different modalities of cellular signaling: - Nonspecialized synapses o Humoral: molecules (hormones) in the blood can be released in the interstitial fluid and reach their target. o Paracrine: communication by releasing molecules that will spread around and act on neighboring targets (they do not go into the blood). o Autocrine: the cell releases a molecule that acts on the cell itself - Chemical & electrical synapses – physical stimulation/synapse o Electrical synapses: cells are connected by gap junctions, communication is physical via this channel, cells are very 1 close in term of physical distance but there is not a common device o Chemical synapses: there is chemical signal that reaches the other cell. Synapses, both electrical and chemical, are the devices through which neurons communicate. 3. Electric synapses The electrical synapse is a communication through gap junctions. There is no pre- and a post-synaptic neurons, there is just a direction of the impulse that can go in both directions. It is formed of 2 separated membranes which never merge together. They are separated by a minimal distance and contain transmembrane proteins, GAP junctions (that are channels (2 subunits)). a. GAP junction GAP junctions consist each of 2 (hemi)channels perfectly in line. They are geometrically aligned so that the pore 1 is in continence with pore 2 but the two membranes are separated and the channels are not merged together. This heometric alignment allows possiblily to obtain a minimal resistance thanks to the channels. This creates a patch of the membrane with a lot of pores that have minimal resistance for ions. This is a functional merging: whatever enters the channel will go directly to the other channel because they are so close that it cannot go away. Functionally, it is a unique channel, but structurally it is made by 2 subunits. There can be also other devices that condition the opening of these sites: normally, they are considered open as leak channels but they can also be in a closed conformation. The only difference with channels is that they are paired channels and the ions flow by means of diffusion. b. Transmission Considering an inward current in a cell, the charges that distribute in the membrane enter in the other patch of membrane and if there is an electrical gradient, they end up in the other cell. 2 e.g., if there is a huge inward current of sodium this will be called by the electronegativity and by the chemical gradient in the cell at rest. The sodium will pass from the excited cell to the resting cell that will be depolarized or hyperpolarized depending on the ion. If this is multiplied for a huge number of ions the current becomes very important. The synapse is a place of very high conductance for an ion. The membrane potential is in the presynaptic membrane, after a while there will be depolarization in the other. The synaptic delay is very brief: 0.1ms c. Model of study: snail aplysia Snail aplysia releases ink when is aware of a danger. One of the way to release the ink is the stimulation of the tail. Neurons are connected to the gland that releases the ink. Recording the activity of these neurons and stimulating one of them, it was expected a bigger efficiency of releasing ink the more neurons are stimulated. The efficiency would ground on the number of neurons able to target the gland. However, it was found that if there is only a moderate stimulation in one of the neurons, the amount of ink is the same: this is because they are connected with gap junctions so what happens in one is easily transmitted to the other ones, causing simultaneous activation of a lot of cells together (this recalls the heart & smooth muscle organization as it is full of gap junctions which involve a huge number of cells in a short time). There is a wave through the gap junctions, it is an efficient device that is represented by a lot of structures for a powerful excitation of a lot of cells together. GAP junctions, and therefore electrical synapses, allow the possibility for rapid and synchronous discharges. 3 d. Electric synapses in the heart The heart is an electrical syncytium because the myocardiocytes are connected by GAP junctions, through which not only the ions can be transmitted but also metabolic peptides. Another advantage of this junction is that it is bidirectional. There is no preferential direction: the direction is determined by the first cell that depolarizes. The electrical synapse is formed by a huge multitude of channels. In some specific structures the directionality is important and is given by other systems, for example the muscle needs to have a simultaneous activation with a precise direction, but if something blocks somewhere the bidirectionality guarantees the transmission to all the areas. 