BMS2001 Micro Lecture 3 PDF
Document Details
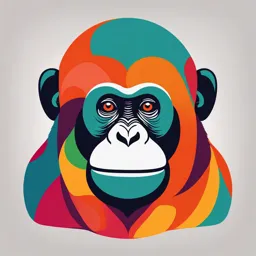
Uploaded by SufficientManticore
Tags
Summary
This document discusses Archaea, their characteristics, and differences between bacterial and archaeal cells. It also touches on viral structure and life cycles. The content provides details for an undergraduate-level microbiology course.
Full Transcript
Archaea Many features in common with Eukarya – genes: replication, transcription, translation Features in common with Bacteria – genes for metabolism Other elements are unique to Archaea – unique rRNA not found in bacteria – capable of methanogenesis Best known for gr...
Archaea Many features in common with Eukarya – genes: replication, transcription, translation Features in common with Bacteria – genes for metabolism Other elements are unique to Archaea – unique rRNA not found in bacteria – capable of methanogenesis Best known for growth in anaerobic, hypersaline, pH extremes, and high‐temperature habitats Also found in marine arctic temperature and tropical waters Archaeal size, shape, arrangement Much like bacteria, cocci and rods are common shapes Other shapes can also exist – branched/flat shapes – no spirochetes or mycelial forms yet Sizes vary (typically 1‐2 x 1‐5 μm for rods, 1‐5 μm in diameter for cocci) Smallest observed is 0.2 μm in diameter Largest (so far) is a multicellular form that can reach 30 mm in length! Bacterial vs. Archaeal Cells Differ from bacterial cell envelopes in the molecular makeup and organization Archaeal Lipids and Membranes Bacteria/Eukaryotes Fatty acids attached to glycerol by ester linkages Archaea Branched chain hydrocarbons attached to glycerol by ether linkages Some have diglycerol tetraethers (fig. b1 and b2), a monolayer structure instead of a bilayer structure Archaeal vs. bacterial cytoplasm Very similar – lack of membrane‐enclosed organelles May contain inclusion bodies (e.g. gas vesicles for buoyancy control) The following may be different: – Ribosomes Bacterial and archaeal ribosomes = 70S Proteins in archaea are more similar to eukaryotes than to bacteria – nucleoid region single circular chromosome Some archaea are complexed with histones polyploidy in some archaea Comparison of Bacteria and Archaea Common Features of Eukaryotic Microbes Membrane‐delimited nuclei Membrane‐bound organelles that perform specific functions Intracytoplasmic membrane complex serves as transport system More structurally complex and generally larger than bacterial or archaeal cells Cilia and Flagella for Motility Flagella (s., flagellum) Cilia (s., cilium) – 100‐200 μm long – 5‐20 μm long – move in undulating – beat with two phases, fashion working like oars set of microtubules in a 9+2 arrangement Virus Structure and Life Cycles Virion size range is ~10–400 nm in diameter and most viruses must be viewed with an electron microscope General Properties of Viruses All virions contain a nucleocapsid which is composed of nucleic acid (DNA or RNA) and a protein coat (capsid) cannot reproduce independent of living cells nor carry out cell division, but can exist extracellularly Capsids: Made of protein subunits called protomers helical, icosahedral, or complex Icosahedral or Complex Capsids An icosahedron is a regular polyhedron with 20 equilateral faces and 12 vertices Some viruses has complex symmetry – poxviruses – largest animal virus – large bacteriophages – binal symmetry head resembles icosahedral, tail is helical Viral Envelopes and Enzymes Viral envelope (lipids and carbohydrates) usually arise from host cell plasma or nuclear membranes Envelope proteins (Spikes), which are viral encoded. – involved in viral attachment to host cell e.g., hemagglutinin of influenza virus – used for identification of virus – may have enzymatic or other activity e.g., neuraminidase of influenza virus Viral Life Cycles Have 5 Steps Nonenveloped viruses lyse the host cell – viral proteins may attack peptidoglycan or membrane Enveloped viruses use budding Lecture 3 – Bacterial Growth Chapter 6,7 Prescott’s Microbiology 10th Edition, McGraw Hill Education Willey Sherwood Woolverton In this lecture, we will learn: Bacterial cell cycle The growth curve Environmental factors that affect microbial growth Laboratory culture of microbes Measurement of microbial growth Reproductive Strategies Eukaryotic microbes – asexual and sexual, haploid or diploid Bacteria and Archaea – haploid only, asexual – Binary fission, budding, filamentous – all must replicate and segregate the genome prior to