Resting Membrane Potential - PHYS 2 PDF
Document Details
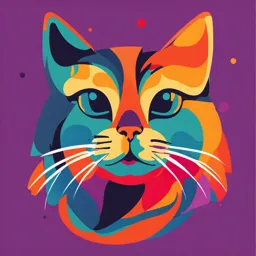
Uploaded by SplendidNovaculite8819
Università degli Studi di Milano Bicocca
Tags
Summary
This document covers resting membrane potential, explaining its significance in excitable cells. It details the distribution of ions across the membrane and describes the factors influencing membrane potential, such as ion channels and transporters. The document also touches upon the control mechanisms of gated channels and their activation and deactivation by ions or molecules.
Full Transcript
PHYS 2 - Membrane excitability: Resting membrane potential An excitable cell is a cell that can actively change their membrane. The nervous system uses electricity to communicate. All cells possess a membrane potential. Excitable cells such as cardiac muscle cells, neurons, … are cells that are abl...
PHYS 2 - Membrane excitability: Resting membrane potential An excitable cell is a cell that can actively change their membrane. The nervous system uses electricity to communicate. All cells possess a membrane potential. Excitable cells such as cardiac muscle cells, neurons, … are cells that are able to alter their membrane potential on appropriate stimulation. The cell electrical potential is recorded by a voltage recorder containing two electrodes: a reference electrode IC and a microelectrode, penetrating the membrane with its tip located inside the cell. The resting membrane potential in cells is of -70mV. In spite of that, certain types of cells display a resting mb potential varying from -40 to -90mV. The membrane itself is not charged: the membrane potential refers to a separation of opposite charges across the membrane. The whole IC and EC volumes are electrically neutral. The difference lies in the distribution of charges. The distribution of charges near the membrane shows an asymmetric separation: there is a slight excess of positive charges EC near the MB and a slight excess of negative charges IC near the MB. The potential is measured in Volts. The magnitude of the potential depends on the number of opposite charges separated. The existence and persistence of the membrane potential is due to the unequal distribution of ions on each side of the membrane. 1. Distribution of ions in the ICF and ECF P: plasma I: interstitial fluid C: intracellular - HCO3-, Na+, Cl- are more present in the ECF than in the ICF. - Greater anions and proteins are present majority in the ICF and in the plasma but are absent in the interstitial fluid. Proteins in the blood cannot transverse the capillary bed. - K+ is present in majority in the ICF 2. Ions pass the plasma MB Ion transporters allow the passage of ions against the ion chemical gradient. These pumps generate an unequal distribution and keep an ion concentration gradient. Ion channels allow ions to diffuse down the concentration gradient. It can cause the selective permeability of certain ions. Pupps (active transport) transport ions against their concentration gradient. This channel (dark green) is permeable for the pink ion but not for Na+. The selectivity is determined in the channel. The distribution of charges inside the channel will allow certain charges to pass the membrane. The narrowness of the channel will allow the selectivity for the nature of the ion. A channel can be either for cations or for anions and depending on the configuration of the channel, it selective for one or more ions. Channels are either - Leak channels are always open. - Gated channels are either open or closed due to: by a clear determinant: o Variation in one portion of the channel o Variation of all the channel structure o Obstruction of the channel by a particle. The conductance is reciprocal of the electrical resistance. It measures how easily a given ion can flow across the membrane along the channel. It depends on the number of channels opened. For example, there are 10 channels for potassium and 1000000 for sodium: the Na+ has an enormous conductance and the K+ a good conductance. The opening control mechanisms of gated channels are: - Ligand dependant: any molecule or ion binding in a non-covalent bond NT - Phosphorylation dependant: the energy of binding a high energy phosphate group inside the cells can cause some ion channels to open or close (as activators of G-proteins in second messengers). - Voltage dependant: : open through the stimuli of change in voltage or membrane action potential with a charge separation across the membrane - Stretch dependant: transfer the energy to cytoskeleton in the plasma membrane to cause a mechanical change in conformation a. Ligand dependant channels: The ligand dependent channels can have Endogenous agonists, reversible agonists and irreversible agonists. Agonist means that the molecule binds and fosters the opening of the channel. - Endogenous agonists are the natural ligands responsible for stimulating that channel. - Reversible and irreversible agonists are ligands that resemble the endogenous one and that are designed to be agonists or antagonist for those channels. Some of those molecules bind to the ligand locus but cannot open the channel, so they keep the channel from opening since the endogenous ligand cannot bind the activation site anymore A channel, such as a voltage gated channels is controlled on both sides. 