Biology Notes (1) PDF
Document Details
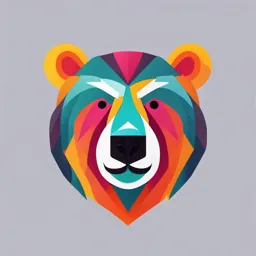
Uploaded by AuthenticEcstasy516
ECCG Sierre
Tags
Summary
These notes cover the topics of water, nucleic acids, and the origins of cells in biology. They focus on the properties of water and its role in biological processes, and delve into the structure and function of nucleic acids. They also discuss conditions on early Earth and the implications for the development of life.
Full Transcript
A1.1 - Water Polar covalent bonds - O₂ atom covalently bonded to 2 H atoms Part. (+) H side is attracted to part. (-) O₂ (weak - partial charges) Intermolecular force - H bond holds mol. together - H-bonds minimizes SA Water striders habitat - detergents...
A1.1 - Water Polar covalent bonds - O₂ atom covalently bonded to 2 H atoms Part. (+) H side is attracted to part. (-) O₂ (weak - partial charges) Intermolecular force - H bond holds mol. together - H-bonds minimizes SA Water striders habitat - detergents interfere Water required for: 1. Effective interaction of naturally formed mol. of life → growth & copying 2. Formation of compartments - cytoplasm (metabolic reactions) Cohesion Adhesion :attraction between water mol. (H bonds btwn. :attraction between water mol. & other mol) substances E.g: Vascular plants - use cohesion to Capillary action: water is pulled through transport water up the xylem. Water narrow tubes & space evaporates from the surface of inner cell walls E.g: Sand & soil - draw moisture above water line (water absorbed - replaced) into air space in the leaf - replaced by water Move water into cell walls - cellulose fibers in within the cell. Water mol move xylem - leaf, xylem walls draw in water & capillary action tension is transmitted to the root replaces it as evaporation occurs, preventing cells drying out. Hydrophobic mol. Hydrophilic mol. :repel water mol. :mol. attracted to water mol. Non-polar Polar Dissolve non-polar solvents Dissolve polar solvents E.g: Hydrophobic portion - cell membrane forms barrier & proteins - membrane give cells properties (Aquaporins anchored by hydrophobic exterior - provide hydrophilic interiors - enable efficient water movement) Cytoplasm - substrates of metabolic reactions & enzymes that catalyse them are dissolved - Mol. interact - allows cells control their composition, respond to environment & maintain function of life E.g: Enzymes require level of water in structures to maintain natural conformation - deliver functionality (amylase binds with starch in aq solution to break it down - sugars) Water dissolves sugars, salts, amino acids - enabling material transport in multicellular organisms. Animals use (water-based) blood/haemolymph to transport nutrients, hormones, & wastes. Blood plasma uses water - solvent to transport small, hydrophilic materials (glucose). Non-polar substances (oxygen gas) do not dissolve well in water. - Animals evolved specialized proteins - haemoglobin in RBCs to bind & transport O₂ Dissolved minerals in water are carried through soil to plant roots. Root hairs absorb minerals & water for transport through the xylem. Plants transport dissolved sugars & organic molecules in sap via phloem. - Phloem sap contains a mix of organic & inorganic substances - sugars & amino acids (sucrose transported - solute in water) Physical properties of water: 1. Buoyancy :force counteracts gravity - High density water - high buoyancy - less dense materials float - air less dense - low buoyancy - Ice is less dense than water due to hydrogen bonding - float. - Cold liquid water sinks, preserving liquid layers under frozen surfaces for life. 2. Viscosity :resistance to flow - Water has low but higher viscosity than air. 3. Thermal :ability of a substance to transfer heat - temperature difference conductivity - Water - high thermal conductivity - Air - low thermal conductivity 4. Specific heat :how much energy is needed to raise the temperature of a capacity substance - H-bonds must be overcome to temp - additional energy - water's high specific heat higher than air & land. Ringed seals Black-throated loon Buoyancy Low-density blubber to their Large wins to generate lift in buoyancy less buoyant air Viscosity Streamlined shape to reduce Loons - shape is hydrodynamic drag against viscosity to reduce drag against viscosity Loons are aerodynamic to reduce drag when flying Thermal Insulating blubber to reduce Tight oiled feathers to exclude conductivity heat loss (low thermal water conductivity) Loons are insulated by air in down feathers Specific heat Seals pups survive in lairs under the snow insulated by trapped air Origin of water (HL) Earth, formed 4-5 billion years ago - too hot for water Water delivered through collisions with water-rich asteroids (comets). - Asteroid collision rates varied - meteorite collisions - dozens daily Reasons for water retention on Earth Gravity, stronger on Earth - exert a greater gravitational force - retain water as the planet cools. Liquid water allowed for life to evolve Search for extraterrestrial life Astrobiologists - extraterrestrial life requires liquid water. Earth is in the goldilocks zone/ habitable zone - refers to the orbital distance from a star where liquid water can be retained. Mars may have once had liquid water, as shown by ancient lake & river impressions A1.2 - Nucleic acids Nucleic acid chains of nucleotides (monomers) Nucleotides join together to form - nucleic polymer by polymerisation (condensation reaction). 1. Pentose sugar 2. Nitrogenous base 3. Phosphate group Sugar-phosphate backbone of DNA & RNA :Nucleotide units covalently bonds to form DNA - Bond forms between the phosphate group attached to the 5’C of one sugar & the hydroxyl (–OH) attached to the 3’C of another sugar, releasing 1 mol. - water & requiring energy. - 5′ end (phosphate) & the 3′ end (–OH group) In DNA/RNA the sequence of the nitrogenous bases forms the genetic code. Covalent bonds between adjacent nucleotides Nitrogenous bases: Purines (2 rings) Pyrimidines (1 ring) Adenine Thymine (DNA) Guanine Cytosine Uracil (RNA) DNA is a double-stranded, helical & stabilized by complementary base pairing: adenine (A) - thymine (T) & guanine (G) - cytosine (C) through H-bonds. - DNA strands are antiparallel: 5′ to 3′ & 3′ to 5′ DNA RNA Pentose sugar Deoxyribose Ribose Pentose sugar structure Nitrogenous base A,G,C,T A,G,C,U Polymer structure Double-stranded mols. Single-stranded molecule connected by H-bonding Complementary base pairing ensures accuracy during DNA replication by maintaining the base sequence during cell divisions Replication - one DNA strand serves as a template to create a new strand - sequence stays the same - cell division. DNA codes for proteins - gene expression (gene can be expressed/not) - Complementary ensures the genetic code produces the same protein every time a gene is expressed - maintaining the characteristics of cells & the organism. DNA - limited capacity to store info b.c - finite num. of base sequences Conservation of the genetic code Genetic code: base sequences in a gene that translate into functional proteins - All living organisms - share same genetic code (common ancestry) - viruses cannot translate DNA independently Directionality of RNA & DNA Replication, transcription & translation occur in 5’ to 3’ direction - Enzymes are specific E.g: DNA polymerase (replication) attaches only to the 3’- OH group. Less energy is required for processes in the 5′ to 3′ direction & the direction is crucial due to the orientation of the enzymes involved. Purine & pyrimidine thymine (T) - adenine (A) - formation of 2 H-bonds cytosine (C) - guanine (G) - formation of 3 H-bonds Stabilises DNA - length of the base pairs is consistent throughout the helix Bonds - A & G - same shape as G & C to accommodate 2nm diameter (helix’s pitch) Purines cannot pair b.c double-ring structures Higher bond specificity stability & bond’s strength Mismatching in DNA replication - mismatch point detected (incorrect length of base pair) & fixed - Mismatch persits - termination of cell division, cell death/cancers Nucleosomes Eukaryotic DNA (nucleus) is bound to histone proteins Prokaryotic DNA (cytoplasm) lacks histones - “naked DNA” A nucleosome consists of ~150 base pairs of DNA wrapped around a core of eight histones & a H1 histone. - DNA linker links nucleosomes together Large eukaryotic genomes require DNA packaging through folding, coiling, & supercoiling to fit into the nucleus. - Nucleosomes enable DNA supercoiling while allowing access by uncoiling & moving histones for replication/transcription. Nucleosomes are the repeating units of chromatin fiber, which further coils to form chromosomes Hershey and Chase data (HL) - Demonstrated that DNA, not protein, is the genetic material. T2 bacteriophages inject DNA into bacterial cells while their protein coat remains outside. DNA was labeled with radioactive phosphorus (32P) - phosphorus is found in DNA but not protein. Protein labeled with radioactive sulfur (35S) - sulfur is found in protein but not DNA. Findings: When 32P-labeled viruses infected bacteria, the bacterial cells & subsequent virus generations were radioactive, proving DNA is the genetic material. When 35S-labeled viruses infected bacteria no significant radioactivity was detected inside the infected cells, showing the protein remained outside. Chargaff’s data Before Chargaff's work, DNA was thought to be single-stranded with repeating tetranucleotide units. Chargaff used paper chromatography to measure concentrations of nitrogenous bases in DNA - % of Adenine = thymine & cytosine = guanine across organisms - supports the double helix model & complementary base pairing. - Evidence used by Crick & Watson to build - double helix model. A2.1 - Origins of cells (HL) Conditions of Earth (3.5 - 3.9 billion years ago) - High surface temp, single ocean & no land masses Early Earth was bombarded by comets & asteroids, introducing water, methane, ammonia & other compounds. Volcanic eruptions & collisions released methane, ammonia, water vapor, & high levels of CO₂ - forming Earth's early atmosphere. - Atmosphere lacked oxygen - no ozone layer, & Earth’s inner core - hotter & liquid - increased motion & produced a weaker magnetic field, increasing exposure to cosmic & solar radiation. - Conditions - extreme weather events; electrical storms. The prebiotic formation of carbon compounds Reducing gases (atmosphere) donates electrons to other mol. - enabled chemical reactions that formed amino acids & hydrocarbons (simple carbon compounds) - Joined to form proteins, lipids, & nucleic acids (complex carbon compounds) some - self-replicate & packaged into membranes - enabled molecules to exist in diff. conditions to the external environment leading to the formation of cells. Earth’s conditions today prevent the spontaneous formation of biological molecules. Cell components can carry out the 8 processes of life: :chemical reactions that Cells contain enzymes to speed up Metabolism take place within the cell(s) chemical reactions in the cell of an organism :responding to changes in Detecting changes in chemicals in the Response to the external environment extracellular environment & moving stimuli towards/away from the chemicals :maintenance of constant Moving ions/other mol. in/out of the cell Homeostasis internal conditions, despite across the cell membrane to control the changes - external environ. concentration of substances in the cell :having some control over Specialised structures (cilia, flagella & Movement their place & position pseudopodia) help them move/change position :increasing in size over Cells can divide to produce more cells, Growth time. & in size Multicellular organisms - in the num. of cells in organism :production of offspring Cells contain genetic material - Reproduction instructions for the cell to function & reproduce. During reproduction of a cell, genetic material is copied to be passed onto the offspring :removal of metabolic waste Metabolic waste products are Excretion transported across the cell membrane, into the external environment :intake/production of Cells can produce their own nutrients Nutrition nutrients. Heterotrophic (photosynthesis) or obtain nutrients by organisms obtain nutrients - consuming other organisms/organic external environment molecules & diffuse mol. across the Autotrophic organisms - membrane (endocytosis). inorganic material Viruses - non-living b.c - no metabolism, unable to reproduce outside of the host cell & rely on the host cell for nutrition & growth. Steps for the evolution of the first cells: 1. Simple organic molecules: amino acids & hydrocarbon chains were formed. 2. Catalysis accelerated chemical reactions 3. Larger organic molecules (RNA & phospholipids) were assembled from smaller molecules. 4. Smaller molecules (RNA) self-replicated 5. The formation of a membrane-bound compartment (cell surface membrane) separated the cell's internal chemistry from its external environment. Evidence for the origins of carbon compounds Experiment simulated Earth's prebiotic conditions to show organic molecules could form spontaneously. Set up a closed system - Water (simulate oceans) - Gas inlet for methane, ammonia, & hydrogen (reducing gases) - Electrical sparks (simulate electrical storms) The water was vaporized, passed through the gases, & electrical sparks, then condensed to form water droplets, representing Earth's primordial soup(early oceans) - The experiment produced basic organic monomers - amino acids. The experiment demonstrated that under early Earth conditions the non-living synthesis of organic molecules was possible (suggests carbon compounds could have originated this way) Spontaneous formation of vesicles by coalescence (merge) of fatty acids into spherical bilayers First cell formed when fatty acids coalesced to form a spherical bilayer, enclosing a space (membrane-bound compartment) The vehicle's interior provided a internal chemical environment - Membrane separation allows cells to control conditions (pH & solute concentration) to function The first genetic material; RNA - 'RNA first' hypothesis suggests RNA - genetic material & catalyst in the first cell-like structures. Hypothesis states: 1. RNA was formed from inorganic sources, replicated using ribozymes, & catalyzed protein synthesis. 2. Membrane compartmentalization occurred, & RNA produced proteins & DNA. 3. DNA became the main genetic material due to its stability, & proteins took over catalytic functions (enzymes) b.c of their variability Evidence supporting the hypothesis: Short RNA's ability to self-replicate RNA has some catalytic activity - genetic material & enzyme of early cells Ribozymes in the ribosome - used to catalyze peptide bond formation (protein synthesis) Other hypotheses include: Miller–Urey hypothesis: Spontaneous generation of organic molecules - conditions of prebiotic Earth. Metabolism first hypothesis: Life began with simple metabolic reactions - formation of simple metabolic pathways forming complex molecules - formed the basis of cells Sulfur world hypothesis: Life based on iron-sulfur chemistry. Lipid world hypothesis: Lipid bilayers evolved before RNA - protective layer encapsulating RNA The last universal common ancestor (LUCA) (HL) :single-celled, anaerobic organism with a DNA genome. LUCA/its descendants outcompeted other early life forms, leading to their extinction. Evidence for LUCA: The genetic code is universal - shared by all organisms & viruses using the same codons for amino acids (E.g: AUG codes for methionine) - Common ancestor from 1 LUCA whose genetic code has been conserved for genetic info. transmission (genetic code changes detrimental to the LUCA) (Genetic code - study diffs. in the genome of organisms groups evolved) Molecular phylogenetics (genetic seq. comparison) demonstrated that LUCA likely gave rise to 2 domains not 3: bacteria & archaea b.c eukaryotes evolved later by endosymbiosis (two-domain phylogenetic tree) Genes common with bacteria & archaea - present in LUCA: 11,000 common genes between bacteria & archaea, indicate LUCA had advanced functions. The concept of horizontal gene transfer (genes can be transferred across species/domains) led scientists to focus on ‘ancient’ conserved genes (bacteria & archaea) - not undergone horizontal gene transfer. - 355 conserved genes shared by both domains - present in LUCA. Stromatolites (fossilized microbial communities) & genetic analysis infer LUCA's characteristics: Existed 2.5 to 3.5 billion years ago. Existed in deep ocean hydrothermal vents, using sulfur, methane, & iron for energy & offering protection. High temp. provided energy for complex org. mol. formation for cellular formation. (Phylogenetic analysis & fossilised evidence) Was anaerobic - lack of O₂ in early Earth's atmosphere Was autotrophic, combining inorganic carbon & H into usable compounds - formic acid & CO₂ A2.2 - Cell structure Cell theory: Cells are the smallest unit of life All cells are formed from preexisting cells All organisms are made up cells Stains bind to cell structures (Light microscope): - Iodine binds with starch - plant cells - Methylene blue binds to the nuclei of cells - animal cells - Gram staining - bacteria Stage micrometres calibrate eyepiece graticules: 1 graticule division = 5µm Converting units: Light v.s Electron microscopy: Light microscopy Electron microscopy Lenses to magnify A beam of electrons to create an image of a sample Low resolving power High resolving power Lower magnification up to x2000 Higher magnification up to x500,000 Dead/alive specimens Dead specimen Studies tissues & living cells Commonly used to view cells and cellular structures Stains help visualise the the structure of the Black & white observations specimen Techniques used in microscopy: Freeze fracture Frozen sample is fractured microscopy Electron microscopy is used to view internal structures (not (membrane structure) normally visible - plasma membranes) Cryogenic electron Sample is frozen to cryogenic temps. - fix mols for stability. microscopy Electron microscopy is used, improving resolution & (3D structure of mols.) reducing damage from the electron beam. Immunofluorescence Fluorescent tags (fluorophores) are attached to antibodies (pathology) specific to target antigens. Light of specific wavelengths causes the tags to emit light - visualising of target molecules. Fluorescent dyes Dyes bind preferentially to certain structures. (biological structures) Labelled areas fluoresce under light, showing the distribution of target molecules. Prokaryotic and Eukaryotic cells Typical cell: DNA, cytoplasm (contains dissolved solutes) & plasma membrane (bilayer of phospholipids) Advantages of compartmentalisation: Allows higher concentrations of substances within organelles. Separates toxins & damaging substances from the rest of the cell (e.g: hydrolytic enzymes in lysosomes away from the cytoplasm) Maintains optimal conditions (pH) for specific enzymatic functions. Eukaryotic cell components: Eukaryotic cell Description 10 - 100 μm Membrane-bound organelles Can be unicellular/multicellular Asexual (mitosis) or sexual (meiosis) reproduction Nucleus Nucleus contains DNA associated with histone proteins, organised into chromosomes. (double-membrane with pores - certain mol.) Cytoplasm Contains organelles & site of metabolic reactions. Cell wall Plants (cellulose), fungi (chitin); absent in animal cells. Plasma Separates the cell’s interior from its external environment & controls membrane entry/exit of substances. Ribosomes 80S- translation (cytoplasm or membrane bound) Rough & smooth R - Has ribosomes attached to its surface which produce proteins that endoplasmic are usually destined for use outside the cell. reticulum S - produces & stores lipids (steroids) Golgi apparatus Processes & packages proteins - to be released in Golgi vesicles. Vesicle Small sac - transport and release substances produced in the cell by fusing with the plasma membrane. Vacuole Maintains osmotic balance, stores substances, & may have hydrolytic functions. Cytoskeleton Protein fibres (microtubules/microfilaments); maintain cell structure, shape, & organelle positioning. Mitochondria Double-membrane-bound organelles that convert glucose into ATP by respiration. Prokaryotic cell components: Prokaryotic cell Description 0.1 - 5.0 μm Earliest cells (~3.5 billion years ago); unicellular; no membrane-bound organelles. Found in extreme environs. Asexual (binary fission) reproduction Nucleus No nucleus; Naked DNA not associated with histone proteins (nucleoid) Cytoplasm Jelly-like fluid suspending ions, molecules, DNA, & ribosomes; site of metabolic reactions. Cell wall Protects against toxins, maintains shape, resists osmotic pressure. Plasma Separates the cell’s interior from its external environment & controls membrane entry/exit of substances. Ribosomes 70S Ribosomes - site of protein synthesis - translation (free in the cytoplasm) (smaller than 80S) Plasmid Small, circular DNA enabling horizontal gene transfer. Other Capsule: polysaccharide outer layer for protection & adhesion. Flagellum: for locomotion Pili: protein filaments (cell wall) aiding adhesion & DNA transfer between cells. Prokaryotic Eukaryotic Unicellular organisms: Paramecium Chlamydomonas Genus of unicellular protozoa (0.25 mm) Genus of unicellular green algae - Found in aquatic environments - (Chlorophyta) (10–30 μm in diameter) stagnant ponds & heterotrophs. - Soil, freshwater, oceans, & snow - Use cilia to move & beat rhythmically - mountain tops. propel cell in directions. How Paramecium & Chlamydomonas carry out the 8 functions of life: Paramecium Chlamydomonas Growth Enlarges with food intake; certain Grows by - org. mol. production size divides - 2 daughter cells (photosynthesis) & absorption of (binary fission) minerals. Certain size - divides into 2 daughter cells (binary fission/sexually) Movement The wave action of the beating cilia Flagella rotate to move towards propels in response to changes in favorable conditions (higher light the environment (to warmer waters) intensity) Response to Detects temperature changes & Eye spot senses light changes & moves stimuli moves towards warmer temp. toward light - photosynthesis Homeostasis Osmoregulation :contractile vacuoles collect excess water & expel it across the plasma membrane - maintain its internal environment. & water balance Nutrition Heterotroph: engulfs food particles Autotroph; photosynthesizes using large for digestion in vacuoles - soluble chloroplasts products are absorbed into - cytoplasm. - Feeds on microorganisms (bacteria, algae & yeasts) Reproduction Binary fission - cell divides into two Certain size - cell divides into two daughter cells (asexual) & less daughter cells. Binary fission (asexual) common sexual reproduction. /sexual reproduction. Excretion Metabolic waste from cytoplasm Excretes waste through plasma collects in vacuoles. Vacuoles move membrane. to anal pore & rupture to expel waste. Contractile vacuoles also remove waste with excess water. Metabolism Breaks down external organic Heterotroph; uses a chemical carbon sources & carbon compounds for source (acetate) energy. Mixotroph; uses both CO₂ & acetate for energy In addition to components of a eukaryotic cell: Animal cell: Centrioles: 2 cylindrical organelles - organize microtubules in cell division. Lysosomes: membrane-bound bags of hydrolytic enzymes; break down molecules & old organelles (found in phagocytic white blood cells - fuse & destroy ingested pathogens) Vacuoles: store water, nutrients & waste. Cilia: hair-like structures made of microtubules - move substances (e.g: epithelial cells of bronchi cilia beat in unison to clear debris out of the respiratory tract). Plant cell: Cell wall: made of cellulose; provides protection, shape & resists osmotic pressure Chloroplasts: double membrane bound - convert light into chemical energy by photosynthesis; contain chlorophyll. Vacuoles: large, regulates osmotic potential & stores substances Fungal cell: - Reproduced by budding - scars (crater ring of tissue forms when daughter cell buds from parent cell - scar num. indicates num. cell has divided Cell wall: made of chitin, provides structure Vacuoles: organize & produce microtubules (cytoskeleton) for cell division. Centrioles: organize & produce microtubules (cytoskeleton) - aid in cell division Atypical Cell Structures in Eukaryotes (contain abnormal/ don't contain normal num. - cell structures & organelles): Multinucleated Cells Anucleate Cells Skeletal Muscle Cells: Mature RBC: Anucleated, allowing Contain many nuclei - formed from the more haemoglobin for oxygen fusion of smaller myocytes. transport. Aseptate Hyphae (Fungi): Sieve Tube Elements (Phloem): Lack septates, resulting in multiple Anucleate - contain minimal nuclei in a single cellular unit cytoplasm & organelles - low resistance for substances moving through a sieve tube element Endosymbiosis, cell differentiation and the evolution of multicellular organisms (HL) Eukaryotes evolved from a common unicellular ancestor (~2.7 billion years ago) with a nucleus & sexual reproduction. Endosymbiotic Theory :eukaryotes evolved from a common ancestor endocytosed a prokaryotic cell- cells remained insides the host - providing energy to the host cell & evolving: Prokaryotes carrying out aerobic respiration → evolved into mitochondria. Cyanobacterium (Prokaryote capable of photosynthesis) → evolved into chloroplasts Evidence: Mitochondria & chloroplasts - similarities with prokaryotes: ~8 μm in length Double membranes (inner from prokaryote, outer from the vesicle the cell was taken into the ancestor). Circular naked DNA & 70S ribosomes Divide by binary fission. Susceptible to antibiotics targeting prokaryotes. Multicellular eukaryotes have specialised cells with distinct functions & structures - form tissues & organs. Cell Differentiation Selective gene expression: - Turning on genes needed for specific cell functions - Turning off unnecessary genes for the function Triggered by cellular environment changes E.g: Human development - early embryonic cells are undifferentiated - as embryo develops cellular environment changes triggered gene expression → cells differentiate into specialised cells (muscle & nerve cells) Cell differentiation allows - multicellular organisms: Increased body size. Greater complexity & adaptability to environment changes Evolution of Multicellular Organisms Multicellularity evolved repeatedly through cell aggregation: Individual cells cluster together for efficient nutrient obtaining, sharing & predator protection. Some cells within clusters differentiated to have specialised roles. A2.3 - Viruses (HL) :small infectious particles (20nm - 500 nm) (electron microscope) Contain: - RNA/DNA - Capsid made of protein - protects the genetic material. - Few/no enzymes - No cytoplasm/plasma membrane RNA viruses have a simple structure & directly use RNA for replication & protein production. Viral structure diversity Capsid shapes: - Simple & spherical (Influenza virus) or complex & multilayered (Bacteriophage). Viruses with diff. genome types: Viral genome Viruses Single-stranded RNA HIV, influenza, coronaviruses Double-stranded RNA Rotaviruses Single-stranded DNA Parvovirus Double-stranded DNA Bacteriophage lambda, variola virus, herpes viruses Genome size (total genetic info. of an organism): - 4 genes (small viruses) to ~2500 genes (giant viruses). Enveloped Viruses Non-Enveloped Viruses :membrane that surrounds the capsid :lack an envelope - ob made of lipids & proteins from the host cell - More resistant & stable to membrane. environmental factors (b.c envelopes - Protects the capsid & viral genome. are damaged by heat, light, - Disguises viruses from the host chemicals). immune system. E.g: norovirus, adenovirus & bacteriophage - Helps the viruses attachment & entry lambda into host cells. E.g: HIV & Influenza Viruses are obligate intracellular parasites (dependant on host cell) - Don’t contain organelles & cell structures to replicate & reproduce outside - host cell. Dependant on the host cell for : Energy Viruses lack their own source of energy → rely on the host cell for energy for replication. Nutrients Unable to obtain nutrients. Replication Do not have the machinery for replicating genetic machinery material. Use the host cell’s ribosomes & enzymes for transcription & translation of viral proteins. Transport Viruses use the host cell’s transport machinery to move within the body or spread to other host cells. To access the host cell & take over its machinery viruses must enter: Endocytosis Receptor-mediated Fusion 1. Viruses are engulfed by the host cell 1. Viruses bind to into an receptors on endosome the host cell (invagination of membrane. the host cell 2. Fuse with the membrane) membrane injecting its genetic material into the host cell Lytic & lysogenic cycles (HL) Viral genome enters the host cell & undergoes replication Some switch cycles when exposed to environ. conditions (UV light/chemical stressors) Bacteriophage lambda uses 2 methods of viral genome reproduction (host cell): Lytic cycle Lysogenic cycle 1. Viral genome takes over the host cell Long time - virus remains - dormant state machinery → causes rapid production 1. Bacteriophage genome enters the host of viral particles cell becoming integrated with the host 2. Host cell is lysed, destroying it - new genome. viral particles release into the 2. Host cell reproduces - new cells contain environment the bacteriophage genome, (prophage). Prophage genome may be cut out from the host cell genome, forming bacteriophages & beginning the lytic cycle - Parts of the prophage remain in the host cell genome, or taken w. - prophage. The origins & evolution of viruses (HL) Possible origins of viruses: virus structure & genetic material diversity - suggests: 1. Encapsulated Originated from ancient RNA/DNA molecules Genetic encapsulated in a capsid which replicated & evolved material 2. Evolution Evolved from viroids (small infectious agents consist of from Viroids a short strand of RNA) that infect angiosperms (flowering plants) 3. Transposons Originated from transposons (genetic elements that move within genomes) 4. Ancient cells Evolved from ancient cells that lost independent living ability, becoming dependent on host cells - reproduction The possibility of convergent evolution in viruses: :unrelated organisms evolve similar traits in response to adapting to similar environments. - Occurred b.c of a limited num. of ways, shapes & sizes optimal for viruses to effectively infect & replicate in host cells. Thus diff. viruses arrived at similar solutions through diff. evolutionary pathways. Viruses demonstrate convergent evolution: Similar entry Viruses infecting diff. cells - evolved similar mechanisms to enter & mechanisms replicate Immune Viruses that infect diff. types of host organisms evolved similar system mechanisms to evade the host’s immune system & spread to new evasion hosts. Shared shapes Viruses evolved similar structures due to limited effective shapes & sizes for infecting & replicating within host cells. Reasons for the rapid rates of evolution in viruses: High mutation rates RNA viruses (retroviruses) have high mutation rates b.c they copy their genetic material using error-prone mechanisms (reverse transcriptase enzymes & RNA polymerases) - less accurate than DNA polymerases. - Mutations passed to next generation (virus progeny) - Harder to treat, vaccinate & immune sys. - recognise & control these viruses Genetic exchange Viruses exchange genetic materials through recombination & horizontal gene transfer - to rapidly acquire new traits/adapt to new environments Short generation Short generation times & high reproductive rates opportunities times for mutations & evolution. Rapid evolution of RNA viruses - case studies: HIV Transmitted through bodily fluids & infects CD4+ cells (immune system) leading to AIDS (no cure). Evolves rapidly due to high replication rates - thus mutation rates from error-prone reverse transcriptase & recombination (exchange RNA - viruses/host cell - acquire new genes & traits) enable HIV to adapt & evade immune responses & treatments. - Lead to new HIV strains with varied virulence/drug resistance - complicate treatment & vaccine development Influenza High influenza mutation rates in Haemagglutinin & neuraminidase (surface proteins) reduce immune recognition & flu vaccine efficacy. Reassortement: Exchange of genetic material between different strains of influenza from a host cell already infected - can create new strains with diff. characteristics - potentially pandemic-causing SARS-CoV-2 SARS-CoV-2 spike protein mutations aid in host cell recognition & (Caused COVID-19) cell membrane fusion. - Some mutations - deleterious/no effects on the virus & some transmissibility reduce vaccine efficacy, & aid immune evasion Accumulated genetic recombination & mutations result in variants: SARS-CoV-2 Delta & Omicron variants. - Freq. mutations in RNA seq. - used to track viruses transmission & developing public health measures. A3.1 - Diversity of organisms Large amount of variation between organisms Species: :a group of organisms capable of producing fertile offspring (Interbreeding) Morphological species concept Biological species concept :organisms classified into species based on :species classified by the ability to interbreed & morphological characteristics (Italics) produce fertile offspring. - Take into consideration - sexual Binomial nomenclature dimorphism (in a species & males & Species are named - genus (capitalized) & females have diff. characteristics) species names (lowercase) - Species sharing - genus have common If 2 members - diff. species interbreed = characteristics. infertile offspring (hybrid) b.c of mismatched chromosome numbers * Rare cases of fertile offspring - E.g: Donkeys have 62 chromosomes, horses have 64, & their hybrid offspring - mules, are often infertile due to the uneven chromosome number. Diversity in chromosome numbers of plant and animal species Eukaryotic DNA is organised into chromosomes (nucleus) Chromosome numbers vary by species but remain consistent within a species - Humans have 22 pairs of autosomes & 2 sex chromosomes (X/Y) = 46 chromosomes. - Chimpanzees (share 98% of human DNA) have 23 pairs of autosomes & 2 sex chromosomes = 48 chromosomes. Diploid cells contain an even num. of chromosome pairs Difficulties distinguishing between populations & species due to divergence of non-interbreeding populations during speciation Speciation: 1 pre-existing species splits into 2+ species. Caused for species splitting that prevent/limit interbreeding between 2 populations: 1. Reproductive barrier 2. Physical barrier 3. Change in behaviour/timing around reproductive cycles 4. Chromosome number changes from errors in cell division - chromosomes don't separate - producing cells with 2x chromosomes → result in new species. 5. Speciation events are slow after the physical population separation. Karyotyping & karyograms :diagnostic techniques to identify sex & chromosomal abnormalities Karyotyping: determining the karyotype - number, size & shape of chromosomes 1. Isolate condensed chromosomes from actively dividing cells 2. Individual chromosomes are stained to highlight banding patterns - images are arranged using by size, banding patterns & centromere location to create chromosome pairs (karyogram) - Isolated at the right stage of cell division, replicated chromosomes appear as sister chromatids joined at the centromere. E.g: Human male Karyogram - 22 pairs of autosomal chromosomes & 1 pair of sex chromosomes. Chromosomes are paired & ordered by size/length, centromere location & banding patterns. Sex chromosomes are mismatched in length - males (XY) & matches in females (XX) - X- longer with more genes & Y - has fewer, male-specific genes Why do Humans & great apes have diff chromosome numbers? Human chromosome 2 resulted from the fusion of chromosomes 12 & 13 in great apes. Evidence: 1. Gene sequences on chromosome 2 match those on great ape chromosomes 12 & 13. 2. Telomeric DNA, typically at chromosome ends (to protect) is found in the fusion region of chromosome 2. 3. A second, mutated, & nonfunctional centromere exists on chromosome 2. Genome sequencing - samples of Neanderthal & Denisovan DNA (fossil evidence) show 46 chromosomes like modern humans. - Suggesting the fusion of chromosomes 12 & 13 occurred before the separation of modern humans, Neanderthals & Denisovans from their common ancestor Comparing genomes : total genetic information of an organism. - Determine the organism's physical characteristics - Store information that directs the development & function of cells - Regulate the expression of genes. Species have the same genome - SNPs contribute to variation & diversity (a single nucleotide is replaced with another) Differences in eukaryotic genomes between different species: - Size (amount of genetic material determined - num.of base pairs) - Base sequences (determine how DNA is expressed - species genetic characteristics) *Genome size is compared using online databases - storing genetic data of sequenced species. Advantages & disadvantages of gene sequences: Advantages Disadvantages Genome seq. - diff species → investigation Slow & costly into the genetics & evolutionary Ethical concerns such as potential relationships discrimination in insurance & Personalized medical treatments & drugs employment. based on the sequencing of our genomes/genomes of the microorganisms that live symbiotically in us - Advances in our health & wellbeing Revision of classification systems - reorganization (e.g: red panda's reclassification into the superfamily - Musteloidea) Exceptions to the biological species concept (HL) Evidence of interbreeding btwn. modern humans & Neanderthals - some populations of humans have Neanderthal DNA in their genomes. - Suggests fertile offspring btwn. 2 species to pass the DNA Asexually reproducing species & horizontal gene transfer Asexually reproducing species challenge concept b.c they are not capable of interbreeding & producing fertile offspring Prokaryotes (Bacteria) Hydra Reproduce through binary fission, Reproduce through budding producing producing genetically identical offspring genetically identical offspring grown on without interbreeding. the parent without interbreeding. The biological species concept doesn't apply to asexual reproduction - difficult to classify these species Prokaryotes also undergo horizontal gene transfer (genetic material is exchanged btwn individuals of diff. species - increasing genetic variation among prokaryotes despite asexual reproduction.) - Further complicates species classification Identifing species with new/incomplete specimens (fossils) (HL) Dichotomous key: identify species using yes/no questions based on physical characteristics Environmental DNA barcoding :technique for analysing environmental samples to identify the DNA of organisms & measure biodiversity 1. DNA is extracted from environmental samples (water/soil) 2. Specific DNA sequences are identified to create a barcode for the unknown specimen - Seq. selected show more variation bwtn. species than in species - distinguish diff. species 3. The unknown barcode is compared with a catalogue of known barcodes for identification Applications of DNA Barcoding: 1. Identify species from environmental samples without direct observation. - