U.S. Army Medical Center of Excellence Interservice Physician Assistant Program Block 2-1 Microbial Genetics PDF
Document Details
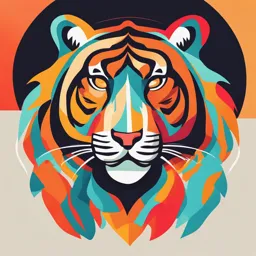
Uploaded by IncredibleSerpentine9954
U.S. Army Medical Center of Excellence
2024
MAJ Hulseberg, PhD, ASCP(M)
Tags
Related
Summary
This document provides an overview of microbial genetics, including the structure and function of genetic material, gene replication, gene expression, and genetic variation in microorganisms. It covers key concepts such as DNA replication and transcription, along with related concepts of genetic transfer. The material is presented in a lecture format. It may be for a physician assistant program.
Full Transcript
U.S. Army Medical Center of Excellence Interservice Physician Assistant Program Block 2-1 Microbial Genetics Fall 2024 MAJ Hulseberg, PhD, ASCP(M) Terminal Learning Objective Explain the structure and function of microbial genetic material a...
U.S. Army Medical Center of Excellence Interservice Physician Assistant Program Block 2-1 Microbial Genetics Fall 2024 MAJ Hulseberg, PhD, ASCP(M) Terminal Learning Objective Explain the structure and function of microbial genetic material and the mechanisms of gene replication, gene expression, and genetic variation in microorganisms Enabling Learning Objectives Define terms associated with microbial genetics such as genome, transcription, translation, mutation, etc. Identify the composition, structure, and function of microbial genetic material Explain the components and mechanisms of genome replication, transcription, and translation of microbial genes including the nature of the genetic code Explain the factors affecting gene regulation and expression including inducible and repressible operons Describe types, mechanisms, and effects of genetic transfer and gene alterations in microbes such as plasmids, transformation, transduction, conjugation, and mutation Outline DNA replication Transcription Translation Gene expression/regulation Mutations Genetic transfer Central Dogma of Molecular Biology Function of DNA is to carry genetic information and transfer it to new cells as strands of DNA are copied Nucleic Acid Structure Nitrogenous base (A, T, G, C) Nitrogenous base OH (A, U, G, C) 5’ RNA nucleotide 3’ DNA Structure DNA is a double-stranded helix dsDNA Two strands of paired nucleotides (bases) attached to deoxyribose sugar backbone Complementary base pairing Adenine to thymine Guanine to cytosine Strand direction (orientation) Antiparallel strands 3’ end: end without phosphate bound to 3’ carbon 5’ end: end with phosphate bound only to 5’ carbon DNA Replication Strands in original or parent DNA molecule separate Unwinding (and rewinding) due to action of enzymes which break hydrogen bonds between bases and/or hold part of strand stable Replication fork forms as dsDNA helix unwinds, exposing the separated strands Allows synthesis of complementary DNA strands using parent strands as templates DNA Replication DNA polymerase catalyzes replication process at replication forks DNA polymerase adds new complementary nucleotides to exposed 3’ end of growing strand Can only catalyze binding to 3’ end of an existing nucleotide i.e., incoming nucleotides cannot bind to 5’ end DNA Replication New strand with 3’ end nearest the replication fork is called leading strand, and it grows toward the replication fork New strand with 3’ end facing away from the replication fork is called the lagging strand Replication on lagging strand is discontinuous (more complicated) Lagging strand requires primase and RNA primer to initiate DNA polymerase synthesizes short sections called Okazaki fragments (not connected into continuous strand) DNA ligase: polymerase that inserts a nucleotide to join Okazaki fragments Joins fragments at 3’ and 5’ carbons of nucleotide DNA Replication Semiconservative replication Each new double-helix consists of one new strand and one original strand Complementary base pairing ensures correct placement of nucleotides in new "daughter" DNA strands Editing by DNA polymerase corrects for errors in placement of nucleotides Transcription Transfer of genetic code carried on DNA gene onto a messenger RNA (mRNA) strand by means of DNA- dependent RNA polymerase Ribonucleic Acid (RNA) mRNA, tRNA, rRNA Differences from DNA 2’ carbon on ribose has a hydroxyl group Uracil (U) instead of thymine (T) Single-stranded but with extensive intramolecular base-pairing Messenger RNA (mRNA) Carries genetic code from DNA to site of protein synthesis (ribosome) Genetic code in the form of a triplet code or codon Codons – sets of three nucleotides on mRNA that specify a given amino acid in polypeptide sequence 3 nucleotides = 1 codon 1 codon = 1 amino acid Codons are arrayed in a specific reading frame Three possible reading frames Only one is correct for protein synthesis Codons Examples UUU, UUC → phenylalanine GCU, GCC, GCA, CCG → alanine AUG → methionine / Start UAA, UAG, UGA → Stop Practice UUU – Phe UUU – Phe UUU – Phe CUU – ? UCU – ? UUC – Phe AUU – ? UAU – ? UUA – ? GUU – ? UGU – ? UUG – ? 