3. Which are the main driving forces moving ions across the membrane The passage of ions through the channels occurs by means of simple diffusion, following the concentration or chemical gradient. If there is a difference in concentration, there will be a Net movement. These movements, are not unidirectional, which means there is not only the movement towards the lesser concentration, there is always the return of some molecules towards the more concentration area, but is way smaller concentration. Thus happens until the difference in concentration gradient doesn’t exist anymore, forming the equilibrium. When the equilibrium is reached it doesn’t mean that the molecules stop moving, it just means that they move without a net movement (without altering the concentration in each side). It is a homogeneous movement. Simple diffusion across the membrane can happen only through the ion channels: a membrane is permeable to an ion inly is it possesses ion channels for that ion. If the membrane is impermeable to an ion, no diffusion can take place across the membrane, even though a concentration gradient may exist. The ion is a mass with a charge: as movement of a mass causes the movement of a charge. In the slide, if you look at the overall concentration of charges, Potassium (+) is very much concentrated inside and less concentrated outside. On the left, there is electro neutrality so no electrical gradient from inside to outside but there is a chemical gradient from inside to outside. As a result, no net movement. On the right side, the channel is only permeable to potassium (+) ions so (+) ions will move out. As soon as the positive ions move out, the negative charge is stuck inside. There will be a flux of positive charges, but unbalance of negative charges inside. As a result electroneutrality is lost In the beginning there is no electrical force, only the chemical force -as a result of chemical gradient. Potassium (+) goes out for a certain time until the movement stops. The negative charges inside will have a stronger call for positive charges, as more and more potassium (+) is left corresponding to time. To sum it up, at the beginning the chemical gradient wins. but as the time passes and more and more positive charges leave, negative charges inside start calling for the positive forces outside. It gets harder for potassium (+) to go out. Electrical gradient opposes the chemical gradient. The electrical gradient builds up with the excess if negative charges inside. The outflux of positive charges stops when the electrical gradient is as strong as the chemical gradient. The net movement is O. The K+ will go out with decreasing intensity until the net mouvment is O because the electrixal gradient is as strong as the chemical. The electrochemical eq induces a homogenous distribution. 4. Ions move across the membrane Movement of potassium out following the electrochemical gradient (both electrical and chemical forces). At the beginning there is the domination of chemical forces, but the equilibrium is reached when the electrical forces counterbalance the chemical ones. Injecting current, membrane potential changes because the electrical gradient is artificially increased. K+ will go from ICF to ECF, the equilibrium potential for K+ in our biological context is -90 millivolt Na+ does the exact opposite, it is more concentrated on the outside than inside. If we measure the equilibrium potential when we reach equilibrium. the value is +60 millivolt. Because inside the cell defines the sign, there will be a lot of positive charges inside because the sodium enters. Equation that allows to calculate exact equilibrium potential for a given ion is done by the NERNST EQUATION As conventionally, EII (EC side) is null, Knowing the constant values and the chemical gradient of a given ion we actually can calculate its electrochemical equilibrium potential by using NERNST EQUATION 5. Resting membrane potential The resting membrane potential is equal -70mV. It does not correspond to any eq potential of the ions. This is due to 3 reasons: - Membrane is permeable to more than 1 ion - Membrane is not equally permeable to ≠ ions - Membrane contains active transports maintaining ICF and ECF ion compositions in a stable state. Na/K pumps, important for energy consumption. They transport Na and K in an asymmetric way against their gradient. Pumps do not generate the distribution, they just maintain it. Is there a determining ion ? K+ ions: Although K+ is more concentrated inside the cell, it tends to diffuse outside the cell according to - The concentration gradient - The high permeability of the cell membrane. However the K+ outflux is limited by - Attraction between K+ and intracellular proteins. - Repulsion between K+ and Na+ outside cell membrane. - K+ -Na+ pump which derive K+ inside the cell. Na+ ions: Although Na+ is more concentrated outside the cell , it tends to diffuse inside the cell according to: - The concentration gradient. - The electrical gradient. However the Na+ influx is limited by : - the low permeability of the cell membrane to Na+. - K+ -Na+ pump which pumps Na+ outside the cell. The intensity of the current of an ion can be measured, given by the electrochemical driving force (Fe) multiplied by the conductance. The electrochemical driving force is another way to describe electrochemical gradients. Vm = actual value of membrane potential in time, Ek = equilibrium potential for potassium Equilibrium potential of sodium is +60, the difference is: (-70 - (+60)) = 130. Therefore, the conductance of sodium is higher compared to potassium. The membrane will allow potassium to go out until it reaches -90, at the end net movement will stop and the membrane potential will be at equilibrium potential. Sodium has a huge driving force but it has only one channel, so the intensity of the current is tiny (low conductance) → negativity will be reduced, amount of the difference in the potential will be reduced. The potential depolarizes, it goes towards more positive values. Potassium starts flowing outside, the tiny current of sodium gets completely counterbalanced by the tiny current of potassium at -70. The two reach a compromise. The unequal distribution generates the chemical gradient, the amount of the current depends on conductance Goldman equation calculates the membrane potential, it is basically Nernst equation considering all the ions flowing By using two electrodes, a membrane potential difference of -70mV can be measured but the value can vary between -40mV and -97mV among different cells. It depends on the movement of ions across the membrane through channels, the direction of movement is controlled by the electrochemical gradients of the different ions control. Main currents are caused by the entry of sodium ions and exit of potassium ions, whereas tiny currents are caused due to the flowing of ions through leak channels. The average of the summation of all the currents caused by ions (Na+, K+ and Cl-) moving across the membrane gives rise to the membrane potential and this value is not equal to the equilibrium potential of either of these ions: in the conditions of the interstitial fluid and intracellular fluid, the resting membrane potential is just a bit more positive than the equilibrium potential of K+ which is -97mV; however, the resting membrane potential value is far from the equilibrium potential of Na+ which is +60mV and it is very far from the equilibrium potential of Ca+2 which is +129mV. The resting membrane potential is determined by the ion currents flowing through the membrane. The currents are caused due to the membrane’s selective permeability and the conductances of different ions. The conductance is higher for K+ and Cl- and lower for Na+. The movement of the ions are determined by their conductance as well as their electrochemical driving force. It is called electrochemical because the ions are masses with a charge so both the mass (chemical) and charge(electrical) must be considered. Na-K pump on membrane potential The membrane potential is not determined by the Na-K ion pumps: the pumps are important in maintaining the unequal distribution of Na+ and K- inside and outside the cell. In a membrane without pumps, the ion currents will continuously move through the membrane and they will only perturbate the fluid near the membrane both inside and outside. However, if this goes on for a long time the concentration will change and this will lead to a change in gradient. The change in gradient means that the Nernst equation changes so the equilibrium potential will also change and this will lead to a change in the driving force: consequently, the amount of current changes and the membrane potential will also change. The job of the ion pumps are to maintain the gradient by transporting ions against their gradient using energy. These pumps are asymmetric as 3 Na+ are moved out while only 2 K+ are moved in. Pumps are more efficient when substrate concentration is higher and this is because chemical reactions occur at the level of the pump: the higher is the substrate concentration, the higher will be the probability of chemical reactions occurring so the higher will be the rate of reaction. The efflux of Na+,, which is a measure of the efficiency of the pump, can be reduced by the removal of external K+ and also by metabolic inhibitors. The availability of energy is the second determinant of the pump efficiency. The Na+ efflux is used to measure efficiency rather than K+ influx because it is easier to measure what’s outside the cell than what is inside. In summary, the membrane conducts ions very poorly as there aren’t many leak channels and this allows the separation of ionic species which results in a difference in potential between the outside and the inside of the membrane. The magnitude of the resting potential is determined by the selective permeability/conductance of the membrane to ionic species; it can be quantified by considering both the diffusive (chemical) and electrophoretic (electrical) properties of each ion passing through the membrane. Electrical equivalent circuit In order to understand the time dependence and individual contributions of ionic species to the membrane potential in excitable cells, it is convenient to use an electrical equivalent circuit: the resistors can be considered the electrical equivalent of ion channels as the currents moves through the resistors in a circuit; the capacitors can be considered as the electrical equivalent of the membrane and the batteries can be considered as the electrical equivalent of Na-K pumps. Resting potential It is called the resting potential because it is a default condition for every cell and is an attribute compared to another situation, the active potential. The active potential is expressed only in the excitable cells while all the other cells have only a resting potential. In the excitable cells, it is called the resting potential because it is the basic condition and starting from there, the excitable cells can change their membrane potential into different values. The clinical point: ion disorders Blood assessment is used during exams because it gives information about the interstitial fluid as they communicate with each other. The reason why blood is good for monitoring the ions concentration is because when the blood goes into the tissue, the ions in the blood are in equilibrium with the ions in the interstitial fluid. In some cases what you see in the blood is perfectly superimposable with the interstitial fluid The normal potassium level is 3.5-5mEq/L. - If a patient has moderate hypokalaemia, so reduced K+ levels: 2.5-3mEq/L, he will have disturbed functioning of the skeletal muscles causing muscular weakness, myalgia and muscle cramps and disturbed functioning of the smooth muscles causing constipation. - If the patient has severe hypokalemia, so less than 2.5mEq/L, he will have disturbed functioning of the skeletal muscles causing flaccid paralysis and disturbed nerve function causing hyporeflexia and respiratory depression. He will also have a disturbed heart function and this is because a change in gradient leads to a change in equilibrium potential which leads to a change in current. This causes an oscillation in membrane potential and if this change occurs in a cardiomyocyte or a pacemaker cell, they will be affected as these cells are excitable.