BS332 Lecture 2 2024 PDF
Document Details
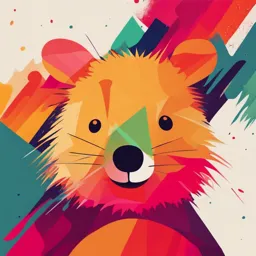
Uploaded by RockStarLobster8287
University of Essex
Vass Bavro
Tags
Summary
This document is lecture notes about membrane fluidity, detergents, lipid rafts, and membrane proteins. It discusses phases, phase diagrams, and how temperature affects membrane structure and function. It also includes relevant diagrams to illustrate concepts.
Full Transcript
BS332 – Lecture 2 Fluidity of the membrane and lipid phases. Detergents and Membrane Stability. + Time permitting – Lipid Rafts & structural studies of membrane proteins Prof Vass Bavro v...
BS332 – Lecture 2 Fluidity of the membrane and lipid phases. Detergents and Membrane Stability. + Time permitting – Lipid Rafts & structural studies of membrane proteins Prof Vass Bavro [email protected] = Optional material! Membrane Fluidity Phases and phase diagrams Membrane Fluidity Lipids and proteins diffuse laterally in the bilayer by Brownian movement. Lateral diffusion can be studied using fluorescence microscopy using a technique called Fluorescence Recovery After Photobleaching (FRAP). Lipids are fluorescently labelled and then bleached with an intense laser beam The rate of recovery of fluorescence in that region can then be monitored. ` This diffusion can be very rapid – a molecule in the outer leaflet on an erythrocyte can circumnavigate the entire cell in a few seconds. A typical phospholipid travels at around 1 m per second. Given that a typical bacterial cell is about 2-8 m in length, that allows it to sample the whole membrane within a single minute. Phases and phase diagrams Phase is a region of space (a thermodynamic system), throughout which all physical properties of a material are essentially uniform. A thermodynamic system is described by a number of thermodynamic parameters (e.g. temperature, volume, pressure) which are not necessarily independent. The number of parameters needed to describe the system is the dimension of the state space of the system (D). State space is the set of all possible configurations of the system. Thermodynamic properties can be divided into two general classes: intensive and extensive. An intensive property is independent of the size of the system. Temperature, pressure, specific volume, chemical potential and density are examples of intensive properties. Mass and total volume are examples of extensive properties. So are energy, Gibbs energy (G), enthalpy (H), entropy (S) etc… but more of these later with Dima! Phases and phase diagrams For a given system composition, only certain phases are possible at a given pressure (P) and temperature (T). The number and type of phases is hard to predict and is usually experimentally determined. These results can be plotted in phase diagrams. For a single component system (such as H2O below), at first approximation, the possible phases are determined solely by pressure and temperature. So, we can draw a P/T phase diagram. The lines (aka phase equilibrium curves) show points where two or more phases can co-exist in equilibrium. At temperatures and pressures away from the markings, there will be only one phase at equilibrium. Normal freezing point Normal boiling point A critical point (or critical state) is the end point of a phase equilibrium curve. The most prominent example is the liquid-vapor critical point, the end point of the a P/T curve that designates conditions under which a liquid and its vapor can coexist. Above that is a so-called “supercritical fluid” state. Lamellar phases FUNCTIONAL MEMBRANE! Lamellar phase refers generally to packing of polar-headed long chain nonpolar-tail molecules (such as phospholipids) in an environment of bulk polar liquid, as sheets of bilayers separated by bulk liquid. Liquid or fluid lamellar (Lα) also known as liquid disordered or liquid crystalline is the closest to functional biomembrane state under physiological conditions, but gel-phases also exists. Gel phases are tightly packed, mobility is Commonly observed crystalline and liquid crystalline lamellar phases in biological lipids. reduced (no lateral diffusion) and they are generally impermeable. Kulkarni, C. V. (2012). Lipid crystallization: from self-assembly to hierarchical and biological ordering. Nanoscale, 2012, 4, 5779–5791 Lamellar phases – temperature transition Gel-to-fluid phase transition is temperature controlled! Transition phase Lamellar fluid crystal phase is an example of a mesophase – a state intermediate between crystal and liquid. Bilayer composition affects diffusion and phase transition temperature More contacts between As temperature is decreased bilayer changes from