Biology: Meiosis and Genetics - Chapter 7 PDF
Document Details
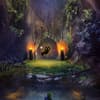
Uploaded by iiScholar
Arizona State University
Tags
Summary
This document is a chapter from a biology textbook covering the process of meiosis, including genetic recombination and independent assortment. The chapter covers the stages of meiosis, genetic concepts, dominance, and hereditary principles.
Full Transcript
osome) are **diploid** (2*n*). **Figure 7.1** Number of chromosome sets in diploid and haploid cells. In humans, [gametes](javascript:void(0)) (ie, sperm, ova \[eggs\]) are haploid, whereas somatic cells (nonreproductive body cells) are diploid. Some organisms are [polyploid](javascript:void(0))...
osome) are **diploid** (2*n*). **Figure 7.1** Number of chromosome sets in diploid and haploid cells. In humans, [gametes](javascript:void(0)) (ie, sperm, ova \[eggs\]) are haploid, whereas somatic cells (nonreproductive body cells) are diploid. Some organisms are [polyploid](javascript:void(0)), which means the cells of these organisms contain more than two complete sets of chromosomes. A diagram of a cell division AI-generated content may be incorrect. Chapter 7: Genetics 271 7.1.02 Phases of Meiosis **Sexual reproduction** involves the joining of haploid **gametes** (ie, egg, sperm) to produce a diploid zygote by **fertilization**. The production of these haploid gametes requires division of diploid parental cells via **meiosis** rather than [mitosis](javascript:void(0)). Meiotic cell division consists of two major stages, meiosis I and meiosis II, and like mitosis, each of these stages is divided into four phases: prophase, metaphase, anaphase, and telophase (Figure 7.2). **Figure 7.2** Stages of meiosis. During prophase I of meiosis, homologous chromosomes in the diploid parental cell pair side by side (ie, undergo **synapsis**), and [crossing over](javascript:void(0)) takes place. The paired homologous chromosomes align at the metaphase plate in metaphase I, and the members of each homologous pair separate to opposite poles of the cell during anaphase I.  Chapter 7: Genetics 272 Unlike anaphase of mitosis, chromosomal sister chromatids do not separate during meiotic anaphase I. Telophase I and cytokinesis then occur, completing meiosis I and resulting in the formation of two haploid **daughter cells**, the chromosomes of which are still composed of two connected sister chromatids. Meiosis II then proceeds via a mechanism very [similar to mitosis](javascript:void(0)), except that mitosis typically occurs in diploid cells, whereas meiosis II occurs only in haploid cells. During meiosis II, sister chromatids separate to form distinct chromosomes that move to opposite poles of the cell during anaphase II. Following completion of telophase II and cytokinesis, haploid gametes are produced. 7.1.03 Independent Assortment Offspring that result from sexual reproduction are [genetically diverse](javascript:void(0)) because the haploid gametes (ie, sex cells) that join to form the offspring are themselves genetically diverse. This diversity arises during formation of the gametes via [meiosis](javascript:void(0)). One feature of meiosis that helps generate genetic diversity among gametes is the [independent assortment](javascript:void(0)) of homologous chromosomes that occurs, as shown in Figure 7.3. **Figure 7.3** Potential chromosome combinations resulting from independent assortment. A diagram of a cell division AI-generated content may be incorrect. Chapter 7: Genetics 273 Each pair of homologous chromosomes in a diploid cell includes a maternal chromosome (ie, chromosome derived from the mother) and a paternal chromosome (ie, chromosome derived from the father). When pairs of homologous chromosomes align at the metaphase plate during metaphase I of meiosis, the orientation of each pair with respect to which of these chromosomes is closer to a given cellular pole is random. Consequently, at the completion of meiosis I, each daughter cell has an equal probability (ie, 50%) of containing either the maternal or paternal chromosome from a given homologous pair. Furthermore, because the movement of each pair of homologous chromosomes during meiosis is independent of the movement of every other pair, each haploid gamete produced via meiosis contains a random combination of maternal and paternal chromosomes. This **independent assortment** of homologous chromosomes into gametes contributes to the gametes\' **genetic diversity** because the maternal and paternal chromosomes in a homologous pair typically exhibit genetic differences. Therefore, each different combination of maternal and paternal chromosomes in a gamete creates a unique combination of genetic information. 