4 Chemical synapses In the chemical synapses there is a distinction between the pre and post synaptic membrane and a space in between that is the synaptic cleft. At the level of the presynaptic membrane, there are the synaptic vesicles disposed in a precise way: some very near and some other layers beyond. In the presynaptic vesicles are neurotransmitters (NT). 4 On the post synaptic membrane there are devices called receptors made by a protein on the membrane that is receiving a signal in the interstitial fluid. The electrical activity arriving at the synapse leads to the fusion of the vesicle with the cell membrane and the consequent release of vesicles by exocytosis. (1) The NT is synthesized and stored into vesicles (2) An action potential invades the pre-synaptic terminal 5 (3) The depolarization od the presynaptic terminal causes the opening of the voltage gated Ca2+ channels. (4) There is an influx of Ca2+ through channels (5) Ca2+ causes vesicles to fuse with the presynaptic membrane (6) The NT is released in the synaptic cleft via the exocytosis (7) The NT binds to the receptor molecules in the post-synaptic membrane (8) Opening or closing of post-synaptic channels (9) Post synaptic current causes excitatory or inhibitory post)synaptic potentials that change the excitability of the post-synaptic cell (10) The retrieval of the vesicular membrane from the plasma membrane a. Calcium: the mechanical mediator Calcium is the intermediate step: it works as a mechanical mediator. When the action potential arrives, the membrane is depolarized, calcium voltage gated channels present ALO pre-synaptic terminal open and Ca enters the cell because of the electrochemical gradient. Calcium enters for a short time as the depolarization is very short ( the membrane comes back to negative value). Moreover, the equilibrium potential of calcium is +130mV so there is a lot of driving force favouring its entrance. Calcium binds to proteins of the cytoskeleton and different other proteins involved in synaptic events. The calcium interacting with these proteins, favours the movement of vesicles and the release of neurotransmitters. There is also the repolarization of the membrane and reuptake of the vesicles. 6 The chemical synapse is very precise because the two membranes are very close and the receptors are expressed in huge number in the post synaptic membrane. The regions of the membrane close to that region are without these receptors in such a way that there is a higher probability to bump in the receptors. The neurotransmitter released into the synaptic cleft binds the receptor that will mediate the post synaptic events. The receptors can be different depending on the channels that can be opened by the neurotransmitter but they are always electrical events. The receptor can be a channel that opens directly or it can be in communication with a second messenger that mediates the change in conductance for specific ions. Then the current decides which is the effect. Summarizing the events: 1. Action potential arriving 2. calcium voltage gated channels open 3. release of neurotransmitters 4. binding of neurotransmitters to receptors 5. opening of channels and post synaptic change in membrane potential. 6. variation in membrane potential, this is another graded potential: the amplitude depends on the amount of neurotransmitter, the duration depends on the duration of the activation of the synapse. b. Active zone on the presynaptic membrane The active zone is the portion of the membrane where there are the vesicles ready to be released, it is geometrical and precise: the number of vesicles released by one action potential is well defined. The synapse is built up in such a way that there is a release of a determined amount. The active zone is very geometrical, but it still assures a certain variability in amount of neurotransmitters released, nothing is fixed. It is characterized by a quantal release: the sum of the different releases is due to the different action potentials. c. The importance of Ca2+ Calcium, to be mechanically active, must increase in concentration. However, calcium in the cytoplasm is not even detectable, it is concentrated outside or in RER. The amount of calcium that enters in 2ms is not enough to change the intracellular concentration. The percentage of [Ca2+] increasing in the synaptic button is irrelevant. IC devices are therefore optimized: the calcium channels are architecturally near the vesicles, so they create micro-domains of calcium increase. Vesicles are released by calcium micro-domains near the calcium channels through which the calcium passes by diffusion. The overall concentration will not be affected but only in that particular area. The time will be enough and will be very efficient as a system. The phenomenon will never be explained by measuring the average [Ca2+], but only the local [Ca2+]. 