liquid (fluid) molecules = need more Kinky chains – fewer disordered Lα state to a crystalline state (or gel) Lc or Lβ– PHASE contacts = more liquid energy (temperature) TRANSITION (remember the butter!) to disrupt! The phase transition temperature is lower when the hydrocarbon chains are shorter as this reduces the interaction between lipids. Double-bonds produce kinks making the chains more difficult to pack together and consequently this helps membrane stay fluid as temperature is decreased. Membranes containing (unsaturated) or short lipid chains find it more difficult to undergo a phase transition and form a gel state – increasing the mobility and fluidity. Lipid composition of the membrane can be modified depending on the temperature of the environment – this allows adaptation of the organism to a given temperature. E.g., at low temperatures fatty acids with more cis-double bonds are synthesised and incorporated into the membranes – maintaining a high level of fluidity at low temperatures. The role of cholesterol on membrane fluidity Another strategy for modifying membrane fluidity in eukaryotic plasma membranes is the incorporation of cholesterol. Up to 1 cholesterol molecule per phospholipid in eukaryotes Cholesterol orientates with the polar head group close to the polar groups on the phospholipid molecules – the rigid sterol ring interacts with the regions of the hydrocarbon chains that are closest to the polar head groups Cholesterol tightens the packing of lipids, increases the rigidity of the membrane, decreasing permeability and, at high concentrations, inhibiting Figure 10-4&5 Molecular Biology of the Cell (© Garland Science 2008) phase-transitions into crystalline state. Lamellar phases – temperature transition & the cholesterol effect Cholesterol can act as a buffer for membrane More solid fluidity. Fluid like At low temperatures it disrupts interactions between lipids increasing fluidity. Solid-like At high temperatures it helps to maintain More fluid interactions between lipids decreasing the fluidity. Cholesterol is an “ordering agent” which moves both the Lβ (gel) and the Lα (disordered) phases into a Lo = liquid ordered phase Lamellar phases – temperature transition Gel-to-fluid phase transition is temperature controlled! Tc – transition temp. Usable temperature range of pure phospholipid membranes is very limited! liquid crystalline (Lα)and gel (Lβ) lamellar phases in biological lipids. Extended useful functional range Cholesterol eliminates the transition phase of the lipid bilayer. Cholesterol also increases the bilayer permeability in the gel phase and decreases it in the fluid phase. Monteiro et al., DOI: 10.1098/rsif.2014.0459 Membrane Fluidity - summary Membrane fluidity has to be tightly regulated Useful functional range Artificially stiffening the membrane causes membrane transporters and enzymes to cease functioning The fluidity of a lipid bilayer depends on its composition and temperature In a synthetic bilayer composed of a single phospholipid type, as the temperature is lowered the bilayer transitions from a liquid state to a two-dimensional rigid crystalline (or gel) state. This change in state is known as the phase Monteiro et al., DOI: 10.1098/rsif.2014.0459 transition. Gel-to-fluid phase transition is temperature controlled! Tc – transition temp. Cholesterol is a modifier that extends the useful functional range of the membrane! Extended useful functional range Lipid phase transition - mesophases So far, we only discussed the lipids on their own! But what if we mix them with water? …enter monoolein! Biophys J. Vol83 Dec 2002 3393–3407 Mesophases in pink shading Phase transition diagram for monoolein in water Lipid mesophases One of the best characterized lamellar phases is the now familiar liquid disordered phase or liquid crystal (Lα) (A) Remember! The Lα lipid phase most closely resembles a functional lipid bilayer. The normal hexagonal (HI) (B) and the inverse hexagonal (HII) (C) lipid phases are non-lamellar lipid phases in which the lipids form tubular structures. In the HI phase, the center of the cylinder is made up of the hydrophobic tails, while in the HII phase, the hydrophobic tails face outwards and the core of the tube is comprised of the hydrophilic head groups Nanoscale, 2012, 4, 5779–5791 Lipid phases – cubic phases Cubic phases are good ordered approximation for membrane environments PNAS Dec 1996, 93 (25) 14532-14535 Non-lamellar lipid self-assemblies: (a) Hexagonal (H2), (b) Fd3m – micellar cubic phase, and (b) (c) Ia3d, Pn3m and Im3m cubic phases are based on mathematical minimal surfaces G, D and P respectively; thin arrows indicate