Buffer System PDF
Document Details
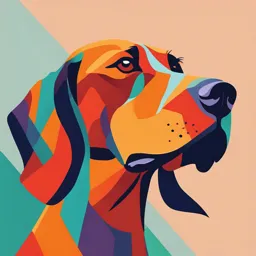
Uploaded by CohesiveParable7264
University of Oxford
Tags
Summary
This document provides an overview of buffer systems in the human body. It details different buffer systems like bicarbonate, phosphate, and protein and their mechanisms. The document also covers the importance of buffering in maintaining homeostasis and how various systems, such as respiratory and renal, help regulate blood pH.
Full Transcript
Buffer system Introduction: In the human body, maintaining a stable pH (7.35–7.45 in arterial blood) is important for cellular functions, enzymatic reactions, and overall physiological processes. Buffer systems are the body's first line of defense against sudden changes in pH, and they func...
Buffer system Introduction: In the human body, maintaining a stable pH (7.35–7.45 in arterial blood) is important for cellular functions, enzymatic reactions, and overall physiological processes. Buffer systems are the body's first line of defense against sudden changes in pH, and they function to neutralize excess acids or bases. Definition of a Buffer A buffer solution is an aqueous solution that resists changes in pH upon the addition of an acid or a base. It consists of a weak acid and its conjugate base or a weak base and its conjugate acid. They work by neutralizing added acids or bases, helping maintain a relatively constant pH. Major Buffer Systems in the Human Body: 1. Bicarbonate Buffer System: Components: Bicarbonate ion (HCO₃⁻) and carbonic acid (H₂CO₃). Location: Extracellular fluid (blood plasma). Mechanism When excess H⁺ ions are present, bicarbonate ions combine with them to form carbonic acid, which then breaks down into CO₂ and H₂O, releasing CO₂ through respiration. If the pH rises, carbonic acid can release H⁺ ions, lowering the pH. Importance: This is the primary buffer in the blood, and it works closely with respiratory and renal systems to manage pH changes. 2.Phosphate Buffer System: Components: Dihydrogen phosphate (H₂PO₄⁻) and hydrogen phosphate (HPO₄²⁻). Location: Primarily in intracellular fluid and kidney tubules. Mechanism: When pH decreases, HPO₄²⁻ can bind with H⁺ ions to form H₂PO₄⁻. When pH increases, H₂PO₄⁻ can donate H⁺ ions, stabilizing pH. These two ions are in equilibrium with each other as indicated by the chemical equation below. _ H2PO4 - H + HPO4 + 2 The total concentration of this buffer in both plasma and erythrocytes is less than that of other major buffer systems. It’s concentration in the plasma is very low.( It accounts for only 5% of the non- bicarbonate buffer value of plasma). Importance: The buffer system is crucial in the kidneys, where it assists in the excretion of hydrogen ions. 3.Protein Buffer System Nearly all proteins can function as buffers. Proteins are made up of amino acids, which contain positively charged amino groups and negatively charged carboxyl groups. Components: Amino acids and proteins, such as hemoglobin in red blood cells. Location: Both intracellular and extracellular fluids Mechanism: Proteins have amino groups that can accept H⁺ ions and carboxyl groups that can release H⁺ ions, depending on pH. In Low pH (Acidic) Conditions Low pH means a high concentration of H⁺ ions in the environment. The amino group (-NH₂) can accept an additional H⁺ ion, becoming positively charged as -NH₃⁺. This helps reduce excess H⁺ ions in the environment, acting as a buffer to prevent further decreases in pH. The carboxyl group (-COOH), already protonated, does not release H⁺ at low pH because it is less likely to lose H⁺ in an acidic environment. Overall effect: The amino group accepts H⁺, mitigating some of the acidic effect and contributing to pH stabilization. In High pH (Basic) Conditions High pH indicates a low concentration of H⁺ ions, creating a more basic environment. The carboxyl group (-COOH) tends to release an H⁺ ion, becoming negatively charged as -COO⁻. This release of H⁺ adds protons back into the solution, helping to counteract the increase in pH. The amino group (-NH₂), which is deprotonated at high pH, remains uncharged and does not accept H+ because of the low availability of H+ ions. Overall effect: The carboxyl group releases H⁺, helping to stabilize pH by countering the basic environment. Structures of protein showing amino and carboxylic groups 4. Hemoglobin Buffer System: Location: Red blood cells. Mechanism: Hemoglobin can bind H⁺ ions, especially after oxygen has been released to tissues, helping to buffer changes in pH. This system