7 The channel arrangement is truly responsible for an efficient synaptic transmission. As soon as there’s a channel, there are pumps that allow changes in concentration of Ca2+ since the unequal distribution is the one assuring the electrochemical gradient. Without that, there would be no diffusion of Ca2+ from outside to inside (this is true for all ions). As soon as there is the activation of the calcium channels, the calcium inside increases and the pumps put the calcium outside the cell. When the substrate increases, the pumps increase their efficiency, which is slower than diffusion. So, there’ll be a window of time explaining the release of the neurotransmitter. The functional architecture of the device is what assures a regularity of this release: there’s a translation from an electrical signal into a chemical signal that must become again an electrical signal on the post synaptic membrane. The background needs to be regular and well organized, having a perfect anatomo-functional structure. At the synaptic level, the vesicles are pushed towards the membrane, they anchor to it and then diffuse. The vesicles then have to go up towards the body of the neuron to be refilled with neurotransmitters since they’re produced in the soma. Some vesicles go up the axon to be refilled while the others come down ready to be released. d. Receptors Receptors on the post-synaptic cell play their role in the second step of synaptic transmission. The action of a neurotransmitter on the post-synaptic cell depends on the properties of the receptor it binds to. For example, Ach can induce both an excitatory and inhibitory action, whether the receptor is either nicotinic or muscarinic. They are typically membrane-spanning proteins which have the ability to convert the chemical signal back to an electrical one, influencing the opening and closure of ion channels of the post-synaptic cell and finally inducing a graded potential called post-synaptic potential. 8 Receptors can be channels themselves (ionotropic channels) or can be proteins that in the end induce a change in the channel configuration (metabotropic channels) Ionotropic channels (case A) are composed of - an extracellular domain which binds the neurotransmitter, - a membrane spanning domain that forms an ion-conducting pore. When the EC domain binds to the chemical messenger, it induces a conformational change in the whole receptor, opening the gated channel portion (ligand-gated channels). An example of this kind of receptor is the Ach receptor at the neuromuscular junction. Metabotropic receptors (case B) are separated from the ion channels they regulate. They are composed of one or two subunits with several (usually seven) membrane spanning domains that are able to induce a signaling cascade inside the post-synaptic cell. In fact, binding of the neurotransmitter switches the receptor into an “active” state that stimulates the production of second messengers such as cAMP or diacylglycerol. In turn, second messengers activate protein kinases that in most of cases act directly on the gated channels to open them. An example of this kind of receptor is the norepinephrine one in neurons of the cerebral cortex. e.g., acetylcholine nicotinic receptor is one of the two types of acetylcholine receptors (nicotinic, which is an ionotropic receptor and muscarinic, which is metabotropic). So, neurotransmitters can also have different types of receptors. e.g., GABA (gamma aminobutyric acid) receptor, an ionotropic receptor for chloride. e.g., metabotropic receptors 9 There’s no excitatory or inhibitory neurotransmitter. It is not the neurotransmitter that defines the line of events but it’s the receptor. For the same neurotransmitter, there can be different receptors with opposite effects. Therefore, the post synaptic events (change in synaptic potential) can be a depolarization or hyperpolarization depending on the type of channel. e.g., modulating or closing a potassium channel will result in a depolarization but by modulating or closing a sodium channel, the effect will be opposite (an hyperpolarization). In some specific cases, the neurotransmitter can make a second messenger modify a pre-existing protein or regulate the expression of some other receptors. This is the basic for the long-term changes at the level of the synapses. e. Post synaptic potentials The post-synaptic potential can be excitatory (EPSP) or inhibitory (IPSP). “Depolarization” means going towards the threshold. If the cell is excited, it means bringing the membrane towards the threshold without having to trigger the potential. Modulate the excitability of the potential means changing the membrane potential. So, just the fact that there’s a movement towards the threshold means there’s an excitation. - Excitatory post synaptic potential (EPSP): sodium in – depolarization - Inhibitory post synaptic potential (IPSP): potassium out, chloride in – hyperpolarization The post synaptic potentials are graded potentials that occur on the soma and dendrites. They need to arrive to the axon hillock in order to