water channels meeting at different angles. (d) Sponge phase – made of disordered bilayer, and (e) disk-like lipid micelles called bicelles Detergents Extended bilayers are energetically unfavourable Why is a planar bilayer energetically unfavourable? What structure would be more favourable? Forming sealed compartments underpins the generation of cells and subcellular compartments. Why Sphere? => smallest surface to volume ratio! Figure 10-8 Molecular Biology of the Cell (© Garland Science 2008) Main types of detergents Anionic (negative) Zwitterionic (dipolar ion) = net charge is 0 SDS -Sodium dodecyl sulfate aka sodium lauryl sulfate (SLS) Bile acid (anionic) Sodium stearate – Soap! Na-salt of a fatty acid Non-ionic CHAPS or 3-[(3-CHolamidopropyl) dimethylAmmonio]-1- PropaneSulfonate. Triton X-100 (Polyethylene glycol p-(1,1,3,3-tetramethylbutyl)-phenyl ether) Maltoside Octyl β-D-galactopyranoside HEGA11 (Undecanoyl-N-Hydroxyethylglucamide) Detergents and CMC. VERY IMPORTANT! Critical micelle concentration (CMC) is defined as the concentration of detergents above which micelles are spontaneously formed. Adding detergent to the solution above the CMC concentration results in it being associated entirely with micelles, and there is no increase in the concentration of free detergent molecules The CMC is important in biology because at concentrations above it the detergents form complexes with lipophilic (membrane) proteins. Usually at least 2x CMC is needed to keep a membrane protein “happy” in a detergent solution, but much higher concentration is needed for solubilisation of membrane proteins – that is, their extraction out of the membrane and keeping them in solution. CMCs differ greatly depending on the nature of the different detergents and on the composition of the solution. Domiphen bromide CMC can drop 50 fold depending on the concentration of NaCl! Low CMC detergents would “foam” easily and are not good for Cationic solubilisation purposes. detergent Source: https://www.biotek.com/resources/application-notes/rapid-critical-micelle-concentration-cmc-determination-using-fluorescence-polarization/ ROLE OF DETERGENTS IN CRYSTALLOGRAPHY Part 1: Solubilise Grow and lyse cells Isolate membranes Solubilize protein – high concentration of detergent (many times the CMC) N Purify the micelle-encapsulated protein; exchange of the Z detergent to high-CMC Newby Z et al., Nature Protocols 4, 619 - 637 (2009) Exchange of the detergent to Transfer to Transfer to LCP high-CMC one; nanodisc Stable? Monodisperse? Set screens! ROLE OF DETERGENTS IN CRYSTALLOGRAPHY Part 2: Crystallise Grow and lyse cells Isolate membranes Type I – hydrophobic Type II - micelle mediated contacts (polar contacts/lamellar stacks heads of lipids only) Solubilise protein – high concentration of detergent (many times the CMC) Purify the micelle- encapsulated protein; Type III – vesicular proteoliposomes forming crystal lattice exchange of the detergent to Russo Krauss et al., Int. J. Mol. Sci. 2013, 14(6), 11643-11691 high-CMC Exchange of the detergent to Transfer to Transfer to LCP high-CMC one; nanodisc Stable? Monodisperse? Set screens! ROLE OF DETERGENTS IN CRYSTALLOGRAPHY Against logic… make the protein soluble just to make it insoluble again! (Low) (High) Russo Krauss et al., Int. J. Mol. Sci. 2013, 14(6), 11643-11691 CUBIC PHASE CRYSTALLISATION Against logic… make the protein soluble just to make it insoluble again! 3D crystal growth Wallace et al., PLoS One, 2012 https://doi.org/10.1371/journal.pone.0024488 CUBIC PHASE CRYSTALLISATION Wallace et al., PLoS One, 2012 https://doi.org/10.1371/journal.pone.0024488 DETERGENT PROBLEM AND A POSSIBLE SOLUTION SMART Solution – SMALP!! Negative stain EM reconstruction of SMALP- solubilised AcrB (Postis et al., Biochim Biophys Acta. (2015); 1848(2): 496–501) NEW TECHNOLOGY - SMA But can we bypass the detergent altogether? Styrene maleic acid co-polymer (SMA) solubilises membrane proteins direct from the membrane into a nanoscale SMA-lipid particle (SMALP) Styrene Maleic Acid (SMA) pH-dependent excision of intact TM protein from membrane Lipids SMA K+ channel Paulin S et al., Nanotechnology 25 (2014) 285101 (7pp) Dörr et al., (2014) PNAS 111, 18607 Membrane proteins in Cryo-EM Membrane proteins in Cryo-EM Image classification allows studying macromolecular dynamics. Mixtures of macromolecular complexes in distinct conformational or compositional states may be imaged directly, and image classification may be used to obtain structures for each of the states. Thereby, image classification allows characterization of the functional cycles of dynamic molecular machines from a single experiment. Different functional states of the eukaryotic V-type ATPase61 are shown Nature. 