also helps transport CO₂ from tissues to the lungs for exhalation. Importance: Hemoglobin plays a key role in maintaining blood pH, especially in the venous blood, where CO₂ levels are high. Buffer Systems and Acid-Base Disorders Each buffer system works in conjunction with respiratory and renal systems to compensate for acid-base imbalances: 1.Respiratory System Compensation(Lungs): Rapidly regulates blood pH by adjusting CO₂ levels through breathing. Example: In response to acidosis(the pH is low). The respiratory rate increases, removing CO₂ and raising pH. 2.Renal System Compensation(Kidneys): Adjusts blood pH more slowly by controlling excretion of H⁺ and reabsorption of HCO₃⁻. Example: In response to alkalosis, the kidneys retain H⁺ and excrete more bicarbonate. Interrelationship of hemoglobin in the red blood cells and the H + from the bicarbonate buffering system. Isohydric and Chloride Shift: Introduction: In the human body, the regulation of acid-base balance and efficient gas exchange are essential processes for maintaining homeostasis. Two important mechanisms that contribute to this balance, especially in red blood cells are; isohydric shift chloride shift. The two processes enable the transport of carbon dioxide (CO₂) from tissues to the lungs, buffering pH changes in the blood along the way. Isohydric Shift: The isohydric shift refers to the process in which hydrogen ions (H⁺) released from carbonic acid are buffered by hemoglobin without altering the blood pH. Mechanism: 1. Carbon Dioxide Entry: In tissues,CO₂ diffuses into red blood cells (RBCs). 2. Formation of Carbonic Acid: CO₂ combines with water (H₂O) in RBCs, catalyzed by the enzyme carbonic anhydrase, to form carbonic acid (H₂CO₃). 3. Dissociation of Carbonic Acid: Carbonic acid quickly dissociates into bicarbonate (HCO₃⁻) and hydrogen ions (H⁺). 4. Hemoglobin Binding of H⁺:The H⁺ ions bind to deoxygenated hemoglobin (Hb), which acts as a buffer. Hemoglobin is a good buffer because, after releasing oxygen, it has a higher affinity for H⁺ ions. This process prevents the free H⁺ ions from significantly lowering blood pH. 5.Isohydric Nature: This buffering action by hemoglobin occurs without changing the overall pH of the blood, hence the term "isohydric," meaning “same hydrogen” (no net change in free H⁺ concentration in blood). Chloride Shift (Hamburger Phenomenon): The chloride shift is the exchange of bicarbonate (HCO₃⁻) and chloride ions (Cl⁻) across the red blood cell membrane, allowing for the transport of bicarbonate out of RBCs and into the plasma. Mechanism: 1.Formation of Bicarbonate: In RBCs, as CO₂ is converted into HCO₃⁻ (bicarbonate) and H⁺ ions, the bicarbonate concentration within RBCs rises. 2. Bicarbonate Diffusion: Bicarbonate ions move out of the RBCs into the plasma to maintain electrochemical balance. 3. Chloride Influx: As bicarbonate ions exit the RBC, chloride ions (Cl⁻) from plasma enter the RBCs to maintain electrical neutrality. 4. Reverse in Lungs: In the lungs, this process reverses. Bicarbonate re-enters RBCs, combines with H⁺ to form carbonic acid, which then converts back to CO₂ for exhalation. Chloride ions exit the RBCs as bicarbonate re-enters. Fig.2. Chloride Shift: The entering of chloride ions(Cl+) into the red blood cell from the plasma. Carbon dioxide is carried into the cell from respiring tissues. Compensatory Mechanisms Compensatory mechanisms are physiological processes that the body initiates to restore homeostasis when it encounters stressors, diseases, or disruptions in normal function. Importance in Pathophysiology: These mechanisms help to sustain essential functions, especially under conditions of acute or chronic stress. However, prolonged compensation can lead to pathologies if the underlying cause isn’t addressed. Example in diabetes Mellitus: Prolonged compensatory hyperinsulinemia in insulin resistance eventually leads to beta-cell exhaustion and hyperglycemia. Types of Compensatory Mechanisms: 1.Renal (Kidney Function). Example: Regulation of acid-base balance through bicarbonate reabsorption or hydrogen ion excretion. Mechanism: Kidneys compensate for respiratory acidosis or alkalosis by adjusting bicarbonate levels. 2. Respiratory System(Lung function) Respiratory Compensation: Adjusts breathing rate and depth to maintain blood gas levels(CO2 ,O2). 3. Hormonal: Example: Renin-Angiotensin-Aldosterone System (RAAS). Mechanism: Activates in response to low blood pressure or sodium depletion, leading to water and sodium retention to increase blood volume.