BYM2101 Basic Principles in Molecular Biology PDF
Document Details
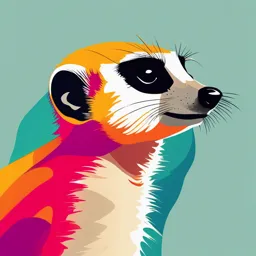
Uploaded by WellEducatedRetinalite3611
Tags
Related
- Human Genetics and Molecular Biology Notes PDF
- Molecular Biology and Genetics - Explorations: An Open Invitation to Biological Anthropology (2nd Edition) PDF
- Human Genetics and Molecular Biology PDF
- Chapter 13 Genetics and Molecular Biology - PDF
- Molecular Biology and Genetics Exam January 2023 PDF
- Molecular Biology Summary PDF - DNA, RNA, Genetics
Summary
This document provides an overview of the course "BYM2101 Basic Principles in Molecular Biology." It includes the course aim, content, recommended books, learning outcomes, weekly topics, evaluation, and calendar.
Full Transcript
BYM2101 Basic Principles in Molecular Biology The aim of the course To teach; The structure and basic functions of the genetic material in the cell How they manage the organisms, The processing, regulation, damage and repair of the genetic material, How the basic theoretical...
BYM2101 Basic Principles in Molecular Biology The aim of the course To teach; The structure and basic functions of the genetic material in the cell How they manage the organisms, The processing, regulation, damage and repair of the genetic material, How the basic theoretical knowledge is applied (technology) Course Content Advances in Molecular Biology, Structure and Functions of Nucleic Acids, DNA Replication, Genome Organization, Gene Mutations and Repair Mechanisms, Transcription and Post-Transcriptional Regulations in Prokaryotes and Eukaryotes, Protein Structure and Synthesis Mechanism Epigenetics Recommended Books -Fundamental Molecular Biology, Lizbeth A. Allison, Jonn Wiley & Sons, Inc., USA -Leland H. Hartwell et al., Genetics: From Genes to Genomes. McGraw Hill Edu. USA. - David Clark Nanette Pazdernik, Molecular Biology, Academic Cell, USA - Nancy L. Craig... et al., Molecular biology: principles of genome function, Oxford: Oxford University Press. Learning Outcomes STUDENTS; 1. Understand the developments in molecular biology and the events inside the cell and gain the ability to follow the literature. 2. Will be able to describe the structure, organization and function of the genetic material in the cell, 3. Will be able to discuss and analyze molecular mechanisms related to genetic material. 4. Understand the role of molecular biology in solving diseases. 5. Will be able to use the basic principles of Molecular Biology for the analysis of problems in nature Weekly Topics INTRODUCTION TO MOLECULAR BIOLOGY THE STRUCTURE OF DNA THE STRUCTURE OF RNA THE STRUCTURE AND FUNCTION OF PROTEIN FROM NUCLEOTIDES TO CHROMATIN (Genome Organization) DNA REPLICATION AND TELOMERE MAINTENANCE MUTATIONS AND REPAIR MECHANISIMS TRANSCRIPTION IN PROKARYOTES TRANSCRIPTION IN EUKARYOTES RNA PROCESSING AND POSTTRANSCRIPTIONAL GENE REGULATION THE MECHANISM OF TRANSLATION EPIGENETIC MECHANISMS OF GEN REGULATION EVALUATION How to calculate the end-of-term average score First Midterm: 30% (week 8/9) Second Midterm: 30% (week 13/14) End Term (Final) Exam: 40% (week 16-17) (+)s from in-class activities will be added directly to the end-of- term average score. CALENDAR The 2024-2025 Fall Semester is a total of 15 weeks. Final exams will be held on January 13-23 January 27th - February 1st Make Up exams February 17th, 2025 Spring Semester start INTRODUCTION TO MOLECULAR BIOLOGY For decades, DNA was largely an academic subject and not the source of dinner table conversation in the average household. In 1995 this changed when media coverage of the O.J. Simpson murder trial brought DNA fingerprinting to homes across the world. Two years later, the cloning of Dolly the sheep was headline news. Then, in 2001, scientists announced the rough draft of the human genome sequence. In commenting on this landmark achievement, former US President Clinton likened the “decoding of the book of life” to a medical version of the moon landing (1961, N.Armstrong). Increasingly, DNA has captivated Hollywood and the general public, excited scientists and science fiction writers alike, inspired artists, and challenged society with emerging ethical issues. X-ray diffraction photograph of DNA. This image of the DNA double helix was obtained by Rosalind Franklin in 1953, the year in which Watson and Crick discovered DNA’s structure, aided by Franklin’s work. The image results from a beam of X-rays being scattered onto a photographic plate by the crystalline DNA. Various features about the structure of the DNA can be determined from the pattern of spots and bands. The cross of bands indicates the helical nature of DNA. The real starting point of Molecular Biology occurred when James D. Watson and Francis Crick suggested the three dimensional (3D) structure the of deoxyribonucleic acid (DNA) in 1953. The discoverers of the structure of DNA. James Watson (left) and Francis Crick seen with their model of part of a DNA molecule in 1953. History of Molecular Biology 384-322 B.C. Aristotle proposes the theory of pangenesis (hereditary characteristics are carried and transmitted by gemmules from individual body cells). 