2016 September 15; 537(7620): 339–346. as an example. doi:10.1038/nature19948. Membrane proteins in Cryo-EM State-of-the-art! cryo-EM density map for β-galactosidase bound to the inhibitor phenylethyl β-D-thiogalactopyranoside where the ordered regions are resolved at a level of detail seen in X-ray maps at ∼ 1.5 Å resolution. The cryo-EM ‘‘revolution’’ has been fueled in large Bartesaghi et al., Atomic Resolution Cryo-EM Structure of -Galactosidase, measure by advances in detector technology and image Structure (2018), https://doi.org/ 10.1016/j.str.2018.04.004 processing methods. Model Membranes Liposomes are bilayer-encapsulated vesicles Important tools for studying membrane properties Useful for drug delivery Vary in size from 25nm to 1mm Figure 10-9 Molecular Biology of the Cell (© Garland Science 2008) Model membranes Front. Physiol., 13 February 2017 https://doi.org/10.3389/fphys.2017.00063 Lipid membrane model systems ranging from flat lipid bilayers to differently sized liposomes. SUV, small unilamellar vesicle; LUV, large unilamellar vesicle; MLV, multilamellar vesicle; MVV, multivesicular vesicle; GUV, giant unilamellar vesicle. Bicelles are detergent-stabilized phospholipid bilayer discs into which membrane proteins can be reconstituted for biophysical studies. Black Lipid Membranes (BLMs) Electrophysiology setup using BLM Lipid bilayer spread over teflon and then stretched until only a single bilayer covers the hole between two electrolyte solutions. BLMs – important for the study of membrane channels. Allow for electrophysiological measurements of membrane; membrane permeability and polarity in vitro – as they allow electrodes to be placed on both sides of the membrane! Patch Clamping of membranes Image from http://www.els.net/WileyCDA/ElsArticle/refId-a0003382.html DOI:10.1002/9780470015902.a0003382.pub2 Interactive video - https://neuroscience5e.sinauer.com/animations04.01.html Lipid Rafts Lipids might form rafts Some lipid classes form stable interactions and therefore may result in the formation of “rafts” Those can form functional microdomains and can Lehninger Principles of Biochemistry David L. Nelson; Michael Cox sequester specific proteins and other molecules Here phospholipids mixture is shown to partition into patches in artificial liposomes Figure 10-13&14 Molecular Biology of the Cell (© Garland Science 2008) Lipids might form rafts Atomic Force Microscopy (AFM) images of rafts show elevations Cholesterol and sphingomyelin are thought to form rafts Sphingomyelin molecules are long and saturated - their patches are thicker Figure 10-12&14 Molecular Biology of the Cell (© Garland Science 2008) Roles of the rafts Segregation of proteins: In the Golgi membranes glycosphingolipids and cholesterol form rafts associating with specific cargo proteins Rafts preferentially attract signalling proteins e.g. kinases – clustering activity and amplification of signal However, the precise role of lipid rafts is still unclear Interactions within the membrane – a summary A) Interleaflet coupling [interdigitating lipid acyl chains in green-gray; zoom: interdigitating ethyl groups of upper (green) and lower (red) leaflets]; B) Asymmetric distribution of lipids and ions [right hand-side: color-coding of lipid species]; C) Negatively charged lipids (in yellow) of the plasma membrane inner leaflet [for the association of proteins with basic-rich domains (light green)]; D) Lipid self-assemblies (pink); including rafts. E) Hydrophobic mismatch (blue/purple); F) Specific protein-lipid interactions [*sphingolipid- and **cholesterol-binding pockets]. doi: 10.3389/fcell.2016.00106 Summary Phospholipids self-organise spontaneously into bilayers in polar solvents. Bilayers exist in a variety of phases, which change depending on the temperature and composition of the lipid/solvent Lipid fluid lamellar phase resembles the physiologically relevant state of the biomembranes. Cholesterol is an important modifier of phase transitions and creates rafts. Mesophases are created in water-lipid mixtures. Cubic mesophases can be used for crystallisation of the membrane proteins. Detergents are lipid-like compounds which can destabilise the membrane and extract membrane proteins out of membranes - solubilisation. Critical micellar concentration (CMC) is a concentration above which the concentration of free detergent molecules remains constant and they form micelles. Lipid rafts can form functional domains Cryo-EM and X-ray crystallography are the principal approaches for determining the structure of membrane proteins