be transformed into a single action potential. The converter is the first node of Ranvier (dendritic) and axon hillock (somatic). It will convert the graded potentials into action potentials: if the generator potential is big and long lasting, there is a repetitive discharge of the neuron, as the axon hillock is the only placed with the voltage-gated channels (if you keep stimulating its membrane above the threshold you will generate a repetitive discharge. As the graded potentials loose current across minimal distances (the λ), the architecture is very important because the location of the synapse determines its weight of power in creating or inhibiting the final action potential: so, if a synapse is located closer to the axon hillock, it will influence the final action potential more than another one located further away. 10 However, all the synapses, even at a large distance from the axon hillock, are important because they all contribute to the modulation of excitability. The summation of signals is not always linear because other than the spatial differences, the time differences of synaptic transmissions need to be considered. In fact, the first post-synaptic potential can generate intracellular changes that facilitate or inhibit the transmission of the next ones, (for example, by detaching less or more vesicles) contributing to an acute, short-term modulation. Finally, synapses can be modulated also for long term, for example through the expression of which and how many receptors, by changing their sensitivity, and the amount of neurotransmitter released or reabsorbed. This long-term modulation confers plasticity to the nervous system, used in complex processes such as learning. Case A: there’s an excitatory post-synaptic potential. The Glutamate synapse is made by a channel opening. However, the channel is not only for one ion but for two: Na+ and K+. In this case, the current of Na+ is going to be higher with respect to the current of K+ because the electrochemical driving force for Na+ is higher than the electrochemical driving force for K+. This is because the electrochemical gradient force is the distance between the equilibrium potential of the ion and the actual potential of the membrane. At -60, the equilibrium potential for Na+ is +30 so (+30 -60 -> 90 of gradient). For K+ it’ll be -60, -90 -> 30. So, if the conductance is always the same, the current will be much higher for Na+. 11 Na is powerful to bring the membrane towards more positive values, in fact it reaches the threshold. It will always reach the threshold because the reversal potential is the value of the membrane potential where the two currents will equalize each other. Even though sodium is stronger, by going towards positive values, the driving force on the sodium will be less strong and the driving force on the potassium will be stronger. What happens is that Na+ “wins”, it brings towards more positive values which means increasing the distance of the K+ to its equilibrium potential. So, by going positive, the gradient for potassium will be stronger and at a certain point there’ll be a reversal potential. The reversal potential is 0 mV (because the two forces equalize in this situation) and so is more positive than the action potential threshold -40 mV. In other words, by switching on this device, there’ll always be a depolarization as a net result and it’ll always be able to reach the threshold which is always more negative than the reversal potential. Even though it goes on forever and reach the reversal potential, it has already reached the threshold so for sure this is a depolarizing excitatory of synaptic potential. This is an excitatory synapse because whatever happens, the reversal potential which is the potential that changes the sign in the system is always way above the threshold values. There’ll never be a risk to have a reversal potential when it’s not at threshold. That’s why this synapse can be considered excitatory. Case B: situation opposite to A. GABA is opening chloride channels. The resting potential of chloride is -70 so what happens is that the current will go towards more negative values and since the reversal potential is more negative than the threshold, it’s inhibitory. All the synapses that are hyperpolarizing currents are inhibitory because in all cases the reversal potential will be more negative than the threshold. Case C: unusual case where the reversal potential is at -50 which means that the synapse will never depolarize more than -50. The reversal potential is more negative than the threshold even while depolarizing. This is something that usually happens involving ions that are activated not at resting membrane potential but at different membrane potentials, so the starting point is already affected by the value of the membrane potential. General rule of postsynaptic action: If the reversal potential is more positive than