25% Phe 25% Phe 50% Phe 25% Leu 25% Ser 50% Leu 25% Ile 25% Tyr 25% Val 25% Cys Why 64 combinations for just 20 amino acids? Degeneracy protects cell from minor errors in DNA or mRNA Codons Coding strand AKA sense strand DNA mRNA Template strand Transcribed strand AKA noncoding or antisense strand Polypeptide Transfer RNA (tRNA) Transports and then transfers amino acids to growing peptide chain Acceptor stem: binding site for a specific amino acid Anticodon: site on the tRNA that binds with the codon on the mRNA Specifies which amino acid will be carried by the tRNA Acceptor stem Anticodon stem Ribosomal RNA (rRNA) Assists protein synthesis by serving as a “facilitator” Site where codons (mRNA) and anticodons (tRNA) meet Prokaryotic ribosomes are ~40% protein (blue) / 60% rRNA (brown) Peptide bonds between amino acids catalyzed by the large ribosomal subunit 50S (50S) mRNA message decoded by the mRNA 30S small ribosomal subunit (30S) Ribosomal Designations De facto units of ribosomes are Svedberg (S) values, which are based on how rapidly the subunits settle to the bottom of test tubes under the centripetal force of a centrifuge (the sedimentation rate) Eukaryotic ribosomes have Svedberg values of 80S Small subunit is 40S Large subunit is 60S Prokaryotic ribosomes have Svedberg value of 70S Small subunit is 30S Large subunit is 50S Remember these S units are NOT units of mass (otherwise, the math here would make no sense ☺) Transcription Three main steps: Initiation Elongation Termination G C Transcription Initiation RNA polymerase binds to the promoter region upstream of gene to be transcribed Signals DNA to unwind so polymerase can “read” bases Unwound DNA (exposed gene) is called the transcription bubble G Transcription Elongation - addition of nucleotides (NTPs) Template to an mRNA strand Coding strand RNA polymerase reads unwound DNA strand strand Moves ~40 bases per second Transcription proceeds 5’ → 3’ direction Strand that is read is called the template strand Also called theG noncoding or antisense strand mRNA Transcription Termination – transcription ends when RNA polymerase crosses a stop (termination) sequence in the gene Uracil triphosphate (UTP) is added to the mRNA Polymerase stops and releases the transcript (mRNA strand) G Transcription Template strand Check on Learning Coding strand The sequence of the new mRNA transcript is complementary to which strand of DNA: template strand or coding strand? Which strand has the same sequence as the mRNA? G Template -ATGATCTCGTAA- Coding -TACTAGAGCATT- mRNA? -AUGAUGUCGUAA- Translation Translation and transcription are couple processes in prokaryotes No compartmentalized nucleus to separate activities Stop codon Translation Three different tRNA binding sites in large ribosomal subunit A (aminoacyl) site – correct tRNA is selected for codon being read P (peptidyl) site – transpeptidation (peptide bond formation) between amino acids on adjacent tRNAs E (exit) site – empty (uncharged) tRNA is ejected out of the ribosome Important for understanding mechanism of action for antibiotics targeting protein synthesis Translation Termination mRNA continues to be fed through ribosome 5’ → 3’ until reading frame reaches “stop” codon Polypeptide chain is then released Ribosome either dissociates or shuffles along to the next start codon on the mRNA Regulation of Gene Expression 1 = Constitutive gene Always Transcribed 1 Bacterial Chromosome 2 = Inducible gene 3 = Repressible gene Substrate A 2 Substrate B 3 Transcription turned ON Transcription turned OFF Operons Related genes that are regulated as a group (e.g., related genes which code for a specific metabolic pathway) Regulatory gene – codes for repressor protein which can bind to operator site Promoter site – binding site for RNA polymerase during transcription Operator site – binding site for repressors (only in prokaryotes) Operons Inducible operon – expressed only when certain environmental conditions are present “Positive control” (off until turned on) Example: lac operon The lac operon is an inducible operon that is subject to activation in the absence of glucose Encodes three structural genes, lacZ, lacY, and lacA, needed to acquire and process the disaccharide lactose from the environment Operons In the absence of lactose….. lac repressor binds with operator sequence