1866 Gregor Mendel (the “Father of Modern Genetics”) publishes his paper on inheritance of traits in peas 1869 Friedrich (Fritz) Miescher isolates an acidic, phosphorus-rich substance he called “nuclein” from the nuclei of white blood cells in pus from soiled bandages 1875 E. Strasburger describes what will later be called chromosomes 1882 Walter Flemming describes behavior of chromosomes during mitosis 1884 E. Strasburger, Oscar Hertwig, R.A. von Kölliker and August Weismann independently identify the cell nucleus as the basis of inheritance 1888 W. Waldeyer names chromosomes (“color bodies”) 1889 Hugo DeVries hypothesizes the existence of “pangenes 1893 August Weismann proposes his “germ plasm” theory and challenges the widely held idea that acquired characteristics can be inherited 1900 Hugo DeVries, Karl Correns, and Erich Von Tschermak independently rediscover and verify Mendel’s Laws 1902 Walter S. Sutton and Theodore Boveri observe that chromosomes in cells behave in ways parallel to Mendel’s characters during meiosis, and propose a chromosomal basis for heredity 1902 Archibold Garrod explains the concept of human inborn errors of metabolism, linking inheritance to proteins 1905 Nettie M. Stevens and Edmund B. Wilson independently develop the idea of sex determination by chromosomes 1902 Walter S. Sutton coins the term “genes” for Mendel’s “characters” 1905 Reginald C. Punnett devises the Punnett square 1906 William Bateson coins the term “genetics” 1910-1913 Thomas H. Morgan and Alfred H. Sturtevant announce the gene theory and chart the first linear map of genes 1928 Frederick Griffith demonstrates a heritable “transforming principle” that transmits the ability of bacteria to cause pneumonia in mice 1929 Phoebus Aaron Levene characterizes and names the compounds ribonucleic acid and deoxyribonucleic acid, and a “tetranucleotide” structure of DNA, in which the 4 bases of DNA are arranged one after another in a set of 4 1941 George W. Beadle and Edward L. Tatum formulate the one gene-one enzyme hypothesis 1938 Rudolf Signer, Torbjorn Caspersson and Einer Hammarstein find molecular weights for DNA between 500,000 and 1,000,000 daltons, suggesting that DNA must be a polynucleotide Proteins and DNA are studied by many scientists using X-ray crystallography. The term “molecular biology” is coined by Warren Weaver 1944 Owald Avery, Colin MacLeod, and Maclyn McCarty demonstrate that Griffith’s bacterial transforming principle is not protein but DNA and suggest that it may function as the genetic material 1949 Roger and Colette Vendrely, together with André Boivin, show a constant amount of DNA in all tissues of the same animal and find half as much DNA in the nuclei of sperm cells as they find in body cells 1950 Erwin Chargaff shows amounts of the bases A and T, and G and C are equal Chargaff ’s rules. 1.The number of A residues in all DNA samples was equal to the number of T residues. [A] = [T], Accordingly, the number of G residues equals that of C. [G] = [C]. 2.The amount of the purine bases equals that of the pyrimidine bases: [A] + [G] = [T] + [C]. 1952 Alfred Hershey and Martha Chase use bacteriophage (viruses) to confirm that DNA is the hereditary material 1953 Maurice Wilkins and Rosalind Franklin use X-ray crystallography to reveal the repeating structure of B-form DNA (using DNA purified by Signer) Double helix structure of DNA is first described by Watson and Crick 1953 Cohen and Boyer, and their team, developed genetic engineering 1973 A gene from frog was transferred into bacterial cells The first human protein (somatostatin) is produced in a bacterium (E. coli) 1977 The first recombinant protein (human insulin) approved for the market 1982 Polymerase chain reaction (PCR) technique developed 1983 Launch of the Human Genome Project 1990 The first genome sequence of an organism (Haemophilus influenzae) is determined 1995 A first draft of the human genome sequence is completed 2000 Tens of millions of gene sequences from prokaryotes and eukaryotes are deposited in GenBank. CRISPR-Cas9 genom editing system/technology wins the Nobel Prize 2020 The connection between DNA and heredity, however, was not demonstrated until the middle of the 20th century. Much of the inspiration for the study of heredity originated from the research of Gregor Johann Mendel, an Augustinian monk working in Austria in the 1860s. Mendel bred different varieties of garden peas (Pisum sativum), such as those with round seeds and wrinkled seeds. He