threshold, excitation results. If the reversal potential is more negative than threshold inhibition occurs. If it’s not possible to reach the threshold because the structure is preventing it, it might be considered as inhibitory because it prevents to reach the potential. f. The neurotransmitters production and release 12 The neurotransmitter is synthesized in the soma, passes through the axon and towards the synaptic ending. Small molecules can go directly and reach the vesicle at the synaptic end, big molecules reach it through vesicles along the axon. The duration of the post-synaptic event depends on the clearance of the neurotransmitter. The neurotransmitter is a molecule, so the interaction with the post synaptic receptor is a reaction. Here the probability to bump into the receptor is very high. After the release of the neurotransmitter, the highest probability phenomenon is the reaction between the neurotransmitter and the receptor. However, this reaction is not never-ending: as long as there are a lot of reactants, there’ll be a lot of binding, but as sson as the reactants decrease, the probability to bind the receptor decreases and the probability to be detached from the receptor increases. 13 This is because the synaptic structure (in this case) is not sealed. In that precise representation*, the membranes are very near each other but there’re ways to escape at one side (imagine it tridimensional) into the fluid. There’s the possibility to be reuptaken by the glial cells. In some cases, there are also enzymes expressed in the synaptic devices which can change the neurotransmitter, inactivating or separating it in different components. Summary of events: 1) Release of a big amounts of molecules (neurotransmitters) 2) Huge probability to bind a receptor 3) Channel opening 4) Flowout of neurotransmitters** 5) Decrease of the probability to have open channels **Some of them will go out of the cleft, some will be uptaken by the glial cells, some will be uptaken by the same cell that released them (autocrine uptake) and some can be inactivated by the enzymes. In the end, there will be a complete detachment of the neurotransmitter and a complete clearance of it. This takes a certain time. It’s possible to increase or decrease the duration by increasing or decreasing the stimulation of the synapse. Some post synaptic events are longer whereas some shorter depending on the velocity of the clearance. g. Geometrical connectivity The axo-dendritic connectivity is the most frequent event where the axon goes on the dendrites: the axon goes on spines, which are membrane structures specialized to get in contact with incoming axons. Sometimes they get in contact but there’s no exchange in terms of synapse. There’re also axo-somatic and the axo-axonic types. This last one is very rare. 14 e.g., in this case there’s an ultra specialization. It doesn’t look that the post synaptic is the target but actually the pre-synaptic finds a way to the specific. This happens when there’s an axon with multiple synapses where the precision is in the receiving cell. This usually happens when the axon must diverge to different cells. There’re different types of possible interactions: in some cases there are the interaction spines and also the synapse, whereas in some other cases there are the interaction spines but not the synapse. Architecture of synaptic contacts is important for integration of incoming messages 15 A neuron (such as the one in the pic above) can have different receiving synapses in different places and with different effects. The neuron must integrate all the synapses all the time. Since the dendrites and the soma are the recipient parts of the neuron, their job is to receive and collect the synapses which are inducing graded potentials. All the post synaptic events go towards the axon hillock, which receives all the synapses and averages the information. Basically, the axon hillock is a membrane continuously changing in potential because it’s continuously averaging the inputs (the graded potentials) coming from everywhere in the cell in time and amount. The very important thing in the brain it’s not just the discharge of the neurons but the probability of discharge. The axons working together are connected in such a way they modulate the excitability one of the other. So, if a neuron receives from different other areas, the power of each area will be different depending on the power of synapses on the receiving neurons. In some cases it’s essential keeping a more or less excited neuron, which is more or less ready to respond to others: when other neurons fire, the structure can be more or less ready to go. A neuron therefore is not active only when it fires. It’s just making the others more or less likely to fire. So, architecture matters because graded potentials are conducted with dissipation. e.g., the graded potential produces a depolarization that goes above threshold. The synapse is very far from the axon hillock, so by the time the impulse arrives there, it’s not able to fire anymore but it is still able to depolarize the membrane. In order to make this neuron fire, the synapse should be much more powerful. h. Post synaptic potential conduction Graded potentials are characterised by a decremental conduction. To fire, the axon hillock needs to reach the threshold despite dissipation of graded potential. 