Biomolecular Enzymes .gdoc
Document Details
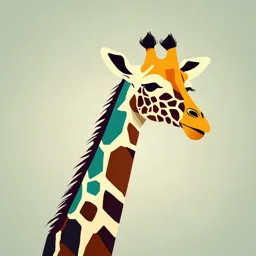
Uploaded by SmilingJasper2616
UCD
Full Transcript
Lecture 1 - Enzymes Important names in enzymes Pasteur - fermentation Hirs, Moore, Stein - ribonuclease Kuhne - enzyme word sequence Buchner - yeast ethanol Philips - x ray structure Michaelis + Menton - kinetic...
Lecture 1 - Enzymes Important names in enzymes Pasteur - fermentation Hirs, Moore, Stein - ribonuclease Kuhne - enzyme word sequence Buchner - yeast ethanol Philips - x ray structure Michaelis + Menton - kinetics 1975 - Restriction enzymes + ligase to Sumner - enzyme is a protein clone 80’s - Taq polymerase for DNA amplification Enzymogolgy Metabolic + cellular processes Drug targets (inhibit enzyme action), disease (eg PKU lacking enzyme for phenylalanine -> tyrosine) Industrial applications : Detergents, textiles, food manufacturing, paper Biological catalysts - increase rate without taking part 10^15 times faster than uncatalysed Proteins + ribozymes with active site for substrate to find for product formation Highly specific for substrate and reaction Most abundant enzyme = RuBisCO (lyase) = carbon fixation in plants IUBMB Classification Type of reaction - 4 components a. Type of reaction, b. Subclass for substrate, c. Bond catalysed, d. Reaction Six classes 1. Oxidoreductase - catalyse redox reaction Eg. Dehydrogenase, oxidase, peroxidase 2. Transferase - catalyse functional group transfer Eg. Kinase, transaminase, polymerase 3. Hydrolase - catalyse cleavage of bonds via water addition Eg. protease, nuclease, alkaline phosphatase 4. Lyase - catalyse breaking bonds Eg. decarboxylases 5. Isomerase - catalyse rearrangement of atoms -> isomer 6. Ligase - catalyse joining of molecules using ATP energy Isoenzymes Different forms of an enzyme within a species that catalyse the same reaction, have similar amino acid sequences and a common origin But may have differences in sequence, structure, metabolism, substrate, Makes enzyme better suited to unique site of action - allow for certain kinetic / regulatory properties, cellular or tissue specificity, substrate specificity Eg. Lactate dehydrogenase for anaerobic respiration 4 Muscle and Heart subunits combine in any way to form -> 5 isoenzymes possible HHHH, HHHM, HHMM, HMMM, MMMM Function : difference in regulation ie. LDH1 = heart LDH5 = skeletal muscle Structure of enzymes Globular proteins - hydrophobic interior, hydrophilic exterior Active site = cleft of amino acid residues where substrate binds and interacts with hydrophobic interior help to make and break bonds = catalytic groups Lecture 2 - Enzymes Cofactor = non-protein component that allows enzyme to function Apoenzyme + cofactor -> holoenzyme Cofactors = coenzymes + inorganic metal ions eg Cu2⁺, Zn2⁺ etc Coenzymes Organic molecules derived from vitamins weakly bound to enzyme Can act as co-substrate -> part of product Eg. NAD⁺ in dehydrogenases and FAD in flavoenzymes Vitamins cannot be synthesised by humans but are precursors for many enzymes Vitamin Niacin B3 Nicotinamide + ribose + Adenosine diphosphate -> NAD⁺ Nicotinamide + ribose + Adenosine triphosphate -> NADP⁺ Nicotinamide is reduced (gains H) to form NADH / NADPH Other substrate involved is oxidised Usually associated with dehydrogenase enzymes Eg. alcohol dehydrogenase + NAD⁺ + ethanol -> acetaldehyde + NADH Enzyme Assays Show production of product / depletion of substrate over time 1. Spectrometer / fluorometer if substrate and product have different absorbance / fluorescence eg NADH absorbs light @ 340nm not NAD⁺ 2. Colorimetric substrate - changes during reaction with enzyme 3. Coupled enzyme assays - link one enzymatic product to another enzyme 4. Radiolabelling of product - detection via autoradiography Eg. coupled enzyme assay - Hexokinase Glucose + ATP + Hexokinase -> Glucose-6-P -> Glucose-6-P + G6P hydrogenase + NADP⁺ -> 6 phosphogluconate + NADH IU units One IU = amount of enzyme needed to catalyse conversion of 1µmol of substrate per minute at 25 ℃ Initial rate = v fast then tapers out due to limiting reagents / saturation Benefits of enzymes Mild conditions needed, highly specific, catalytic power, easily regulated Physical conditions Temperature, pH and pressure must be optimal Eg N2 + 3H2 -> 2NH3 Nitrogenase enzyme needed 20 degrees, pH7, 1atm Without nitrogenase we need 700 degrees, iron catalyst and 900 atm Much more energy and cost efficient pH optimum Depends on state of enzyme / substrate Extreme pH -> denature Pepsin = pH2, Chymotrypsin = pH 7.5, Arginase = pH 10 Temperature optimum 10 ℃= x 2 activity But denatures at too high - Optimum usually 40 ℃ Thermophilic = survive high temp eg taq polymerase withstands 95 ℃for PCR Psychrophiles = optimum below 15 ℃ Mesophiles = optimum around 40 ℃ Specificity Catalyse one type of reaction for one group of molecules - complementarity of active site. Can display >1 type of specificity Substrates bind via VDW, ionic interactions, H bonding, hydrophobic interactions Usually anabolic enzymes more specific than catabolic eg. polymerases (Except restriction enzymes - they are v specific) DNA Pol uses soluble active site to catalyse DNA synthesis has four specificities to ensure correct base pairing Fidelity = accuracy of DNA replication Lock and Key hypothesis - specific substrate key binds to active site lock - no change in structure / flexibility Induced fit model - active site is flexible and undergoes conformational change to perfectly suit the substrate Transition state binding - point of bond make/break = point at which substrate has highest affinity for active site -> enzyme is most stable and favours this state -> drives reaction Serine proteases - differ in region where they cut amino acid chains due to specififcty of side chain interaction Trypsin cleaves after basic aas eg Arg and Lys, Chemotrypsin cleaves after aromatic aas eg. Phe and Tyr Elastase cleaves after small non polar aas eg. Gly Stereospecificity = ability of an enzyme to selectively catalyse the reaction of one stereoisomer over another - only one enantiomer of chiral drugs is therapeutically active Absolute specificity = enzyme catalyses one reaction eg maltase Group specificity = enzyme acts on one kind of functional group eg tyrosine kinase Linkage specificity = enzyme acts on type of chemical bond eg amylase cleaves 1->4 bond Stereochemical specificity = enzyme acts on specific isomer eg D amino acid oxidase Catalytic power Increase reactions by 10^12 Activation energy = energy input needed for transition state to be reached Enzyme favours the transition state, providing an alternative pathway with a lower activation energy barrier Don’t change the overall thermodynamics Mechanisms of actions ( can have >1) 1. Proximity Enzyme active site as template to bring reactants closer together + rotate favourably - loss of entropy, increase in likelihood of effective collsion. Not relevant for isomerases 2. Acid-base catalysis Enzyme side chains can be weak acids and bases and accept / donate protons to facilitate reaction by stabilising transition states. Eg. Glu and Asp R-COOH -> R-COO- EG. Glutamic acid in lysosome = general a-b catalysis Concerted a-b catalysis = eg RNase one amino acid = acidic, other amino acid = base 3. Covalent catalysis Amino side chains form temporary covalent bonds with substrate via nucleophilic attack, making stable intermediate that is easier to bring to transition state Eg. Cys, Ser, His. 4. Transition state stabilisation Active site promoted transition state intermediate Normal transition state v unstable bc energy is at peak Stable analogous transition states bind to enzymes tighter than substrates 5. Electrostatic effects Active site excludes water allowing electrostatic stabilisation through favourable charge distribution. Charge of enzyme can be used to balance other charges 6. Metal ion catalysis 30% metalloenymes where 2+ metal ion is held in place by amino acid residues and positive charge helps stabilise 3 Case Studies - Carbonic anhydrase, lysozyme, chymotrypsin In RBCs - Maintains pH of blood CO2 transported to lungs for excretion. Some bound to hemoglobinas most as bicarbonate ion HCO3-. Active site Zn²⁺ that stabilises negative charge and polarizes water molecule. The enzyme binds a water molecule at the active site. Zn2+ lowers the pKa of the water -> deprotonated easier -> H+ and OH-. OH- attacks the carbon dioxide -> HCO3- and H⁺. Bicarbonate ion released, proton maintains pH. Lysozyme Has general acid catalysis, electrostatic catalysis and ts catalysis Glutamic acid donates a proton to O of glycosidic bond between sugar 4 and 5 of 6 sugar chain bound to active site (acid-base catalysis). Forms positively charged transition state sugar in half chair conformation. Positive charge balanced by Asp residue (electrostatic catalysis). Transition state has higher affinity for enzyme active site. Glyxosidic bond breaks via hydrolysis - Glu accepts H+ and OH- added to sugar #4. Chymotrypsin Catalysis based on active site serine residue -> Hydrolysis of polypeptides Catalytic triad = charge relay system = 3 free amino acids Ser, His, Asp Aspartic acid side chain takes protein from histidine side chain which takes protein from serine -> tetrahedral TS Chemotrypsin mechanism Polypeptide binds to hydrophobic active site. His takes proton from serine -> highly reactive tetrahedral transition state of Ser -> O- binds to polypeptide. C terminal of polypeptide accepts H+ and is released. N terminal of polypeptide bound to Ser Water binds to His instead of C terminal + donates H+ ion to His and OH- ion to N terminal. Peptide is released. Ser attacks peptide bond -> Negative tetrahedral TS His donates H+ to N of peptide bond -> free peptide leaves and N remains Covalent acyl enzyme intermediate Water -> H+ His addition, OH- attacks acyl enzyme -> new C terminus of new polypeptide Enzymes Lecture 4 - Kinetics + inhibition As substrate increases, rate also increases until saturation point where rate becomes independent of substrate k1 k2 E + S ES -> E + P k-1 Assumptions of M-M Only one substrate that reversibly binds to enzyme, No reverse reaction once ES becomes product, the substrate conc is far higher than the enzyme concentration (not limiting reagent) ES in equilibrium with E ie. conc of ES is constant and rate of formation is equal to rate of breakdown M-M Equation expresses rate as a function of substrate conc Vmax = highest rate of reaction when all E is present as ES (saturated) Km = conc of substrate at half of Vmax When S > Km -> v = Vmax - saturated When S < Km -> v proportional to S - S is limiting reagent When S = Km -> v = ½ Vmax Finding Km and Vmax - Lineweaver-Burk 1/V = Km/Vmax x 1/S + 1/Vmax Lower Km = better bc reaction can work with small amount of substrate due to high enzyme affinity. Higher Km = lower affinity as more substrate is needed to reach half max point Higher Vmax = Fast reaction rate Turnover number = Kcat - explains efficiency of the enzyme Maximum number of substrate molecules that one enzyme can convert to product per unit time when fully saturated Kcat = Vmax / Enzyme conc (sec-1) High Kcat = more efficient as more molecules are converted to product Specificity constant ratio : Kcat / Km (M/s) High value = efficient enzyme at making product as can achieve higher product yield (Kcat) with lower affinity (Km) Low value = less product produced or a low affinity for substrate Eg. chymotrypsin Glycine = 1.3x10-1 M^-1 s^-1 but phenyalanine = 1x10^5 M/s^-1 Enzyme inhibition Inactivates the ES complex Reversible : Competitive substrate and competitor bond at the same site (E) + inhibitor (I) -> EI Non competitive = inhibitor doesn’t bind to active site but reduces enzyme - substrate affinity Can bind both at same time E + I + S -> EIS v inactive form Uncompetitive inhibition = inhibitor will only bind when substrate is present in active site Competitive inhibitor No change in Vmax as if enough substrate is present the inhibitor can be overcome Increase in Km as more substrate is needed to reach ½ Vmax Inhibitor constant Ki = potency of inhibitor ie. Conc required to reduce enzyme efficiency by half Drug + Receptor -> DR -> effect IC50 = conc of drug required to reduce efficiency by 50% - potency of inhibitor EC50 = conc of drug that causes 50% of max response - potency of drug Most drugs = competitive inhibitors Eg. methotrexate inhibits dihydrofolate reductase -> reduce nucleotide synthesis Eg. Saquinavir inhibits HIV protease -> reduce viral replication Irreversible inhibitors Covalent bond to amino acid in active site -> inactivated Eg. DIFP binds to serine in active site - serine protease digestion inhibited Eg. Sarin = v potent acetylcholinesterase inhibitor -> muscle spasms Eg. Aspirin = inhibits cyclooxygenases -> pain + inflammation pathway blocked Penicillins Beta lactam antibiotics Inhibit transpeptidase -> peptidoglycan synthesis stops No peptidglycan = weak bacteria -> collapse when try to divide Eukaryotic cells unaffected as no peptidoglycan Lecture 5 - Enzyme Regulation Regulate amount made by gene expression + activation of precursor Regulate activity using inhibitors, modifications and allosterism 1. Gene expression Levels of substrate / product dictates enzyme synthesis Eg tryptophan synthesis in bacteria - operator + repressor protein High Trp -> repressor binds + enzyme synthesis terminated Low Trp -> enzyme production resumed Eg. Lactase synthesis Lactose + lactase enzyme -> glucose + galactose Decrease in consumption of lactose after weaning -> decrease in transcription factor for lactase gene -> struggle to digest dairy 2. Activation of precursor Zymogen = inactivated digestive enzymes cleaved to become active This is to prevent self digestion as they make their way to site of action (pancreas -> duodenum) Eg. chymotrypsinogen (inactive) cleaved into active α-chymotrypsin Eg. Damage -> blood clotting cascade where each factor activates another until thrombin cleaves fibrinogen -> fibrin -> blood clot. Allows for control and amplififcation 3. Covalent Modification Eg. phosphorylation of Ser, Thr or Tyr residues by protein kinases + removal by phosphatases (large change in polarity as PO4 is v negative) Eg glycogen synthase phosphorylated -> inactive Glycogen phosphorylase phosphorylated -> active Phosphorylation cascades allow for amplification of signals Insulin activates the protein phosphatase enzyme for dephosphorylation while adrenaline activates the protein kinases (Phosphorylation) 4. Inhibitors Often feedback inhibition where product of reaction inhibits the synthesis of more product to prevent unessecary syntheis Allosteric control Forms sigmoidal curve = exception to MM equation (hyperbolic curve) Enzyme has many subunits -> one ligand binding has co-operative effect on the binding of catalytic substrate Allow shifting between tense and relaxed state 1st substrate can be an inhibitor or activator which changes conformation of catalytic active site Eg. Aspartate Transcarbamoylase Carbamoyl phosphate + aspartate -> CTP ATP = + allosteric effector. High ATP = sufficient energy -> conversion of ATCase to the R state, enhancing pyrimidine synthesis. CTP (cytidine triphosphate), the end product = negative allosteric effector. CTP binds to regulatory subunits, + stabilizes T state of ATCase, reducing enzymatic activity. Eg. Sildenafil = viagra = inhibit cGMP to allow relaxation Strychnine = poison = inhibt acetylcholine receptors Multifunctional Enzymes >1 catalytic activity on one polypeptide chain Eg. purines need dehydrogenase, hydrolase, and synthase activity of one enzyme Eg. fatty acids = 7 enzyme functions Multienzyme complex = non-covalent mass of enzymes + coenzymes Allow channeling of products between enzymes without having product leave the enzyme complex - efficient bc unstable intermediates quickly changed Metabolic complexity = Many small steps allow speed as each enzyme only acts on one specific metabolite. Also allows fine-tuned regulation and multiple pathways for intermediates