then compared the characteristics of parents and offspring. Results from his experiments led to the formulation of what Mendel described as “the law of combination of different characters”. Mendel’s report was disinterested 36 years. The significance of his work was finally recognized in 1900, upon independent rediscovery of his principles by Hugo DeVries (the Netherlands), Karl Correns (Germany), and Erich Von Tschermak (Austria). This marked the age of classic or Mendelian genetics. The basic principles of genetics; the law of segregation the law of independent assortment the concept of dominant and recessive traits are attributed to Gregor Mendel. The law of combination of different characters. Mendel studied the inheritance of seed change. We know today that wrinkled seeds possess an abnormal form of starch. Mendel’s genetic hypothesis is the 3:1 ratio of dominant/recessive phenotypes observed in the F2 generation of a monohybrid cross. True-breeding (homozygous) round (R) seeds and true- breeding wrinkled (r) seeds were planted. Plants were grown and cross-pollinated and allowed to regrow and mature. The heterozygous F1 plants (Rr) were allowed to self- pollinate and the F2 generation was analyzed: 3/4 round, 1/4 wrinkled (3 : 1 ratio). The law of combination of different characters. Proof of transforming factor (In vivo Experiment) In 1928, Frederick Griffith described a transforming principle that transmitted the ability of bacteria to cause pneumonia in mice. Griffith used pathogenic and nonpathogenic strains of Streptococcus pneumoniae to infect mice. Pathogenic strains: S strains are protected against the defense system of organisms (like mice) due to the polysaccharide coats they synthesize. Therefore, S strains cause pneumonia Nonpathogenic strains (R) lack the polysaccharide coat. Therefore, are not protected against the defense system of organisms and do not cause pneumonia because without the protective coat, the bacteria are attacked and killed by the immune system of the infected animal. When Griffith injected mice with live bacteria of an S strain, they invariably died of pneumonia. When he injected mice with live R bacteria, the mice remained healthy. Mice injected with heat-killed S bacteria also remained healthy. However, when heat-killed S bacteria and live R bacteria were injected together, the mice died. Griffith called this the “transforming principle.” He concluded there was transfer of some component of the pathogenic (S) bacteria which allowed the nonpathogenic (R) bacteria to make the polysaccharide coat and evade the mouse immune response. Griffith Experiment In vitro experiments Oswald Avery, Colin MacLeod, and Maclyn McCarty used this assay to show in 1944 that Griffith’s transforming principle was DNA not RNA or protein. They demonstrated that purified DNA was sufficient to cause transformation, and that the transforming factor could be destroyed by enzymes that degrade DNA (deoxyribonucleases) but not by protease or ribonuclease enzymes. The importance of technological advances: the Hershey–Chase experiment, 1952 An important event in the history of the characterization of DNA was the emerging availability and utility of radioisotopes in basic science research in the early post-World War II years. Radioisotopes Different isotopes of the same element have the same number of protons in their atomic nuclei but differing numbers of neutrons. Radioisotopes are radioactive isotopes of an element. They can also be defined as atoms that contain an unstable combination of neutrons and protons, or excess energy in their nucleus. Radioisotopes allowed Alfred Hershey and Martha Chase to carry out a classic experiment in 1952 showing that the genetic material of a virus that infects bacteria, bacteriophage T2 (literally “bacterium eater”), is DNA. The DNA of bacteriophage T2 (phage for short) was known to be contained within a protein coat. Hershey and Chase designed an experiment to determine whether the protein or DNA carried the genetic information to make a new phage. First, they selectively labeled phage DNA with the radioactive isotope 32-phosphorus (32P) and phage protein with 35-sulfur (35S). DNA contains phosphorus but no sulfur; while protein is composed of some sulfur (in the amino acids methionine and cysteine) but no phosphorus. Next, they incubated bacteria (Escherichia coli) with the labeled phage. During infection, the phage attaches to the bacterium and injects its DNA. The importance of technological advances: the Hershey– Chase experiment, 1952 They separeted bacteriophage from bacteria (Escherichia coli) after a short period of incubation. 