16 In the pic there are 2 different types of circuit. There’s a sensory neuron incoming which makes contact with: - an interneuron that ends up on flexor MN - an extensor MN. By looking at the action potential it’s possible to see which one is stimulated (interneuron). It’s also possible to see an inhibitory post-synaptic potential on the flexor MN and an excitatory one on the extensor MN. This shows that the post synaptic potential is a graded potential: the amplitude of the post synaptic potential is related to the strength of the pre-synaptic; so, if the synaptic input is strong, the post synaptic potential will be increased in amplitude. In the pic is shown that in the second case, the time is longer because there are two synapses needing to fire. Fast or slow adaptation The time course of the stimulus is regulated by the properties of the membrane of the neuron, responsible for the neural adaptation: fast or slow adaptation. Adaptation is the phenomenon when the neuron decreases the frequency of discharge, when receiving a constant stimulus. It is due to the presence of an additional population of potassium, adding to the preexisting K and Na channels that operate to form a single action potential This additional population of potassium channels is responsible for the additional hyperpolarization of the membrane, able to slow down the frequency of action potentials triggered, by increasing the difficulty of the membrane potential to reach the threshold. The steady state frequency of discharge is linearly correlated with the intensity of the current. Two populations of neurons therefore appear: slow-adapting neurons and fast-adapting neurons. 17 Slow adapting neurons get excited during the whole period of stimulation whereas fast adapting neurons are exited at the level of changes of stimulus. Two families of receptors exist to correspond to these neurons: - tonic, metabotropic receptors slow adapting neurons - phasic, ionotropic fast adapting neurons. The amount of NT released influences the amplitude of the post synaptic membrane potential (a graded potential), and is therefore influenced by the frequency of discharge of the Pre synaptic membrane. Binding of neurotransmitters may influence the postsynaptic neuron in either an excitation or inhibitory way changing the membrane potential towards or far from the threshold respectively. In any case the graded potential is spread towards the axon hillock by decremental conduction, hence even if the original intensity would have been able to elicit an action potential, maybe when it finally reaches the axon hillock it will not be able to cause a sufficient depolarization that can trigger an action potential. This occurs due to dissipation. The release of NT, coded by presynaptic membrane potential, allows the formation of either rapid, short-acting and fast post-synaptic potentials. The chemically graded ion channels open and allow either: - the entry of Na+, for the formation of an EPSP - the exit of K+ or the entry of Cl- for the formation of an ISPS The release of NT can also allow the formation slow acting post-synaptic potentials via G-p° coupled receptors. The activation of these receptors causes the activation of a second messenger pathway that has two consequences. The first is the alteration of the opening state of ion channels. The ion channels will can open causing: - the entry of Na+, for the formation of an EPSP - the exit of K+ or the entry of Cl- for the formation of an ISPS The ions can also close causing - the restriction of the Na+ for the formation of an EPSP - The restriction of the K+ for the formation of an IPSP The second consequence of the actiavtino of a secondary messenger pathway is that it will modify pre-existing p° and regulate the synthesis of new p°. This allow the coordinating of an IC response. 18 The integration of all the post-synaptic graded potentials is done at the level of the axon hillock. The axon hillock is a trigger zone who will fire action potentials if it passes a certain threshold of excitation. The graded potential is spread across the neuron in a decremental conduction, rendering it liable to dissipation. IPSP and EPSP are therefore subject to dissipation. This also causes the location of the synapse to be crucial in the integration of its respective post-synaptic potential. The integration of the IPSP and EPSP at the level of axon hillock can bring the neuron to threshold by either temporal or spatial summation. As soon as the threshold is reached, an action potential is generated. The summation is total as soon as all the receptors are saturated. The temporal summation is the summation of a single neuron over time whereas the spatial summation is the summation of multiple neurons at any given time. From graded potential to action potential: - For neurons, the graded potential are the psot-synaptic potentials and the encoder is the axon hillock. - For sensory neurons, the graded potential is ths receptor potential and the encoder is the first portion of the axon expressing voltage depended Na+ and K+ channels. i. Current to frequency coding Increasing the intensity of the current reduces the refractory period until the point of absolute refractoriness. 19 Parallelly, increasing the intensity causes the firing of more AP. The combination of EPSP and IPSP allows the generation of ≠ patterns of discharge. The relationship between the frequency of discharge and the current intensity depends on the neuron type. - corticospinal neurons discharge with a frequency between 40 Hz to 230 Hz in a range of intensity from 4mA to 25mA. They can therefore be characterized current-to-frequency specialized and very sensitive. - spinocerebellar neurons between 40 Hz to 260 Hz in a rang on intensity from 4mA to 25mA. They are sensitive small changes in current, which is justified as they code for fast changing phenomenas. - Motor neurons, alter their frequency of discharge from 5 Hz to 100 Hz, in response to higher variations in electrical current, from 5mA to 50mA, They are much less sensitive to variations in intensity. 20 j. Paired-pulse facilitation and depression Paired-pulse facilitation, is a phenomenon in which postsynaptic potentials (EPPs, EPSPs or IPSPs) evoked by an impulse are increased when that impulse closely follows a prior impulse. PPF is a form of short-term synaptic plasticity, changing the power of the synapse. Voltage-gated Ca2+ channels are located within the presynaptic terminal, able to open, when an action potential invades the presynaptic membrane. A higher concentration of Ca2+ enables synaptic vesicles to fuse to the presynaptic membrane and release their contents (neurotransmitters) into the synaptic cleft to ultimately contact receptors in the postsynaptic membrane. The amount of neurotransmitter released is correlated with the amount of Ca2+ influx. Therefore, short-term facilitation (STF) results from a build up of Ca2+ within the presynaptic terminal when action potentials propagate close together in time Short-term depression operates in the opposite direction of facilitation, by inactivating presynaptic Ca2+ channels after repeated action potentials. k. Synaptic inhibition Synaptic inhibition can occur un two ways: a pre-synaptic inhibition or a post-synaptic inhibition. - In a presynaptic inhibition, a modulatory neuron synapses on one collateral of the presynaptic neuron, selectively inhibiting one target. - In post synaptic inhibition, all targets will be inhibited equally. 21 7. The targets of neuronal communication The CNS regulates the activity of ≠ effectors by initiating AP in the cell bodies of efferent neurons whose axons terminate on these organs. The ≠ effectors are: - Cardiac muscle - Smoot muscle - Most exocrine glands - Some endocrine glands - Adipose tissue. These effectors are innervated by the autonomic nervous system, the involuntary branch of the peripheral efferent division. Postganglionic fibers do not end in a single terminal swelling like a synaptic knob: the autonomic fibers have many swellings called varicosities. These varicosities allow the simultaneous release of NT over a large area of the innervated organ instead than in a specific area. Skeletal muscle is innervated by the somatic nervous system, the branch of the efferent division subject to voluntary control. The somatic nervous fibers end on the skeletal muscle with the classical synaptic knob: a restricted area is stimulated. 8. Clinical case – NT hypothesis of depression. In a synapse a single NT may target different receptor types on the post-synaptic membrane. Some PSM receptor types may not be expressed constantly, but they are induced by the release of certain NT. This is typically found in synapses in the Hippocampus). This phenomenon can result in a long term empowerment of the synapse, as an increase in the receptors number can lead in a stronger cell response, and the generation of new synapses. Via this hardware, long term modulation of the synapse can be induced (a change in shape such as a widening of the synapse) and new synapses can be created. These long term modulation can refer to - Potentiation (long lasting increase in in signal transmission between the 2 neurons) - Depression (a long lasting decrease in signal transmission). This is the basis of synaptic plasticity(e.g. for memory processing in the hippocampus) 22