7.1.04 Genetic Recombination The process of meiosis allows eukaryotes to produce genetically diverse haploid gametes for sexual reproduction. As depicted in Figure 7.4, meiosis involves a process called **crossing over**, which results in **genetic recombination** between paired homologous chromosomes via the exchange of corresponding DNA segments. Chapter 7: Genetics 274 **Figure 7.4** Contribution of crossing over to genetic recombination via meiosis. Crossing over takes place during prophase I. During this stage of meiosis, homologous chromosomes undergo **synapsis**, a process in which homologous chromosomes line up side by side. Because each chromosome consists of two identical (sister) chromatids at this point in meiosis, the adjacent alignment of a pair of homologous chromosomes is called a **tetrad** (because it consists of four chromatids). Tetrads arise when a **synaptonemal complex** (protein structure) forms between homologous chromosomes and holds them tightly together (Figure 7.5).  Chapter 7: Genetics 275 **Figure 7.5** Role of the synaptonemal complex during crossing over. The tetrad structure allows physical contact between the paternal and maternal chromosomes of a homologous pair, which allows equivalent segments of maternal and paternal chromatids to be exchanged between the chromosomes. This exchange of DNA is the hallmark feature of crossing over and results in the formation of **recombinant chromosomes** that consist of DNA derived from two different parents. Crossing over results in the production of these genetically distinct recombinant chromosomes and is therefore an important source of [genetic diversity](javascript:void(0)) in sexually reproducing species. 7.1.05 Comparing Mitosis and Meiosis Meiosis and mitosis are both forms of **cell division**, the mechanism by which cells reproduce. Meiosis results in the formation of haploid gametes (ie, egg, sperm), and [mitosis](javascript:void(0)) occurs in somatic (non-sex) cells for organism [growth and repair](javascript:void(0)). A comparison of these two processes is outlined in Figure 7.6. A diagram of a cell division AI-generated content may be incorrect. Chapter 7: Genetics 276 **Figure 7.6** Similarities between meiosis and mitosis. Although meiosis and mitosis are similar in many ways, these processes exhibit important differences, which are summarized in Table 7.1.  Chapter 7: Genetics 277 **Table 7.1** Important differences between meiosis and mitosis. **Characteristic** **Meiosis** **Mitosis** Number of rounds of cell division 2 1 Number of daughter cells formed 4 2 Ploidy of daughter cells Haploid Diploid (same as parent cell) Occurrence of synapsis and crossing over between homologous chromosomes Yes No Generates genetic diversity Yes No Primary purpose Gamete production Organism growth, repair Ultimately, meiosis functions to produce genetically diverse haploid gametes required for sexual reproduction, whereas mitosis produces genetically identical daughter cells needed for organism growth, renewal, and repair. **Mendelian Concepts** Introduction **Heredity** (ie, genetic inheritance) involves mechanisms by which genetically based traits are passed from one generation to the next. Scientific understanding of these mechanisms continues to expand, but some of the most fundamental concepts regarding the study of heredity are associated with experiments performed by Gregor Mendel in the mid-1800s. These fundamental concepts underlie numerous techniques used in the study of genetics today. This lesson covers basic concepts and terminology related to genetics, various mechanisms by which genetic traits are inherited, and graphical tools for investigating inheritance. 