Gait Analysis PDF
Document Details
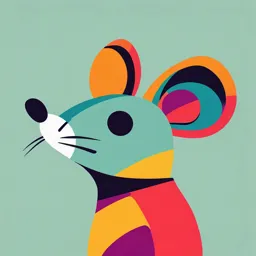
Uploaded by FunByzantineArt
Tags
Summary
This document provides a detailed overview of gait analysis, including definitions of normal gait, physiological and mechanical aspects. It covers the key phases within the gait cycle, initial contact, midstance and terminal stance, and swing phase. The text is well-structured with clear explanations and is likely intended for students or professionals in a health-related field.
Full Transcript
Gait analysis Gait is the medical term to describe human locomotion, or the way that we walk. Interestingly, every individual has a unique gait pattern. A person’s gait can be greatly affected by injury or disease process. By evaluating the gait pattern of an individual, a therapist can determine s...
Gait analysis Gait is the medical term to describe human locomotion, or the way that we walk. Interestingly, every individual has a unique gait pattern. A person’s gait can be greatly affected by injury or disease process. By evaluating the gait pattern of an individual, a therapist can determine specific weaknesses and adjust rehabilitation programs to address these issues. Definition of normal gait Physiological Definition: It is a mechanism which depends upon closely integrated action of the subjects, bones, muscles and nervous system (including peripheral and central nervous system) The degree of integration determines the different gait patterns. Any defect of any part of them or all of them will lead to pathological gait. Mechanical definition: It is a form of bipedal locomotion as there is an alternating action between lower extremities. One leg is in touch with the ground for restraining, supporting and propulsion. The other leg is in swing phase for creating a new step forward. So, gait is the result of a series of rhythmic alternating movement of arms, legs, and trunk which create forward movement of the body. Prerequisites of gait: 1. The ability to support or assume upright position. (the ability to maintain head, arms, and trunk HAT against gravity) 2. The ability to maintain balance in an upright position during static and dynamic situation. 3. The ability to develop or create new step forward through controlling of foot movement to achieve safe ground clearance and gentle heel contact. N.B: HAT constitute 75% of total body weight. Phases of Gait cycle Phases of gait Gait cycle: is used to describe the complex activity of walking, or our gait pattern. This cycle describes the motions from initial placement of the supporting heel on the ground to when the same heel contacts the ground for a second time. At normal adult walking speeds, one cycle lasts 1 second and has a length of 1.4 meters. During one gait cycle, each extremity passes through two phases, a single stance phase and a single swing phase. I-Stance Phase: Is defined as the interval in which any part of the foot of one extremity is in contact with the ground (60% of the gait cycle) and it ends when the reference foot lifts off the ground. So, it is called “the supporting phase” or “weight bearing phase”. stance phase Stance Phase is divided into 5 phases: 1- Initial contact (heel strike): Initial contact is an instantaneous point in time only and occurs the instant the foot of the leading lower limb touches the ground. This phase occurs at about 0%- 2% of GC. The heel is usually the foot section that makes initial contact, but other parts of the foot may contact the ground first in the presence of some pathologic conditions. 2- Loading response (flat foot): The loading response phase occupies about 2%-10% of the GC and constitutes the period of initial double stance. During loading response, the foot comes in full contact with the floor, and body weight is fully transferred onto the stance limb. During this phase, the body’s impact forces with the ground are absorbed. 3- Mid stance Mid-stance represents the first half of single support, which occurs from the 10%- 30% of the GC. It begins when the contra-lateral foot leaves the ground and continues as the body weight travels along the length of the supporting lower limb until it is aligned over the forefoot. In this phase the body’s COM moves directly over the foot. 4- Terminal stance (heel off): Terminal stance constitutes the second half of single-limb support. It begins with heel of the reference foot rise and ends when the contra-lateral foot contacts the ground. Terminal stance occurs from the 30%- 50% of GC. 5- Pre-swing (toe off): Pre-swing occurs when only the toe of the reference limb is in contact with the ground. This phase called weight release or weight transfer. This phase occurs from 50%- 60% of GC. It is the terminal double stance interval. II-Swing phase: Is defined as the interval in which the foot of the reference limb is not in contact with the ground (40% of the gait cycle) and it ends just prior to heel strike of the same extremity. It denotes the time when the foot is in the air, so it is called “non- weight bearing period”. The swing period primarily is divided into three phases: Initial swing, mid-swing and terminal swing. swing phase 1- Initial swing (acceleration): It is the period from toe of the reference limb leaves the ground to the mid-swing of the reference limb. This phase is from 60%-73% of GC. 2- Mid-swing: It is period when the reference limb passes directly under the body. The reference limb reaches vertical tibial position just before the end of mid-swing. This phase is from 73%-87% of GC. 3- Terminal swing (deceleration): It is period from the end of mid-swing to the point just before initial contact. The knee is extending in preparation for new heel strike. This phase is from 87%-100% of GC. Intervals of gait cycle: Each gait cycle (stance and swing phase) is subdivided to intervals. I- Stance phase is subdivided into double limb support (initial double stance IDS, and terminal double stance TDS) and single limb support intervals: Intervals of gait cycle Double limb support (initial double stance and terminal double stance): Initial double stance: is the period when both feet are in contact with the ground. One foot is in initial contact and loading response and other foot is in pre-swing. Terminal double stance: in late stance, the ipsilateral foot is in preswing and contralateral foot is in initial contact and loading response. As velocity increases, double-limb support time decreases. Running constitutes forward movement with no period of double-limb support. In normal walking, initial double stance takes up about 10% of GC, also the terminal double stance takes up to 10% of GC. Single limb support SLS: is the period when only one foot is in contact with the ground (mid stance and terminal stance). In walking, this is equal to the swing phase of the other limb. It takes up to 40% of GC. II- Swing phase has one interval as one foot is not in contact with the ground and it takes up to 40% of gait cycle. Functional Tasks of Gait From a functional perspective, there are three fundamental tasks associated with human gait: weight acceptance, single limb support, and limb advancement. The stance phase plays a role in all three of these basic tasks, each of its sub-phases contributing to varying degrees. Initial contact and loading response are the two sub- phases primarily responsible for weight acceptance. Single limb stance occurs at midstance and is the time when balance during ambulation is most precarious. The body’s center of mass has shifted laterally and is centered over only one supporting limb at this time. Limb advancement creates forward motion of the body and includes the stance sub- phases of terminal stance and preswing; these sub-phases provide propulsive forces to move the limb forward and thereby move the body forward. The stance sub-phases utilize effective force absorption and efficient energy expenditure to accomplish these tasks. The swing phase is concerned with only one of the three fundamental tasks: limb advancement. Limb advancement during the swing phase requires sufficient clearance of the foot from the floor. The limb performs this activity during the first half of swing and prepares for initial contact during the latter half of swing. During the first two sub-phases of the swing phase, initial swing and midswing, the limb flexes at the hip, knee, and ankle to functionally shorten the limb so the foot clears the floor. The knee then begins rapid extension in terminal swing to lengthen the limb; this motion increases step length and forms a rigid limb in preparation for stability at initial contact. Analysis of gait Analysis of gait classified into: 1- Kinematic analysis 2- Kinetic analysis 1- Kinematic analysis: Kinematics is the science of motion. In human movement, it is the study of the positions, angles, velocities, and accelerations of body segments and joints during motion. It classified into 3 categories: Distance and time variables, Joint angles from sagittal and frontal plane for upper and lower limbs, and Determinants of gait 1- Distance and time variables: Distance variables (spatial parameters): Step length: It is the linear distance between the initial contact (measured at the midpoint of the heel) of one foot to the initial contact of the opposite foot. Step length Values for normal adult’s step length are: 64 cm average for women, 73 cm average for men, and the overall average is 70.5 cm. Stride length: It is the linear distance between two successive foot contact of the same limb. It consists of two step lengths, left and right, each of which is the distance by which the named foot moves forward in front of the other one. It is usually measured from the midpoint of heel Stride length Values for normal adult’s stride length are: 128 cm average for women, 146 cm average for men, and the overall average is 141 cm. However, stride length is not always twice the length of a single step because right and left steps may be unequal. Stride width: It is the horizontal linear distance between the midpoint of the heel of one foot and the same point of the other foot. Step width measures typically between 2.5 to 12.5 cm for adults with the average of 8 cm; this standard does not hold true in some pathological conditions. Degree of toe out: It is the angle formed by each foot’s line of progression and a line intersecting the center of heel and the second toe. Out-toeing of about 7° is typical in mature adults. The degree of toe out decreases as the speed of walking increases in normal adults. Degree of toe out Time variables (Temporal parameters) Step time: it is time spent during single step between heel strike of one leg and heel strike of the other leg. Stride time: It is the time interval between successive instant of foot floor contact of the same foot. (i.e. it is the amount of time it takes to complete one stride). Stance time: It is the time that passes during the stance phase of one limb in a gait cycle. Swing time: It is the time while the foot is not in contact with the floor. (0.4 sec.) Single limb time: it is the time when only one limb is on contact with the supporting surface. Double limb time: it is time spent with both feet on the ground during one gait cycle. It decreases as the speed of walking increases. Swing / stance ratio: It is the ratio between swing time and stance time Cadence: It means number of steps per minute. (110 steps /min) Slow: 60 – 70 Steps / min. Medium: 80 – 90 Steps / min. Fast: 120 Steps / min. A shorter step length will result in an increased number of steps at a constant distance. As one walks with increased cadence, the duration of the double limb stance decreases. Speed: It is distance / time. - In male: 89 meter/min. - In female: 74 meter/min. (45 cm / sec) 1- Measurement of joint angles The kinematics, or “geometry,” of walking has been studied and recorded, looking at the angular movements of each body segment in all three planes. Advances in photographic techniques and computer technology have permitted more accurate study from a three-dimensional perspective. Since the sagittal plane motions are the largest and easiest to track, they have been most often investigated. On the other hand, displacement in the frontal and transverse planes is smaller and more difficult to measure; therefore, results are less accurate and reliable and more divergent and inconsistent. Sagittal Plane kinematics In the sagittal plane, the two vertical oscillations of the body’s COM follow a smooth sinusoidal curve. The curve’s highest point occurs at midstance for each foot while the lowest segment of the sinusoidal curve is at double support. PELVIS The pelvis must multifunction during gait, providing both stability and mobility to upper and lower extremities. The pelvis offers a stable base of support for the lower limb and HAT but it also must allow for the contributions of thoracic and lumbar spine motions. Additionally, the pelvis must be stable enough to transmit weight as it transfers from one limb to the other, and it also needs to move the acetabulum in a favorable position for hip motion. In the sagittal plane, the pelvis remains relatively level, demonstrating an average anterior-posterior tilt excursion of only about 3° during the gait cycle. Two full cycles of sinusoidal motion occur with each step; the pelvis reaches initial contact in a near-neutral position and moves through midstance in a slightly posteriorly tilted position. By the end of midstance when the hip begins moving into extension, the pelvis tilts anteriorly just slightly. By the time the limb reaches preswing, the pelvis tilts posteriorly again. During swing, the pelvis first completes its posterior tilt and then tilts anteriorly from initial to midswing, and in terminal swing it moves toward a posterior tilt in preparation for landing once again. Hip joint The hip joint moves through 40° of sagittal motion in a single gait cycle. At initial contact, the hip is flexed at 25° to 30°.As the stance phase continues, the hip progressively moves into extension, reaching its maximum extension at 10° of hyperextension by terminal stance. From initial contact to midstance, the trunk moves forward over the foot and continues its forward movement to advance in front of the foot immediately after midstance. Hip hyperextension at terminal stance is accompanied by extension of the lumbar vertebrae and an anterior pelvic tilt. Once the hip reaches maximum extension at terminal stance, the hip begins movement towards flexion during preswing as it prepares to lift the limb off the ground; by the end of stance, the hip is near neutral, and as the limb begins into the swing phase, the hip continues a steady increase into flexion until midswing when the hip is slightly more than 30° of flexion. After midswing, its flexion decreases slightly and is maintained until terminal swing when it starts to move towards extension in preparation for initial contact. These typical sagittal plane arcs that occur at 10° of hyperextension (at terminal stance) and at 30° of flexion (at midswing) occur during normal walking speed but are slightly higher in fast walking. Clinical note: because pelvis and lumbar spine motions are mechanically linked at sacroiliac joint, exaggerated pelvic tilting during walking may increase the stress at lumbar spine. These stresses could eventually irritate the structures within this region resulting in low back pain Summary of hip joint: Hip Stance phase Swing phase joint IC LR MSt TSt * PSw Isw MSw * TSw 30º 25º 0º 10º 0º 20º 30º 30º flexion Flexion hyperextension flexion flexion flexion TSt: hyperextension arc, MSw: flexion arc Note: hip extension arc acts to transfer weight to contralateral limb, while hip flexion arc acts to clear foot from the ground. Knee joint The knee joint moves through two cycles of flexion and extension during the gait cycle. At initial contact, the knee is near full extension (about 3° to 5° of flexion). Immediately following initial contact, the knee flexes during loading response. This flexion movement provides shock absorption of compression forces that occur when the body weight impacts the ground, and it also assists in weight transfer as the body begins to move toward a single leg support stance. By the end of the loading response phase, the knee is flexed 15° to 20°.The knee then moves towards extension during midstance; this motion minimizes muscular effort required during single limb weight-bearing. Maximum extension at the knee occurs during terminal stance. Immediately after achieving full extension, the knee starts to flex to achieve its maximal stance-phase flexion at 35° to 40° by preswing. During initial and midswing, the knee flexes to shorten the limb and clear the foot from the floor. Maximum knee flexion of the entire gait cycle occurs during midswing, with the knee at 60° to 65° of flexion. During terminal swing, the knee extends to nearly full extension, so it is able to assist the other limb joints in achieving an adequate step length, advancing the limb, and preparing for initial contact once again. Summary Knee Stance phase Swing phase joint IC LR * MSt TSt PSw Isw MSw * TSw * * 5º 15-20º 0 or 5º 0º 35-40º 40-60º 60-65º 0º flexion Flexion flexion flexion flexion flexion LR: the 1st arc of knee flexion, TSt: the 1st arc of knee extension, MSw: the 2nd arc of knee flexion, TSw: the 2nd arc of knee extension Note: first knee flexion arc acts as a shock absorption, while 2nd knee flexion arc acts to clear foot from the ground First knee extension arc acts to transfer weight to contralateral limb, while 2 nd knee extension arc acts for preparation for new step. Ankle joint and foot The ankle plantarflexes and dorsiflexes twice during the gait cycle. At the moment of initial contact, the ankle joint is in sagittal plane neutral. During loading response, the ankle rapidly plantarflexes (15º planterflexion) so the entire plantar foot surface contacts the floor. After loading response, the tibia advances forward over the fixed foot into midstance, so by the end of midstance the ankle is in about 5-10° of dorsiflexion. At terminal stance 10º of ankle dorsiflexion is the close-packed position of the ankle and provides maximal talocrural joint congruency. As the heel rises off the floor during terminal stance, a second wave of plantarflexion occurs, peaking at about 20°-30º by preswing. This plantarflexion motion propels the limb into swing phase then planter flexion starts to decrease till 10º at initial swing. The foot dorsiflexes to neutral or slight dorsiflexion to clear the toes from the floor and maintains this position throughout the midswing and terminal swing. An essential but often overlooked sagittal plane motion occurs in the metatarsophalangeal joints (MTP) during the stance phase after midstance to preswing. During loading response, the MTP joints are in a neutral position. The MTP joints hyperextend as the body’s COM moves forward of the weight-bearing limb and the ankle starts to plantarflex. Extension becomes most apparent during terminal stance until the MTPs reach their maximum motion,55° to 60°, by the end of preswing. During the swing phase, hyperextension of the MTP joints is maintained at about 30° to 40°35 for toe clearance but gradually decreases to 25° by the time of initial contact. Summary Ankle Stance phase Swing phase joint IC LR* MSt TSt* PSw* Isw MSw* TSw 0º 15º 5-10º 10º 20-30º 10º 0º 0º neutral planter dorsiflexion dorsi planter planter Flexion flexion flexion flexion LR: 1st planterflexion arc, TSt: 1ST dorsiflexion arc, PSw: 2nd planterflexion arc, MSw: 2nd dorsiflexion arc Note: 1st planterflexion arc acts as shock absorption, 2nd planterflexion arc act for propulsion. 1st dorsiflexion arc act for transfer weight to the contralateral limb, 2nd dorsiflexion arc act to clear foot form ground and preparation for new step Frontal Plane kinematics Pelvis The pelvis tilts laterally in the frontal plane about 8° on each side. In unilateral stance, the pelvis of the swing leg tilts laterally downward about 8°. This lateral drop occurs on the swing leg since the stance leg adducts. This small but important motion serves two purposes: 1-It puts the hip abductor muscles of the stance leg on a slight stretch, thereby putting them at an improved length tension advantage 2-It reduces the rise of the center of mass of the swing leg. This reduction in the limb’s COM elevation was initially thought to be important in decreasing energy expenditure. Hip joint: Hip motions in the frontal plane directly impact pelvic motion. Frontal plane hip motions offer crucial contributions to stability and efficiency during gait. At initial contact, the hip is adducted to about 10° and continues to adduct another 5° during loading response, serving to puts the gluteus medius on the stance side on slight stretch. This position places the gluteus medius on stretch so it may generate the forces needed to stabilize and hold the contralateral pelvis level during unilateral stance. From midstance through terminal stance, the thigh moves into a relatively neutral position. The hip abducts about 5° during swing phase to assist in clearing the foot from the floor and returns to neutral as it approaches the end of terminal swing. Knee joint: At initial contact the knee is in slight abduction and moves to its maximum of about 3° of abduction during initial contact. During the swing phase, the knee moves into adduction to a maximum of about 8° N.B: Total abduction-adduction motion of the knee is minimal because of the stable collateral ligaments supporting the joint. Ankle joint and Foot Subtalar Joint Subtalar joint motions of inversion and eversion occur during gait. The subtalar joint is in slight inversion at initial contact and immediately moves into eversion after making contact with the ground. The subtalar joint rapidly moves through to its maximum range of eversion, about 5°, after loading response and by the time the limb is in early midstance. At midstance, the subtalar joint begins moving toward inversion. By preswing, it is at its maximum inversion position, approximately 8° to 11°. During the swing phase, the subtalar joint returns to neutral and is in slight inversion by the time the limb is in terminal swing. Midtarsal Joint As does the rest of the foot, the midtarsal joint follows the motions of the subtalar joint during all phases of gait. The two joints comprising the midtarsal joint, the talonavicular and calcaneocuboid joints, each have approximately 11° to 15° of total motion in the frontal plane during normal gait cycle. Immediately after initial contact, the midtarsal joint flattens to absorb impact forces and to allow the entire foot to touch the ground. Once in midstance, the arch elevates, following the inversion movements of the midtarsal and subtalar joints, to move the forefoot joints into more congruent positions in preparation of terminal stance and preswing. Transverse Plane kinematics Rotations within the transverse plane, around vertical axes occur at the vertebrae, pelvis, hips, knees, and ankle and feet. These rotations are observed during walking with the smooth reciprocal motions observed of the trunk and extremities. Pelvis: The pelvis rotates anteriorly on the swing side 4° and rotates posteriorly on the stance side another 4°—producing a total of 8° of pelvic movement in the transverse plane. The magnitude of this pelvic rotation increases 10°to 20°with increased speed. Maximum amount of anterior pelvic rotation occurs at initial contact in concert with maximum hip flexion (30°); these motions work cooperatively to provide a longer stride. Hip joint: During each stride, the limb moves through an arc of both medial and lateral rotation in the transverse plane. At initial contact, the hip is in slight lateral rotation, supported by the protracted position of the pelvis. Immediately after loading response, however, the hip medially rotates and maintains a medially rotated position through loading response and midstance until the hip moves into extension. From midstance to terminal stance, the hip moves to neutral rotation and continues into lateral rotation at preswing. During initial swing, the hip is in lateral rotation and oscillates between medial and lateral rotation until it returns to slight medial rotation as the limb prepares for ground contact. Peak hip medial rotation occurs at the end of loading response and maximum lateral rotation occurs at the end of preswing. Studies have shown that the total hip rotation ranges between 8° and 14° for normal adults. Knee joint: Throughout the entire gait cycle, the knee rotates a total of about 10° to 20°. Of this total rotation, the femur rotates on the tibia 6° to 7° medially, and laterally and the tibia rotates 8° to 9° in each direction. At initial contact, the knee is in slight lateral rotation (tibia relative to the femur) but then medially rotates as the limb accepts the weight. As the foot pronates during loading response, the tibia medially rotates about 8°, allowing the knee to flex. After midstance, the femur and tibia begin to rotate laterally in the transverse plane through preswing. When body weight is shifted to the other leg in preswing, the tibia is led into lateral rotation by the supinated foot and the limb moves into swing. During swing, the thigh, knee, and leg move toward and into medial rotation until terminal swing, when they laterally rotate in preparation for initial contact. Ankle and Foot Midfoot rotation in the early part of the stance phase is essential for shock absorption and allows the foot to adapt to uneven surfaces as the foot moves into loading response. At midstance, the tarsal joints rotate into supination to transform the foot to a rigid lever that permits propulsion of the body forward as the foot pushes against the ground. Trunk and upper extremity kinematics The trunk and upper extremities play an important role in maintaining balance and minimizing energy expenditure during gait. In addition, small intricate spinal movements and muscular actions at the trunk serve to dampen the gait-related oscillations and accelerations produced by the movement of the lower extremities. As a result, accelerations of the head segment are 10% to 40% less than those of the lower portion of the trunk. This dampening effect, keeping the head more stable, serves an important role in optimizing visual and vestibular function during gait. Head and Trunk During normal gait the head and trunk travel as one unit. Throughout the gait cycle the head, arms, and trunk (HAT) and center of gravity (COG) deviate from the mean line of progression in all three planes. Displacement pattern is a sinusoidal curve. So, the HAT and COG displace twice in upward direction and twice in downward direction in sagittal plane. The displacement in lateral direction in horizontal plane is once ipsilateral on right lower limb and once contralateral on left lower limb. The peak downward deviation occurs in loading response and in preswing. The peak upward deviation occurs in midstance and late midswing. Shoulder joint In the sagittal plane the shoulder exhibits a sinusoidal pattern of movement that is out of phase with hip flexion and extension. As the hip (femur) moves toward extension, the ipsilateral shoulder (humerus) moves toward flexion, and vice versa. At heel contact, the shoulder is in its maximally extended position of approximately 25º beyond the neutral position. The shoulder then progressively rotates forward to reach a maximum of 10º of flexion by 50% of the gait cycle. In the second half of the gait cycle, as the ipsilateral hip moves forward toward flexion, the shoulder extends to return to 25º of extension by the next heel contact. The pattern of movement of the shoulder is consistent across individuals, although the magnitude of movement varies greatly. In general, the amplitude of shoulder movement increases with greater speed. The major function of arm swing is to balance the rotational forces in the trunk. Restriction of arm motion has not been shown to have a significant effect on the energy cost of ambulation. Elbow joint The elbow is normally in approximately 20º of flexion at heel contact. As the shoulder flexes in the first 50% of the gait cycle, the elbow also flexes to a maximum of approximately 45º. In the second half of the gait cycle, as the shoulder extends, the elbow extends to return to 20º of flexion. Kinetic analysis of gait It is the analysis of forces acting on body during gait. Forces of gait include internal and external forces. External forces are: inertia, gravity, and ground reaction force (GRF). Internal forces: are primarily by muscles. The ligaments, tendons, joint capsules, and bony components assist the muscle by resisting, transmitting and absorbing forces. A- External forces The gravitational force acts directly downward through the COG of the body at which the body weight is represented by a line (line of gravity LOG). If the LOG passes directly through a joint axis, no gravitational torque is created around that joint. If the LOG passes at distance away from the joint axis, a gravitational torque is created. This torque will cause motion of the body segments around that joint axis. If the LOG is located anterior to the joint axis, the torque will cause anterior motion of the proximal segment. If the LOG located posterior to the joint axis, the torque will cause a motion in the posterior direction. The gravitational torque is opposed by a counterbalancing torque created in the opposite direction. This counterbalancing torque is done mainly by active muscular tension. Passive tension by ligaments and joints capsules may assist in this counterbalance. Pathway of GRFV The analysis includes the location of the GRFV in relation to joint axis during stance phase form sagittal plane and frontal plane Sagittal plane The relationship of the GRFV to the joint axis used to express the type of moment (flexion, extension) that is acting around joints. The GRFV location in relation to joint axis (anterior, posterior) and the resulting moments represented in the following table Initial contact Loading Mid stance Terminal Preswing response stance Hip A/ flexion A/ flexion P/ P/ P/ extension extension extension Knee A/ extension P/ flexion A/ A/ P/ flexion extension extension Ankle P/ P/ A/ A/ A/ planterflexion planterflexion dorsiflexion dorsiflexion dorsiflexion Frontal plane: The relationship of the GRFV to the joint axis of hip, knee, and subtalar joints in frontal plane may lie lateral or medial or neutral and that determines the type of moment either adduction or abduction (or eversion and inversion). This relation is summarized in the following table. Initial Loading Mid stance Terminal Preswing contact response stance Hip L/ abduction M/adduction M/adduction M/adduction L/ abduction Knee L/ abduction M/adduction M/adduction M/adduction Neutral Subtalar Neutral L/ Eversion L/ Eversion M/ Inversion Neutral b- Internal forces Internal forces which are developed during gait are created primarily by the muscles. The ligaments, tendons, joint capsules and bony components assist the muscle by resisting, transmitting and absorbing forces. To be in a state of equilibrium during gait, the internal and external forces should be balanced and the sum of all forces acting on the body and its segment must be equal to zero. For this reason, the moment created by GRFV must be counteracted by muscle activity and other soft tissue structures. Principle: How to know the acting muscle and type of muscle contraction during gait: 1- Identify the pathway of GRFV and the moment resulting from this passage. If the vector is anterior to the joint so the muscle or other structure in the opposite direction is acting to counterbalance the effect of gravity. 2- Determine the desired joint motion in this sub phase of gait 3- if the joint motion occurs in one direction (e.g. flexion) and the acting muscle works in the opposite direction (extensors), the type of contraction is eccentric Summary of Muscular Activation Patterns through the Gait Cycle Muscles perform several functions during gait. Muscles may act as stabilizers, accelerators, or decelerators. When muscles provide stabilization, they often work isometrically to position one joint or segment while another joint or segment moves. If muscles work as accelerators, they usually contract concentrically to move a segment forward. If they work as decelerators, they are eccentrically contracting to slow a movement or absorb forces produced by a motion. Throughout the stance phase, muscles work to provide weight acceptance, limb stabilization, energy absorption, and propulsion of the limb into swing phase. Sagittal plane analysis Hip joint: -Initial contact: The GRFV passes anterior to hip joint creating flexion moment that is counterbalanced by eccentric contraction of gluteus maximus and hamstring. -Loading response: As limb starts to move from flexion into extension the contraction of gluteus maximus changed into concentric contraction. The activity of gluteus maximus increases while activity of hamstring decreases. -Mid stance, terminal stance and preswing: As the GRFV passes posterior to hip joint, it creates extension moment which is counterbalanced by flexors (mainly iliopsoas, tensor fascia lata, and rectus femoris) activity. The contraction is eccentric at midstance and terminal stance while it changed into concentric at preswing to initiate swing phase. -Initial swing: Hip flexors mainly iliopsoas, sartoruis, and gracilis contract concentrically to initiate swing phase. The sartoruis, and gracilis display small levels of activity at knee joint to induce knee flexion. -Midswing: Muscle action may be absent or minimal in flexors. -Terminal swing: flexors activity stopped and hamstring and gluteus maximus contract eccentrically to control forward progression of lower limb. Kinetic analysis Sagittal plane Knee joint: During stance phase, knee extensors are active to decelerate knee flexion. During swing phase both flexors and extensors contribute to limb progression. The quadriceps is the dominant muscle at knee. Popliteus and the short head of biceps femoris provide direct knee flexion in addition to the help of gastrocnemius muscle. -Initial contact: GRFV passes anterior to knee joint creating extension moment also, concentric contraction of quadriceps occurs as a continuation of their role during terminal swing. Excessive knee hyperextension is prevented by antagonistic action of hamstring. The hamstring muscle is also acting to decelerate the hip joint. The effect of the hamstring muscle force at knee joint is half that at the hip joint because of the shorter lever arm over the knee joint compared to the lever arm over the hip joint. -Loading response: As there is flexion moment at the knee joint created by passage of the GRFV posterior to the knee joint. Quadriceps muscle is active to counterbalance this moment. Eccentric contraction of the quadriceps develops to control the amount of knee flexion. This action serves as shock absorber. N.B: function of eccentric contraction of quadriceps during loading response: 1- control amount of knee flexion. 2- Prevent excessive knee flexion. 3- Decelerate the body. 4- Shock absorption. Dr Noha Elserty -Midstance and Terminal stance: As the GRFV passes anterior to the knee joint in midstance and terminal stance, quadriceps have relaxed, and knee extension stability is provided by 1- Strong ankle planter flexors provide a stable tibia over which the femur continues to advance. 2- Passage of GRFV anterior to the axis of the knee provides a small passive extensor force. At the end of terminal stance, these mechanisms have the potential to create undesirable knee hyperextension. To avoid this, the popliteus and gastrocnemius provide a flexor action posteriorly. -Preswing: The popliteus and gastrocnemius that initially were preventing knee hyperextension are free to initiate knee flexion to unlock knee. As the GRFV passes behind the knee, the flexion moment is counterbalanced by eccentric contraction of rectus femoris to prevent excessive knee flexion. -Initial swing: Toe clearance is essential which is obtained by concentric contraction of the short head of biceps femoris, gracilis, and sartorius muscles. -Midswing: No muscle action is needed and the limb advances by the effect of: Momentum generated by the continuing hip flexion till 65º flexion. -Terminal swing: Concentric contraction of quadriceps is required to lift the weight of tibia and foot. Excessive knee hyperextension is prevented by eccentric contraction of hamstrings to control the forward motion of lower limb. Dr Noha Elserty Ankle joint: -Initial contact: The GRFV passes posterior to ankle joint creating planter flexion moment. The moment is counterbalanced by activity of dorsiflexors. As the desired motion in this sub phase is to be neutral, so there is concentric contraction of the pretibial group. -Loading response: The GRFV lies posterior to the ankle and creates planter flexion moment that moves the foot toward the floor. As this motion is required, eccentric contraction of pretibial muscles is essential for: 1- Decelerate the foot. 2- Control the amount of ankle planter flexion by slowing downward motion of the foot 3- Prevent excessive planter flexion (prevent foot drop). 4- Absorb shock that happens during rapid limb loading. -Midstance The GRFV passes anterior to the ankle joint causing dorsiflexion moment. The moment is counterbalanced by the activity of planter flexors. As there is passive dorsiflexion by the tibia moving over foot, eccentric contraction of soleus and gastrocnemius muscles control motion. Their activity is to slow the rate of tibial advancement. Peroneal muscles and tibialis posterior are also active but with less effect due to their short lever arm. -Terminal stance: As the GRFV shifts to metatarsal heads, maximum dorsiflexion is generated, and it is counterbalanced by strong eccentric contraction of soleus and gastrocnemius. This contraction function as follow: Dr Noha Elserty 1- Control tibial advancement 2- Prevent excessive dorsiflexion 3- Decelerate tibia on the foot -Preswing: Planter flexors reduce their intensity of action. Their concentric contraction is enough to accelerate advancement of the unloaded limb. -Initial swing and midswing: Toe clearance is essential so tibialis anterior, extensor digitorum longus, and extensor hallucis longus contract concentrically to move foot from planter flexed position at preswing to a position of neutral in midswing. Then they act isometrically to maintain the ankle in neutral position throughout the swing phase -Terminal swing: There is isometric activation of pretibial group then at the end of swing phase there is increase in activity of these muscles to assure the ankle will be neutral for optimum heel contact in the upcoming stance phase. The most active one of these muscles is tibialis anterior. Dr Noha Elserty Frontal plane analysis Hip joint During early stance the HAT shift laterally over the supporting limb which creates demands for lateral stability at the hip joint. This lateral stability provided by gluteus medius, glueus maximus and tensor fascia lata. -Initial contact: The GRFV passes lateral to hip joint and creates abduction moment which is counterbalanced by activity of adductor magnus mainly. The motion required from the hip is adduction so the adductor contract concentrically. The pelvis drops slightly. -Loading response: The GRFV lies medial to hip joint creating adduction moment that is counterbalanced by hip abductors contraction to control the lateral pelvic drop on the swing limb (5º). The desired motion is hip adduction and the contraction is eccentric. The main active muscles are gluteus medius, gluteus maximus, and tensor fascia lata. -Midstance and terminal stance: The GRFV lies medial to hip joint creating adduction moment that is counterbalanced by hip abductors contraction. The desired motion is hip abduction and the contraction is concentric of hip abductor. At these sub-phases pelvis elevates. -Preswing: Dr Noha Elserty The GRFV passes lateral to hip joint and creates abduction moment which is counterbalanced by activity of adductor magnus mainly. The motion required from the hip is abduction so the adductor contract eccentrically. Knee joint Knee at stance phase experiences an adduction torque especially during loading response, midstance, and terminal stance. This is counterbalanced by activity of biceps femoris and gluteus maximus tension on iliotibial band that stabilize the knee. Tension of the ligaments at the knee in frontal plane plays an important role during gait. Subtalar joint The GRFV passes at the lateral border of foot during loading response, so subtalar eversion continues during first part of stance phase. This permit foot to adapt to the supporting surface. The eversion moment is counterbalanced by invertor muscles mainly tibialis anterior, tibialis posterior, FHL, and soleus. Dr Noha Elserty Biomechanics I Dr. Noha Elserty Pathological gait Impaired gait in common Orthopedic conditions Gluteus Medius Weakness Gluteus medius weakness may occur as either a frank injury to the gluteus medius or secondarily following an injury to another aspect of the limb. GRFV passes medially to hip joint and creates a strong adduction moment around hip joint so, there is tendency of pelvis and trunk to drop laterally to the opposite side toward non-stance limb and this action should be compensated by abductors muscles activity. so, the opposite hip and pelvis drops during this phase (LR, MST) if the gluteus medius has insufficient strength to hold the pelvis level. For example, if the right gluteus medius is weak, when the individual is in midstance on the right, the left hip and pelvis drops. This is a Trendelenburg gait. Compensation: an individual may compensate for a gluteus medius deficiency by lean the trunk laterally towards the ipsilateral side of weakness during midstance. 1 Biomechanics I Dr. Noha Elserty Moving the HAT directly over the limb reduces the moment arm of the gravitational force as it shifts the GRFV toward the affected side. So, the weak gluteus medius. is not required to exert as much force. If the GRFV passes via hip joint, no moment will be created. If it passes lateral to the joint, it will create abduction moment which will be compensated by activity in adductor moment. Unilateral weakness cause Trendelenburg gait, while bilateral weakness cause waddling gait. This type of gait on the long run cause scoliosis, back pain, and fatigue. 2 Biomechanics I Dr. Noha Elserty Gluteus maximus weakness During initial contact and loading response the GRFV passes anterior to hip joint and creates flexion moment that should be compensated by extensor muscle action (extensor maximus). When gluteus maximus muscle is weak, it fails to compensate the flexion moment so there is tendency for excessive hip flexion (jack knifing) and anterior pelvic tilting. Compensation: The patient will try to prevent trunk from falling forward by leaning the trunk backward to shift the GRFV behind the axis of hip joint. This pattern of gait called gluteus maximus gait. If the weakness is bilateral there is a backward lean of the trunk during the entire stride. On the long run this cause sever lumbar lordosis. Hip joint abnormalities: Three conditions around the hip joint will lead to difficulties in stabilizing the pelvis using the abductors: congenital dislocation of the hip (CDH, also known as developmental dysplasia of the hip), coxa vara and slipped femoral epiphysis. In all three, the effective length of the gluteus medius is reduced because the greater trochanter of the femur moves proximally, towards the pelvic brim. Since the muscle is shortened, it is unable to function efficiently and thus contracts with a reduced tension. In CDH and severe cases of slipped femoral epiphysis, a further problem exists in that the normal hip joint is effectively lost, to be replaced by a false hip 3 Biomechanics I Dr. Noha Elserty joint, or pseudarthrosis. This abnormal joint is more laterally placed, giving a reduced lever arm for the abductor muscles. The combination of reduced lever arm and reduced muscle force gives these subjects a powerful incentive to walk with lateral trunk bending. In many cases, particularly in older people with CDH, the false hip joint becomes arthritic and they add a painful hip to their other problems. Pain is frequently also a factor in slipped femoral epiphysis. Unequal leg length: When walking with an unequal leg length, the pelvis tips downwards on the side of the shortened limb, as the body weight is transferred to it. This is sometimes described as ‘stepping into a hole’. The pelvic tilt is accompanied by a compensatory lateral bend of the trunk. Functional leg length discrepancy Four gait abnormalities (circumduction, hip hiking, steppage and vaulting) are closely related, in that they are designed to overcome the same problem – a functional discrepancy in leg length. An ‘anatomical’ leg length discrepancy occurs when the legs are actually different lengths, as measured with a tape measure or, 4 Biomechanics I Dr. Noha Elserty more accurately, by long-leg x-rays. A ‘functional’ leg length discrepancy means that the legs are not necessarily different lengths (although they may be) but that one or both are unable to adjust to the appropriate length for a particular phase of the gait cycle. For natural walking to occur, the stance phase leg needs to be longer than the swing phase leg. If it is not, the swinging leg collides with the ground and is unable to pass the stance leg. The way that a leg is functionally lengthened (for the stance phase) is to extend at the hip and knee and to plantarflex at the ankle. Conversely, the way in which a leg is functionally shortened (for the swing phase) is to flex at the hip and knee and to dorsiflex at the ankle. Failure to achieve all the necessary flexions and extensions is likely to lead to a functional leg discrepancy and hence to one of these gait abnormalities. This usually occurs as the result of a neurological problem. Spasticity of any of the extensors or weakness of any of the flexors tends to make a leg too long in the swing phase, as does the mechanical locking of a joint in extension. Conversely, spasticity of the flexors, weakness of the extensors or a flexion contracture in a joint makes the limb too short for the stance phase. Circumduction An increase in functional leg length is particularly common following a ‘stroke’, where a foot drop (due to anterior tibial weakness or paralysis) may be accompanied by an increase in tone in the hip and knee extensor muscles. Ground contact by the swinging leg can be avoided if it is swung outward, in a movement known as circumduction. The swing phase of the other leg will usually be normal. The movement of circumduction is best seen from in front or behind. Circumduction may also be used to advance the swinging leg in the presence of weak hip flexors, by improving the ability of the adductor muscles to act as hip flexors while the hip joint is extended. 5 Biomechanics I Dr. Noha Elserty Hip hiking Hip hiking is a gait modification in which the pelvis is lifted on the side of the swinging leg, by contraction of the spinal muscles and the lateral abdominal wall. The movement is best seen from behind or in front. hip hiking is commonly used in slow walking with weak hamstrings, since the knee tends to extend prematurely and thus to make the leg too long towards the end of the swing phase. 6 Biomechanics I Dr. Noha Elserty Steppage Steppage is a very simple swing phase modification, consisting of exaggerated knee and hip flexion, to lift the foot higher than usual for increased ground clearance. It is best observed from the side. It is particularly used to compensate for a plantarflexed ankle, commonly known as foot drop, due to inadequate dorsiflexion control. Vaulting The ground clearance for the swinging leg will be increased if the subject goes up on the toes of the stance phase leg, a movement known as vaulting. This causes an exaggerated vertical movement of the trunk, which is both ungainly in appearance and wasteful of energy. It may be observed from either the side or the front. Vaulting is a stance phase modification, whereas the related gait abnormalities (circumduction, hip hiking and steppage) are swing phase modifications. For this reason, vaulting may be a more appropriate solution for problems involving the swing phase leg. Like hip hiking, it is commonly used in slow walking with hamstring weakness, when the knee tends to extend too early in the swing phase. It 7 Biomechanics I Dr. Noha Elserty may also be used on the ‘normal’ side of an above-knee amputee whose prosthetic knee fails to flex adequately in the swing phase. Abnormal hip rotation Because the hip is able to make large rotations in the transverse plane, for which the knee and ankle cannot compensate, an abnormal rotation at the hip involves the whole leg, with the foot showing an abnormal ‘toe in’ or ‘toe out’ alignment. The gait pattern may involve both stance and swing phases and is best observed from behind or in front. Abnormal hip rotation may result from one of three causes: 1. A problem with the muscles producing hip rotation 2. A fault in the way the foot makes contact with the ground 3. As a compensatory movement to overcome some other problem. Problems with the muscles producing hip rotation usually involve spasticity or weakness of the muscles which rotate the femur about the hip joint. For example, Imbalance between the medial and lateral hamstrings is a common cause of rotation; weakness of biceps femoris or spasticity of the medial hamstrings will cause internal 8 Biomechanics I Dr. Noha Elserty rotation of the leg. Conversely, spasticity of biceps femoris or weakness of the medial hamstrings will result in an external rotation. Several foot disorders will produce an abnormal rotation at the hip. Inversion of the foot, whether due to a fixed inversion (pes varus) or to weakness of the peroneal muscles, will internally rotate the whole limb when weight is taken on it. 9 Biomechanics I Dr. Noha Elserty Pathological gait Quadriceps muscle weakness Normally, during early stance as weight is being shifted onto the stance leg (loading response), the line of force falls behind the knee requiring quad contraction to prevent buckling of knee. With quadriceps weakness, there is inability to counteract the flexion moment which will further leads to instability at heel strike, high tendency for excessive knee flexion and decrease in shock absorption abilities during loading response. Compensation: the main compensation is the action of hip extensors and ankle planter flexors to pull the femur and tibia posteriorly which results in knee extension. Patient leans forward at trunk, causing COG to be shifted forward so line of force falls anterior to knee, forcing it into extension. 1 Biomechanics I Dr. Noha Elserty N.B. if both quadriceps and the gluteus maximus are paralyzed, a patient may compensate by pushing femur posteriorly with his hand at the initial contact and loading response. The arm support trunk and prevent hip flexion and thrusts knee into extension. After long run of compensation, it leads to fatigue and degeneration of ligaments that support knee. Inadequate dorsiflexion control The dorsiflexors are active at two different times during the gait cycle, so inadequate dorsiflexion control may give rise to two different gait abnormalities. During loading response, the dorsiflexors resist the external plantarflexion moment, thus permitting the foot to be lowered to the ground gently. If they are weak, the foot is lowered abruptly in a foot slap. The dorsiflexors are also active during the swing phase, when they are used to raise the foot and achieve ground clearance. Failure to raise the foot sufficiently during initial swing may cause toe drag. Both problems are best observed from the side of the subject. Inadequate dorsiflexion control may result from weakness or paralysis of the anterior tibial muscles or from these muscles being overpowered by spasticity of the triceps surae. 2 Biomechanics I Dr. Noha Elserty Abnormal foot contact The foot may be abnormally loaded so that the weight is primarily borne on only one of its four quadrants. Loading of the heel occurs in the deformity known as talipes calcaneus (also known as pes calcaneus), where the forefoot is pulled up into extreme dorsiflexion, usually as a result of muscle imbalance, such as results from spasticity of the anterior tibial muscles or weakness of the triceps surae. Except in mild cases, weight is never taken by the forefoot and the stance phase duration is reduced. The reduced stance phase duration on the affected side reduces the swing phase duration on the opposite side, which in turn reduces the overall stride length. In the deformity known as talipes equinus (or pes equinus), the forefoot is fixed in plantarflexion, usually through spasticity of the plantarflexors. In a mild equinus deformity, the foot may be placed onto the ground flat; in more severe cases the heel never contacts the ground at all and initial contact is made by the metatarsal heads, in a gait pattern known as primary toestrike. 3 Biomechanics I Dr. Noha Elserty Excessive medial contact occurs in a number of foot deformities. Weakness of the inverters or spasticity of the evertors will cause the medial side of the foot to drop and to take most of the weight. In pes valgus, the medial arch is lowered, permitting weight bearing on the medial border of the foot. Increased medial foot contact may also be due to a valgus deformity of the knee, accompanied by an increased walking base. Excessive lateral foot contact may also result from foot deformity, when the medial border of the foot is elevated or the lateral border depressed, by spasticity or weakness. The foot deformity known as talipes equinovarus combines equinus with varus, producing a curved foot where all the load is borne by the outer border of the forefoot. Although the term club foot may be applied to any foot deformity, it is most commonly applied to talipes equinovarus. 4 Biomechanics I Dr. Noha Elserty Insufficient push off In normal walking, weight is borne on the forefoot during the ‘push off ’ in pre- swing. In the gait pattern known as insufficient push off, the weight is taken primarily on the heel and there is no push off phase, the whole foot being lifted off the ground at once. It is best observed from the side. The main cause of insufficient push off is a problem with the triceps surae or Achilles tendon, which prevents adequate weight bearing on the forefoot. Rupture of the Achilles tendon and weakness of the soleus and gastrocnemius are typical causes. Weakness or paralysis of the intrinsic muscles of the foot may also prevent the foot from taking load through the forefoot. Insufficient push off may also result from any foot deformity, if the anatomy is so distorted that it prevents normal forefoot loading. A calcaneus deformity obviously makes it impossible to put any significant load on the forefoot. Another important cause of insufficient push off is pain under the forefoot, if the amount of pain is affected by the degree of loading (as it usually is). This may occur in metatarsalgia and also when arthritis affects the metatarsophalangeal joints. 5 Biomechanics I Dr. Noha Elserty Abnormal walking base The walking base is usually in the range 50‒130 cm. In pathological gait it may be either increased or decreased beyond this range. While ideally determined by actual measurement, changes in the walking base may be estimated by eye, preferably from behind the subject. An increased walking base may be caused by any deformity, such as an abducted hip or valgus knee, which causes the feet to be placed on the ground wider apart than usual. A consequence of an increased walking base is that increased lateral movement of the trunk is required to maintain balance. The other important cause of an increased walking base is instability and a fear of falling, the feet being placed wide apart to increase the area of support. This gait abnormality is likely to be present when there is a deficiency in the sensation or proprioception of the legs, so that the subject is not quite sure where the feet are, relative to the trunk. It is also used in cerebellar ataxia, to increase the level of security in an uncoordinated gait pattern. Another effective way to improve stability is to walk with one or two canes. A narrow walking base usually results from an adduction deformity at the hip or a varus deformity at the knee. Hip adduction may cause the swing phase leg to cross the midline, in a gait pattern known as scissoring, which is commonly seen in cerebral palsy. In milder cases, the swing phase leg is able to pass the stance phase leg, but then moves across in front of it. Rhythmic disturbances Gait disorders may include abnormalities in the timing of the gait cycle. Two types of rhythmic disturbance can be identified: an asymmetric rhythmic disturbance shows a difference in the gait timing between the two legs; an irregular rhythmic 6 Biomechanics I Dr. Noha Elserty disturbance shows differences between one stride and the next. Rhythmic disturbances are best observed from the side and may also be audible. An antalgic gait pattern is specifically a gait modification which reduces the amount of pain a person is experiencing. The term is usually applied to a rhythmic disturbance, in which as short a time as possible is spent on the painful limb and a correspondingly longer time is spent on the pain-free side. The pattern is asymmetrical between the two legs but is generally regular from one cycle to the next. A marked difference in leg length between the two sides may also produce a regular gait asymmetry of this type, as may a number of other differences between the two sides, such as joint contractures or ankylosis. Irregular gait rhythmic disturbances, where the timing alters from one step to the next, are seen in a number of neurological conditions. In particular, cerebellar ataxia leads to loss of the ‘pattern generator’, responsible for a regular, coordinated sequence of footsteps. Loss of sensation or proprioception may also cause an irregular arrhythmia, due to a general uncertainty about limb position and orientation. Other gait abnormalities A number of other gait abnormalities may be observed, either alone or in combination with some of the gait patterns described above. They include: 1. Abnormal movements, for example intention tremors and athetoid movements 2. Abnormal attitude or movements of the upper limb, including a failure to swing the arms 3. Abnormal attitude or movements of the head and neck 4. Rapid fatigue. 7 Motion analysis system DR. NOHA ELSERTY The main goal of the human movement analysis is the acquisition of quantitative information about the mechanics of the musculoskeletal system while executing a motor task. In general, motion capture (also known as ‘mocap’) is classified into two: (1) marker-based techniques (2) marker-less techniques. Dr. Noha Elserty 1-Marker-Based Motion Capture The two-basic marker-based techniques are: Active markers (light- emitting diodes or optoelectrical) Passive markers. The objectives of marker system are to accurately represent the motion of limb segments and to define the centers of joint rotation for joint force calculations. Dr. Noha Elserty a- Active marker system: This system used active marker to determine the anatomical sites. Each marker is a LED that is pulsed sequentially and activated from a power source. So, the system automatically knows the position of each marker. Dr. Noha Elserty In this system the basic technique consists of placing markers on the skin surface in locations that accurately represent the actions of the underlying joints. These markers are recorded by cameras and their locations translated into motion by complex computer programs. Dr. Noha Elserty Advantages of active marker system: each marker is activated in an automatically known sequence, so the computer know which site is being recorded. Great number of markers can be used close together. Recording rate is much faster Dr. Noha Elserty Disadvantages of active marker system: Dependency on power supply and transmitting cables is restrictive For long duration test, heat generated by LED’s might be problem Dr. Noha Elserty b- Passive marker system Marker-based analysis is generally performed by mounting retro-reflective markers on the subjects’ bodies and reconstructing their 3-D position via video- based optoelectronic systems. If the marker is visible from at least two calibrated cameras at the same time, the 3-D position of a marker can be reconstructed. Marker location is automatically identified by the system through determination of the center of bright area recorded for each marker. Dr. Noha Elserty System setup: The marker location must assure a minimum distance between markers with interval usually is 5-7cm. -Two cameras are needed to record the position of each marker in order to determine the 3 D coordinates of each marker. Rigid fixation of camera is needed. One camera usually gives 2D coordinate and 2 cameras give 3D. Dr. Noha Elserty For gait analysis 3 to 5 cameras are suitable to capture all markers - Camera height should be 2-3 m above the floor. Dr. Noha Elserty Marker position should be picked up each time by at least 2 cameras. - The angle between 2 cameras is preferred to be greater than 45º. - Windows should be closed to eliminate outside light from test area. - Avoid camera light from flashing directly into camera across the room. Dr. Noha Elserty Passive marker placement applied over the skin directly using double-sided adhesive tapes or can be applied through a wand system. In wand system the marker is strapped to the center of the limb segment to reduce the effect of skin motion Dr. Noha Elserty Advantages of passive marker system: No need for electrical cables Camera works with high speed as it produces large number of frames per second Dr. Noha Elserty Disadvantages of passive marker system: Marker dropout: any event that hides the markers from the video will change the recording. Hand swing over the hip, excessive rotation and overlap of the marker locations within the camera fields can cause marker dropout. Dr. Noha Elserty Anatomical Landmarks Anatomical landmarks are either bony prominences or bone points of geometrical relevance, which are normally identified by palpation. they can also be identified by imaging, or functional movements. The estimation of 3-D points of objects from two or more images is called ‘stereophotogrammetry’ Dr. Noha Elserty There are three major sources of errors in human movement analysis performed with stereophotogrammetry: 1- Instrumental errors: these errors stem from the results of both instrumental noise and volume calibration inaccuracies. 2- Soft tissue artefacts 3- Anatomical landmark misplacement Dr. Noha Elserty Skin marker setup: In bilateral analysis during gait, 20 markers are applied over the skin on specific sites which are: Acromion (2 markers; left and right) 12th thoracic vertebra Sacrum Anterior superior iliac spine (2 markers; left and right) Greater trochanter (2 markers; left and right) Superior border of patella (2 markers; left and right) Dr. Noha Elserty Tibial tuberosity (2 markers; left and right) Knee joint line (2 markers; left and right) Lateral malleolus (2 markers; left and right) Heel (2 markers; left and right) Between 2nd and 3rd metatarsal bone (2 markers; left and right) In unilateral analysis: 7 markers are applied over skin 3 markers over foot 3 markers over knee 1 marker over greater trochanter. Dr. Noha Elserty Marker-less system Marker-less motion capture ensures an important reduction of the amount of time for setup preparation in comparison to marker- based techniques. Besides, inter-operator variability is eliminated since no specialized operator is needed to place markers on the skin. Dr. Noha Elserty Dr. Noha Elserty