Prevents RNA polymerase from initiating transcription Operons Operons Repressible operon – expressed except when certain environmental conditions are present (“negative control”) Example: trp operon In the absence of tryptophan, the trp repressor dissociates from operator → RNA polymerase transcribes operon When tryptophan accumulates, tryptophan binds to a repressor Bound repressor then binds to operator and transcription is blocked Mutations Changes in sequence of nucleotide bases in the genome Caused by substitutions, insertions, or deletions Can affect functional or structural characteristics (altered phenotype) Researchers watched HIV evolve in the laboratory Complexity/diversity of protein structure in response to pressure from an antiviral drug. (Slide 18 of Block 1 Immunology deck) Viruses were subjected to increasing amounts of the drug. Mutant viruses quickly emerged, and after a few weeks, a highly resistant strain with six mutations dominated the population of viruses. Mutations Most, but not all, mutations are deleterious (bad) for the pathogen Viruses mutate quickly under selective pressure of antivirals Researchers watched HIV evolve in the laboratory in response to pressure from an antiviral drug. Viruses were subjected to increasing amounts of the drug. Mutant viruses quickly emerged, and after a few weeks, a highly resistant strain with six mutations dominated the population of viruses. Mutations Causes of mutations Spontaneous mutations – no external cause Replication errors, failure to repair damage, etc. Typically occurs in one in a million replicated genes RNA viruses have more spontaneous mutations and lengthier insertions or deletions Chemical mutagens, e.g. nitrous oxide, base analogs Radiation – X-rays, gamma rays, ultraviolet light Mutations Base substitution – single base is paired with incorrect base Resulting codon change causes: Silent → no change to the amino acid → no effect Ex: CAA (glutamine) → CAG (glutamine) Missense → different amino acid → variable effect Ex: CAA (glutamine) → CCA (proline) Nonsense → protein is incomplete because RNA polymerase is stopped from reading the code → serious effect Ex. CAA (glutamine) → UAA (stop) Normal (“Wild type”) Substitution (Mutation) Mutations Frameshift mutation Insertion or deletion of bases that shifts the reading frame Often results in nonsense mutation Usually alters phenotype (different, non-functional or incomplete protein) Wild-type Insertion Deletion Genetic Transfer Plasmids – small, circular, self-replicating pieces of DNA in bacteria Separate from chromosomal DNA Inheritable / vertically-transmitted (sometimes called “replicons”) Genes usually not essential for growth Genes often code for antibiotic resistance or virulence factors Often manipulated in genetic engineering Bacterial chromosome Plasmids Genetic Transfer Recombination – process of transferring DNA from one bacterial cell to another Generates new genetic combinations 3 types of bacterial recombination Conjugation Transformation Transduction Genetic Transfer Conjugation Transfer of genetic material between two (donor and recipient) bacterial cells involving cell-to-cell contact Sex pilus is a tube connecting two bacterial cells Copy of DNA strand or plasmid is transferred via sex pilus Transferred plasmid may code for antibiotic resistance, metabolic enzyme, or other product Genetic Transfer Transformation Direct uptake of DNA segment (i.e., transfer of genes) from one bacterium to another as “naked” DNA Can confer new characteristics (new phenotype) to recipient cells Genetic Transfer Transduction, Part I DNA from bacteriophage can integrate into bacterial chromosome during lysogenic cycle Once integrated, gene(s) replicate with bacterial chromosome Integrated viral genes are called prophages Viral gene may code for antibiotic resistance or virulence factors Possible to enter lytic phase if prophage separates (“excises”) from bacterial chromosome During assembly step, bacteriophage may “accidentally” package some host (bacterial) DNA Genetic Transfer Transduction, Part II Transduction is the transfer of DNA from donor bacterium to recipient bacterium via bacteriophage Ex. Cholera toxin phage (CTXφ) infects Vibrio cholerae and transfers the cholera toxin Host DNA or plasmid from infected bacterium “accidently” packaged in phage particle during assembly Genetic Transfer Viral Reassortment Genetic reassortment – process of genetic recombination that occurs when two similar viruses infect the same cell and exchange genetic material Happens when nucleic acids from a segmented viral genome are partially assembled into a different virus May result in major (including lethal/non-functional) changes Questions?