division Bacterial Reproduction Methods Different From Binary Fission Form a bud Multiple fission to form baeocytes Filamentous, eventually divide to form uninucleoid spores Bacterial Cell Cycle 1. DNA replication and partition 2. Cytokinesis Most bacterial chromosomes are circular Single origin of replication – site at which replication begins Terminus – site at which replication is terminated, located opposite of the origin Replisome – group of proteins needed for DNA synthesis DNA replication proceeds in both directions from the origin Cell Cycle of E. coli Bacteria Cytokinesis ‐ Septation Septation – formation of cross walls between daughter cells Several steps 1. selection of site for septum formation 2. assembly of Z ring (composed of protein FtsZ) 3. assembly of cell wall synthesizing machinery 4. constriction of cell and septum formation Microbial population growth Generation (doubling) time – time required for the population to double in size – varies depending on species of microorganism and environmental conditions – range from 10 minutes for some bacteria to days for some eukaryotes Theoretical estimation of bacterial growth Consider a cell with: – A generation time of 20 min; – A weight of ~9.5 x 10‐13 g 48 h What is the theoretical biomass ? gram after 48 h of growth? N = N02n = 2^(48 x 60 / 20) = 2.23 x 1043 Total biomass after 48 h = 2.23 x 1043 x 9.5 x 10‐13 g = 2.12 x 1031 g Bacterial Growth Increase in cellular constituents that may result in: – increase in cell number – increase in cell size Growth refers to population growth rather than growth of individual cells Note: Exponential growth is only part of the story The Growth Curve Observed when microorganisms are cultivated in batch culture Usually plotted as logarithm of cell number vs. time Has five distinct phases Lag Phase and Exponential Phase Lag Phase Cell synthesizing new components – to replenish spent materials – to adapt to new medium or other conditions Varies in length – in some cases can be very short or even absent Exponential Phase Also called log phase Rate of growth and division is constant and maximal Population is most uniform in terms of chemical and physical properties during this phase Stationary Phase Closed system population growth eventually ceases, total number of viable cells remains constant – active cells stop reproducing, or reproductive rate is balanced by death rate Possible reasons for stationary phase – nutrient limitation – limited oxygen availability – toxic waste accumulation – critical population density reached Death Phase and Prolonged Decline in Growth Death Phase – programmed cell death fraction of population genetically programmed to die (suicide) Prolonged Decline in Growth The whole population -> many curves combine to form this line Bacterial population continually evolves Process marked by successive waves of genetically distinct variants Natural selection occurs Environmental Factors that Affect Microbial Growth Most organisms grow in fairly moderate environmental conditions X extreme Extremophiles – grow under harsh conditions that would kill most other organisms Able to grow very well - under good conditions Temperature Microbes cannot regulate their internal temperature Enzymes have optimal temperature at which they function optimally High temperatures may inhibit enzyme functioning and be lethal Organisms exhibit distinct cardinal growth temperatures – minimal – maximal – optimal Temperature Ranges for Microbial Growth Most human Important source of heat‐ pathogens are stable enzymes, e.g. Taq mesophiles polymerase from Thermus aquaticus Important for food spoilage Those living in hydrothermal vents Those living in ocean depths Adaptations of Thermophiles Protein structure stabilized by a variety of means – e.g. more H bonds, more proline, chaperones Histone‐like proteins stabilize DNA Membrane stabilized by variety of means – e.g. more saturated, more branched and higher molecular weight lipids – e.g. ether linkages (archaeal membranes) Solutes and Water Activity Changes in osmotic concentrations in the environment may affect microbial cells – hypotonic vs hypertonic solution Microbes Adapt to Changes in Osmotic Concentrations Reduce osmotic concentration of cytoplasm in hypotonic solutions – mechanosensitive (MS) channels in plasma membrane allow solutes to leave Increase internal solute concentration with compatible solutes to increase their internal osmotic concentration in hypertonic solutions Halophiles Halophiles – grow optimally in the presence of NaCl or other salts at a concentration above about 0.2M Extreme halophiles – Grow in salt concentrations of 2M and 6.2M – extremely high concentrations of potassium – cell wall, proteins, and plasma membrane require high salt to maintain stability and activity Solutes and Water Activity (2) Water activity (Aw) – the amount of water available to organisms – equal to ratio of solution’s vapor pressure (Psoln) to that of pure water (Pwater) – Aw = Psoln/ Pwater low water activity means most water is bound by solutes Aw of distilled water = 1; Aw of saturated salt solution = 0.75; Aw of dried fruit = 0.5 Osmotolerant microbes can grow over wide ranges of water activity pH Measure of the relative acidity of a solution – negative logarithm of the hydrogen ion concentration Acidophiles – growth optimum between pH 0‐5.5 Neutrophiles – growth optimum between pH 5.5‐8 Alkaliphiles – growth optimum between pH 8‐11.5 pH Tolerance Mechanisms of Microbes Most microbes maintain an internal pH near neutrality – the plasma membrane is impermeable to proton Acidic tolerance response – pump protons out of the cell – some synthesize acid shock proteins and heat shock proteins that protect proteins Many microorganisms change the pH of their habitat by producing acidic or basic waste products Oxygen Concentration Depends on a microbe’s metabolic processes. Almost all processes use electron transport chains (ETC). In many cases, the terminal electron acceptor is oxygen. – aerobe: grows in presence of atmospheric oxygen (O2) which is 20% O2 – anaerobe: grows in the absence of O2 – obligate aerobe – requires O2 – facultative anaerobes: do not require O2 but grow better in its presence – aerotolerant anaerobes: grow with or without O2 – strict anaerobe: usually killed in presence of O2 – microaerophile: requires 2–10% O2 O2 Basis of Different Oxygen Sensitivities Oxygen reduced to reactive oxygen species (ROS) – superoxide radical – hydrogen peroxide – hydroxyl radical Aerobes produce protective enzymes – superoxide dismutase (SOD) – catalase – peroxidase Strict anaerobic microbes cannot tolerate O2 as they lack/have very low quantities of superoxide dismutase and catalase Pressure Microbes that live on land and water surface live at 1 atmosphere (atm) Some Bacteria and Archaea live in deep sea with very high hydrostatic pressures Barotolerant – adversely affected by increased pressure, but not as severely as nontolerant organisms Barophilic organisms – require or grow more rapidly in the presence of increased pressure – change membrane fatty acids to adapt to high pressures Laboratory Culture of Microbes Culture Media Need to grow, transport, store microorganisms in lab Must contain all nutrients organism requires for growth Agar ‐ sulfated polysaccharide solidifying agent; most microorganisms cannot degrade it Defined (Synthetic) Media Complex Media Media whose exact composition is Nutrients derived from a variety known of extracts; can vary slightly from one batch to the next Peptones ‐ protein hydrolysates from protein sources Extracts ‐ aqueous extracts, usually of beef or yeast Functional Types of Media Supportive or general purpose media ‐ support the growth of many microorganisms Enriched media (e.g. blood agar) – general purpose media supplemented with special nutrients Selective Differential Selective Media Favor the growth of some microorganisms and inhibit growth of others – e.g. MacConkey agar (bile salts and crystal violet) selects for Gram‐negative bacteria Differential Media Distinguish between different groups of microorganisms based on their biological characteristics – e.g. blood agar hemolytic versus nonhemolytic bacteria – e.g. MacConkey agar (lactose and neutral red dye) lactose fermenters versus nonfermenters Mechanisms of Action of Media Types Isolation of Pure Cultures Allows for the study of single type of microorganism in mixed culture Spread plate, streak plate (Practical 1), and pour plate are techniques used to isolate pure cultures Microbial Growth on Solid Surfaces Colony characteristics that develop when microorganisms are grown on agar surfaces aid in identification Differences in growth rate from center to edges is due to – oxygen, nutrients, and toxic products – cells may be dead in some areas Measurement of Microbial Growth Can measure changes in number of cells in a population – counting chambers – flow cytometry – membrane filters Can measure changes in mass of population – Turbidity determined to yield the most probable number (MPN) of viable microbes