35S did not pass into the bacterial cell since the phage protein did not enter the bacterial cell, but 32P phage passed into bacterial cell since phage DNA entered into bacterial cell. This finding demonstrated clearly that DNA is the genetic material in a system Further suggeston is that, could DNA be the universal hereditary material? The next challenge, however, was to explain how DNA could contain enough information to control the life of an organism. DNA was thought to be a fairly simple (tetranucleotide) molecule at that time. How could it replicate, pass itself along cell after cell, and retain the message? One gene–one enzyme hypothesis Normally, Neurospora (mold-single cell microfungus) can be grown on a defined minimal medium (MM). (Autotrops-wild type). But mutants are not. They are grown in supplemented medium (SM). Minimal medium is consisting of sugar, some inorganic acids and salts, a nitrogen source, and niacin (vitamin B3). Supplemented medium is consisting of Minimal Medium, Vitamins, AAs., Purine and pyrmidine base (A, G, C, T, U) Mutation is induced in the synthesis pathway of a compound that is vital and not found in the minimal medium. Molds that were not subject to mutation were able to synthesize this compound. Beadle and Tatum induced mutations in the mold by means of X-irradiation and isolated mutants that required specific supplementary compounds in order to grow (auxotrophs /heterotrops-mutant). Mutant molds did not grow in the (MM). Most probably a metabolic step leading to the synthesis of a specific compound had been blocked. They added Vitamins, Amino Acids and DNA and RNA bases (purine and pyrimidine bases-A, G, C, T, U) to the MM and observed that the molds were grown in the supplemented medium (SM). Selection experiment was made to find out in which metabolic pathway (synthesis steps of a substances) the mutation occurred. They divided mutant organisms into 3 different tubes and each tube was added: A. Mutant molds+MM+ AAs B. Mutant molds+MM+Vitamins C. Mutant molds+MM+ DNA and RNA bases (purine and pyrimidine bases-A, G, C, T, U) Mutant molds were grown in the medium where MM+AAs were added. In the next step, they transferred the mutant organisms into MM medium supplemented with different AAs and observed that mutants were grown in MM medium supplemented with tyrosine AA. As a conclusion, they proved that the mutation occurred in the tyrosine synthesis pathway. There was a one-to-one correspondence between a genetic mutation and the lack of a specific enzyme required in a biochemical pathway. From their results, they formulated the “one gene–one enzyme hypothesis.” Beadle and Tatum’s hypothesis was later revised to the “one gene– one polypeptide hypothesis.” One gene–one enzyme hypothesis SUMMARY Although DNA has been known as genetic material since 1944, it was considered as an academic subject until the mid-90s. However, 3 important events that occurred in the 90s made DNA a popular subject. It is now a topic of public conversation In 1995 O.J. Simpson (Hollywood actor), murder trial brought DNA fingerprinting to homes across the world. Two years later, the cloning of Dolly the sheep was headline news. Then, in 2001, scientists announced the rough draft of the human genome sequence. History of Molecular Biology 1866 Gregor Mendel (the “Father of Modern Genetics”) publishes his paper on inheritance of traits in peas 1900 Hugo DeVries, Karl Correns, and Erich Von Tschermak independently rediscover and verify Mendel’s Laws 1928 Frederick Griffith demonstrates a heritable “transforming principle” that transmits the ability of bacteria to cause pneumonia in mice 1941 George W. Beadle and Edward L. Tatum formulate the one gene-one enzyme hypothesis 1944 Owald Avery, Colin MacLeod, and Maclyn McCarty demonstrate that Griffith’s bacterial transforming principle is not protein but DNA and suggest that it may function as the genetic material 1950 Erwin Chargaff shows amounts of the bases A and T, and G and C are equal Chargaff ’s rules. 1. The number of A residues in all DNA samples was equal to the number of T residues. [A] = [T], Accordingly, the number of G residues equals that of C. [G] = [C]. 2. The amount of the purine bases equals that of the pyrimidine bases: [A] + [G] = [T] + [C]. Because a purine always pairs with a pyrimidine 1952 Alfred Hershey and Martha Chase use radioisotope labelled DNA of bacteriophage viruses) to confirm that DNA is the hereditary material 1953 Maurice Wilkins and Rosalind Franklin use X-ray crystallography to reveal the repeating structure of B-form DNA Double helix structure of DNA is first described by Watson and Crick 1953 Cohen and Boyer, developed genetic engineering 1973 A gene from frog was transferred into bacterial cells The first human protein (somatostatin) is produced in a bacterium (E. coli) 1977 The first recombinant protein (human insulin) approved for the market 1982 Polymerase chain reaction (PCR) technique developed 1983 Launch of the Human Genome