Biopolymers PDF
Document Details
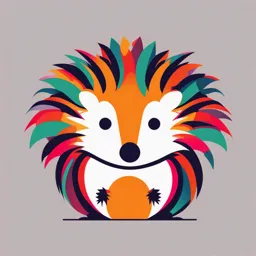
Uploaded by GenialPoltergeist
Tags
Summary
This document discusses biopolymers, which are naturally occurring polymers found in various living organisms. It covers different types of biopolymers, such as polysaccharides, proteins, and nucleic acids, along with their properties, uses, and applications across various industries. The document also examines cellulose and its derivatives like Carboxymethylcellulose (CMC).
Full Transcript
7Biopolymers are naturally occurring polymers produced by living organisms, including plants, animals, and microorganisms. These materials consist of monomeric units covalently bonded to form larger molecular chains. They are categorized into three main types based on their origin and composition:...
7Biopolymers are naturally occurring polymers produced by living organisms, including plants, animals, and microorganisms. These materials consist of monomeric units covalently bonded to form larger molecular chains. They are categorized into three main types based on their origin and composition: 1. Polysaccharides: These are carbohydrate-based biopolymers, such as cellulose, starch, and chitin. They serve as structural components in plants and animals or as energy storage molecules. 2. Proteins: Proteins are biopolymers composed of amino acid monomers. Examples include collagen (a structural protein in connective tissues) and silk (produced by insects and spiders). 3. Nucleic Acids: DNA and RNA are biopolymers responsible for storing and transmitting genetic information. Biopolymers are renewable, biodegradable, and environmentally friendly, making them an attractive alternative to synthetic polymers derived from fossil fuels. They have diverse applications in fields like medicine (e.g., drug delivery systems), agriculture (e.g., biodegradable mulch films), packaging (e.g., bioplastics), and textiles. The study and development of biopolymers contribute to sustainable materials and green technologies. Biopolymers have diverse applications across various industries due to their renewable, biodegradable, and eco-friendly properties. Some of their key uses include: 1. Medical Applications Drug Delivery Systems: Biopolymers like chitosan and alginate are used to develop controlled drug release systems. Tissue Engineering: Collagen and gelatin are employed as scaffolds for cell growth in regenerative medicine. Wound Dressing: Biopolymers such as hyaluronic acid and chitosan aid in wound healing due to their biocompatibility. 2. Agriculture Biodegradable Mulch Films: Used for soil coverage, these films decompose naturally, reducing plastic waste. Fertilizer Encapsulation: Biopolymers control the release of nutrients into the soil. 3. Food Industry Edible Films and Coatings: Polysaccharides and proteins like gelatin and starch are used to preserve food freshness. Food Packaging: Bioplastic packaging materials like polylactic acid (PLA) replace conventional plastics. 4. Textiles Fibers and Fabrics: Biopolymers such as silk and wool are used for making sustainable clothing. 5. Environmental Applications Bioremediation: Biopolymers like starch are used to remove pollutants from water and soil. Waste Management: Biodegradable polymers help in reducing plastic pollution. 6. Cosmetics and Personal Care Biopolymers like hyaluronic acid are included in skincare products for hydration and anti-aging benefits. 7. Packaging Biopolymers are used to manufacture compostable and biodegradable packaging materials, reducing the dependency on petroleum-based plastics. These applications highlight the significant role of biopolymers in promoting sustainability and reducing environmental impact. Cellulose is a natural biopolymer and the primary structural component of plant cell walls. It is a carbohydrate composed of glucose monomers linked by β-1,4- glycosidic bonds, forming long, linear chains. As the most abundant organic polymer on Earth, cellulose is renewable, biodegradable, and environmentally friendly. Key Properties: High tensile strength, making it ideal for structural support in plants. Insoluble in water due to its crystalline structure. Can be chemically modified to produce derivatives like cellulose acetate and carboxymethyl cellulose. Uses: Paper and Textiles: Cellulose is the main raw material for paper production and fabrics like cotton. Food Industry: Used as a thickener, stabilizer, and dietary fiber. Bioplastics: Serves as a base for biodegradable plastics. Medical Applications: Used in wound dressings and drug delivery systems. Cellulose plays a critical role in sustainability due to its abundance and versatility in various industries. Carboxymethylcellulose (CMC) is a water-soluble derivative of cellulose, made by chemically modifying cellulose with carboxymethyl groups. This modification enhances its solubility and functionality, making it highly versatile. Key Properties: High water solubility. Forms viscous solutions, even at low concentrations. Biodegradable, non-toxic, and biocompatible. Uses: Food Industry: Used as a thickener, stabilizer, and emulsifier in products like ice cream, sauces, and beverages. Pharmaceuticals: Acts as a binder in tablets and as a component in gels, syrups, and wound dressings. Cosmetics: Used in lotions, creams, and shampoos for its thickening and stabilizing properties. Industrial Applications: Utilized in detergents, paper coatings, and oil drilling fluids. Its versatility and eco-friendliness make CMC an essential material across numerous industries. The preparation of Carboxymethylcellulose (CMC) involves the chemical modification of cellulose through etherification with chloroacetic acid under alkaline conditions. The process generally includes the following steps: Steps in the Preparation of CMC: 1. Cellulose Pretreatment: Purified cellulose (from sources like wood pulp or cotton) is dried and ground into fine particles to increase its surface area for reaction. 2. Alkalization: Cellulose is treated with a concentrated sodium hydroxide (NaOH) solution to swell the fibers and activate the hydroxyl groups in the cellulose. This step forms alkali cellulose. 3. Etherification: The alkali cellulose is reacted with sodium chloroacetate (or chloroacetic acid neutralized with NaOH) in the presence of heat. The carboxymethyl groups (-CH2-COONa) replace the hydrogen atoms in the hydroxyl groups of cellulose, forming sodium carboxymethylcellulose. 4. Neutralization and Purification: The reaction mixture is neutralized, and unreacted chemicals and by-products are removed by washing with alcohol or water. The product is filtered and dried. 5. Drying and Milling: The purified CMC is dried and milled into a powder form for various applications. Key Factors in the Process: The degree of substitution (DS) (i.e., the number of hydroxyl groups substituted) determines the solubility and viscosity of the CMC. A DS of 0.6 to 1.0 makes CMC water-soluble, suitable for most applications. This process results in a versatile, water-soluble polymer with wide-ranging industrial and commercial uses. Chitosan is a natural biopolymer derived from chitin, which is found in the exoskeletons of crustaceans (e.g., crabs, shrimp) and the cell walls of fungi. It is produced by the deacetylation of chitin, where acetyl groups are removed to yield a more soluble polymer. Key Properties: Biodegradable, biocompatible, and non-toxic. Soluble in acidic solutions. Antimicrobial and antioxidant properties. Uses: 1. Medical: Wound dressings, drug delivery systems, and tissue engineering. 2. Agriculture: Plant growth enhancer and seed coating material. 3. Water Treatment: Removes heavy metals and dyes due to its chelating ability. 4. Food Industry: Preservative and fat-binding agent. 5. Cosmetics: Used in moisturizers and anti-aging products. Its eco-friendly nature and versatile applications make chitosan a valuable material in various industries. The preparation of chitosan involves the chemical processing of chitin, which is extracted from natural sources such as the shells of crustaceans. The process generally consists of the following steps: Steps in the Preparation of Chitosan: 1. Raw Material Collection and Cleaning: Crustacean shells (e.g., shrimp or crab) are washed thoroughly to remove impurities such as proteins and minerals. 2. Deproteinization: The shells are treated with a dilute alkali solution, typically sodium hydroxide (NaOH), at high temperatures to remove proteins. 3. Demineralization: The deproteinized material is treated with a dilute acid, such as hydrochloric acid (HCl), to dissolve calcium carbonate and other minerals, leaving behind chitin. 4. Deacetylation: Chitin is heated with a concentrated alkali solution (e.g., NaOH) under high temperatures to remove acetyl groups, converting it into chitosan. The degree of deacetylation (typically 60–90%) determines the solubility and properties of chitosan. 5. Washing and Drying: The resulting chitosan is washed with water to neutralize and remove any residual chemicals. It is then dried and ground into a fine powder. Factors Influencing the Process: The temperature, concentration of NaOH, and reaction time during deacetylation significantly impact the final product's quality and properties. This process yields chitosan, a versatile biopolymer widely used in medicine, agriculture, water treatment, and more. The chemical structure of chitosan is derived from chitin, with the primary difference being the removal of acetyl groups. Chitosan is a linear polysaccharide composed of repeating units of glucosamine (GlcN) and N- acetylglucosamine (GlcNAc). Chemical Structure of Chitosan: The basic structure of chitosan consists of: Glucosamine (GlcN) as the primary monomer unit. N-acetylglucosamine (GlcNAc) is present in the polymer if the degree of deacetylation is not complete. The repeating structure of chitosan can be represented as: (C6H11NO4)n, where n is the number of repeating units in the polymer chain. The structure can be shown as: Glucosamine: N-acetylglucosamine: The structure of chitosan is as follows: In the deacetylated form, the structure mainly consists of GlcN units connected by β-(1→4) glycosidic bonds. The degree of deacetylation (DD) determines the proportion of GlcN and GlcNAc units. The higher the degree of deacetylation, the more GlcN units there are. Simplified structure (without detailed chemical formulas): The presence of GlcN (with an amine group) gives chitosan its solubility in acidic solutions, while the GlcNAc (with an acetyl group) contributes to the natural structure of chitin.