7.2.01 Gene and Allele Genetic information is transmitted across generations from parents to offspring. The basic unit of genetic information is the **gene** (Figure 7.7), which is a sequence of [DNA](javascript:void(0)) [nucleotides](javascript:void(0)) that encodes a functional product, such as a [protein](javascript:void(0)) or [RNA](javascript:void(0)) molecule. Genes are carried on [chromosomes](javascript:void(0)), and the specific site of a gene on a chromosome is referred to as a **locus** (plural: loci). A single chromosome may contain thousands of genetic loci. **Figure 7.7** Location of genes on chromosomes. A diagram of a cell AI-generated content may be incorrect. Chapter 7: Genetics 279 Genes can be present in alternative forms, which are called **alleles** (Figure 7.8). Inheritance of different alleles for a particular gene by different individuals can result in those individuals expressing different forms of the trait controlled by the gene (eg, flower color). The allele that produces the form of a trait most commonly observed in a natural population is called the **wild-type** allele. As opposed to wild-type alleles, **mutant** alleles often produce altered or abnormal forms of a trait. **Figure 7.8** Alleles of a gene. 7.2.02 Genotype and Phenotype Organisms inherit genetic information from their parents. In a general sense, all genetic information that an organism possesses makes up the organism\'s **genotype**. More specifically, diploid organisms (eg, humans) typically possess two alleles for each gene, and the term genotype refers to the two alleles present at a particular genetic locus. As shown in Figure 7.9, if both alleles at a locus [are the same](javascript:void(0)), the genotype of this locus is **homozygous**. Alternatively, if two different alleles are present at a locus, the genotype of the locus is **heterozygous**. **Figure 7.9** Components of a genotype. An organism\'s genetic makeup (ie, genotype) is expressed to produce the organism\'s observable physical characteristics, or **phenotype** (Figure 7.10). An organism\'s phenotype includes factors such as pattern of development, molecular composition, appearance, and behavior, and phenotype can be affected by the environment.  A diagram of a dna molecule AI-generated content may be incorrect. Chapter 7: Genetics 280 **Figure 7.10** Genotype versus phenotype. 7.2.03 Dominance Alleles, which make up an organism\'s genotype, [can be found](javascript:void(0)) on autosomes, sex chromosomes, and mitochondrial DNA. These alleles may be **dominant** or **recessive**, with dominant alleles commonly being represented by capital letters and recessive alleles by lowercase letters. As shown in Figure 7.11, dominant alleles at a particular locus always affect the phenotype, whereas the phenotypic effects of recessive alleles are apparent only when no dominant alleles are present at the locus. **Figure 7.11** Dominant and recessive alleles.  A diagram of a flower AI-generated content may be incorrect. Chapter 7: Genetics 281 Consequently, **dominant phenotypes** are expressed by organisms that [have either](javascript:void(0)) homozygous dominant or heterozygous genotypes. Conversely, **recessive phenotypes** are [expressed only](javascript:void(0)) by organisms that have homozygous recessive genotypes. 7.2.04 Codominance and Incomplete Dominance Diploid organisms possess two complete sets of chromosomes (ie, have [homologous chromosomes](javascript:void(0))). Consequently, the genotype of a diploid organism is composed of two [alleles](javascript:void(0)) per gene (with the exception of some genes on sex chromosomes). More than one allele exhibiting complete dominance may exist for certain genes in the [gene pool](javascript:void(0)), which means that it is possible for a diploid organism to inherit two different dominant alleles for a particular gene (see Figure 7.12). Alleles exhibiting complete dominance are fully expressed in an organism\'s phenotype. Therefore, when an organism inherits two different dominant alleles for a particular gene, both alleles can be fully and independently expressed. This situation, in which the phenotype shows the full contribution of two different dominant alleles in a heterozygous genotype, is called **codominance**. Alternatively, some alleles exhibit a pattern of expression called **incomplete dominance**, in which neither allele in heterozygotes is fully expressed. When incompletely dominant alleles are present, heterozygous individuals exhibit a blended phenotype intermediate to the phenotypes produced by homozygous dominant and homozygous recessive individuals. **Figure 7.12** Comparison of codominance and incomplete dominance.  Chapter 7: Genetics 282 7.2.05 Penetrance and Expressivity The phenotype an organism exhibits is determined by the organism\'s genotype, environmental conditions, and other factors such as diet. Due to the multiple elements that influence an organism\'s phenotype, not all organisms that possess the same genetic variant (ie, have the same genotype) exhibit identical phenotypes, as illustrated in Figure 7.13. The **penetrance** of a genotype is the proportion of individuals with the genotype that express the expected phenotype. If 100% of individuals with a given genotype express the expected phenotype (at least to some degree), the genotype is said to be fully penetrant (ie, have complete penetrance). If less than 100% of individuals with the genotype express the phenotype, the genotype is said to have incomplete or reduced penetrance. **Variable expressivity** refers to the ability of a single genotype to produce a range of degrees of expression of the expected phenotype among individuals with the genotype. For example, a disease-causing genotype with variable expressivity results in individuals exhibiting a range of disease severities despite all having the same genotype. **Figure 7.13** Penetrance and expressivity. 7.2.06 Genetic Cross Experimental **genetic crosses** allow researchers to observe patterns of inheritance across generations. A simple (monohybrid) [genetic cross](javascript:void(0)) starts by crossing (ie, mating) organisms that have known genotypes and that differ phenotypically in a single trait. The first cross typically involves homozygous parent organisms (ie, true-breeding) for particular alleles. These organisms represent the **parental (P) generation**. In a simple genetic cross, offspring from the P generation cross are called the **first filial (F1) generation** and are all heterozygous individuals that express the dominant phenotype (ie, all F1 members resemble one parent only). Mating among members of the F1 generation results in offspring that represent the A chart with green squares AI-generated content may be incorrect. Chapter 7: Genetics 283 **second filial (F2) generation**, with approximately 75% of this generation expressing the dominant trait and approximately 25% expressing the recessive trait. An example of a simple genetic cross is shown in Figure 7.14. **Figure 7.14** Simple genetic cross. 7.2.07 Punnett Square A **Punnett square** is a graphical tool that can be used to predict the distribution of alleles provided by two individuals with known genotypes involved in a genetic cross (see Figure 7.15). A Punnett square is set up by writing each possible combination of alleles in maternal [gametes](javascript:void(0)) along the top of a grid and each possible combination of alleles found in paternal gametes down the left side (or vice versa). The squares of the grid are then filled in with the corresponding maternal and paternal contributions, and each square represents an equally probable outcome (ie, offspring genotype). The probabilities of specific offspring genotypes resulting from the cross can be determined using the Punnett square.  Chapter 7: Genetics 284 **Figure 7.15** A Punnett square. 7.2.08 Testcross Recessive alleles are [phenotypically masked](javascript:void(0)) by dominant alleles in heterozygotes. Consequently, organisms with homozygous dominant genotypes and organisms with heterozygous genotypes typically exhibit the same phenotype. To determine whether an organism exhibiting a dominant phenotype is homozygous for the dominant allele or heterozygous, a **testcross** can be performed (Figure 7.16). In a testcross, an organism exhibiting the dominant phenotype (and unknown genotype) is mated to an organism exhibiting the recessive phenotype (ie, homozygous recessive genotype). If all offspring resulting from the testcross exhibit the dominant phenotype, it can be concluded that the parent exhibiting the dominant phenotype is *homozygous* for the dominant allele. Alternatively, if approximately half the offspring exhibit the recessive phenotype, it can be concluded that the parent exhibiting the dominant phenotype is *heterozygous*. A diagram of alleled AI-generated content may be incorrect. Chapter 7: Genetics 285 **Figure 7.16** A testcross. 7.2.09 Pedigree Analysis A **pedigree** is a diagram that shows the occurrence of a trait across multiple generations of a family (see Figure 7.17). In the diagram, females (XX) are represented by circles and males (XY) by squares. Direct horizontal lines between individuals represent matings, and offspring resulting from a mating are identified via a vertical line extending down from the horizontal line. Individuals who express the trait being studied are identified by shading. **Figure 7.17** Sample pedigree.  A diagram of a family tree AI-generated content may be incorrect. Chapter 7: Genetics 286