Project 1990 The first genome sequence of an organism (Haemophilus influenzae) is determined 1995 A first draft of the human genome sequence is completed 2000 Tens of millions of gene sequences from prokaryotes and eukaryotes are deposited in GenBank. CRISPR-Cas9 genom editing system/technology is developed and won the Nobel Prize 2020 Proof of transforming factor (In vivo Experiment) In 1928, Frederick Griffith described a transforming principle that transmitted the ability of bacteria to cause pneumonia in mice. Griffith used pathogenic and nonpathogenic strains of Streptococcus pneumoniae to infect mice. Pathogenic strains: S strains are protected against the defense system of organisms (like mice) due to the polysaccharide coats they synthesize. Therefore, S strains cause pneumonia Nonpathogenic strains: R strains lack of the polysaccharide coat. Therefore, they are not protected against the defense system of organisms and do not cause pneumonia. Because of the lack of the protective coat, the bacteria are attacked and killed by the immune system of the infected animal. When pathogenic S strain is injected into a mouse, the mouse dies. When nonpathogenic R strain is injected into a mouse, the mouse lives. When heat killed S strain is injected into a mouse, the mouse lives. When dead pathogenic strain and non-pathogenic live strain of Streptococcus pneumoniae bacteria are mixed and injected into a mouse the mouse dies. As a result, it was decided that a compound in the dead pathogenic bacterial strain transformed the live and non-pathogenic bacterial strain into a pathogenic form. Since it was not known what the compound is, it was called a transforming factor. In vitro experiments Oswald Avery, Colin MacLeod, and Maclyn McCarty used this assay to show in 1944 that Griffith’s transforming principle was DNA not RNA or protein. They demonstrated that purified DNA was sufficient to cause transformation, and that the transforming factor could be destroyed by enzymes that degrade DNA (deoxyribonucleases) but not by protease or ribonuclease enzymes. In the in vitro experiment, the DNA of the pathogenic Streptococcus pneumoniae strain was isolated and divided into 3 separate test tubes. Non-pathogenic live S. pneumoniae strain was added to each test tube. In addition, proteinase, which breaks down protein, was added to the first test tube, ribonuclease, which breaks down RNA, was added to the second test tube, and deoxyribonuclease, which breaks down DNA, was added to the third test tube. End of the experiment, pathogenic S. pneumoniae strain was detected in the first two tubes. Pathogenic S. pneumoniae was not detected in the third tube. As a result, it was decided that the material called the transforming factor in the in vivo experiment conducted in 1928 was DNA, not protein and RNA. The DNAse enzyme breaks down DNA. Isolated DNA of pathogenic S. pneumoniae in the third tube lost its activity and could not transform the non- pathogenic S. pneumoniae into a pathogenic form. One gene–one enzyme hypothesis The experiment had been designed to confirm the hypothesis that each gene encodes a different enzyme. Normally, Neurospora (mold-single cell microfungus) can be grown on a defined minimal medium (MM). (Autotrops-wild type). But mutants are not. They are grown in supplemented medium (SM), (auxotrophs /heterotrops-mutant). Minimal medium is consisting of sugar, some inorganic acids and salts, a nitrogen source, and niacin (vitamin B3). Supplemented medium is consisting of Minimal Medium, Vitamins, AAs., Purine and pyrmidine base (A, G, C, T, U) A healthy Neurospora (NS) (single-celled microfungus) can grow in a minimal medium (MM) containing sugar, some inorganic acids and salts, a nitrogen source and niacin (vitamin B3). Supplemented medium consists of MM + other vitamins, amino acids (AAs), Purine and pyrmidine base (DNA nucleotide- A, G, C, T, U). Healty NS is able to synthesize supplemented compounds that are necessary for its growth. Because of the mutation caused by X-ray or UV irradiation (mutation) in the synthesis pathway of a compound, mutant NS can not synthesize the compound that it synthesized by healty NS using minimal medium. Therefore, mutant NS can not grow. In order to find out which metabolic pathway of mutant NS is disrupted, NS is cultivated in separet media each containing different supplemeted substances added to MM. NS can grow one of the supplemented medium. The mutation occurred in the synthesis pathway of the substance that was added to MM and NS growth occure. For example, if there was growth in the supplemented medium where AA (tyrosine) was added, it means that one of the enzymes in the tyrosine sythesis pathway has been mutated/inactivated. Beadle and Tatum’s hypothesis was later revised to the “one gene–one polypeptide hypothesis.”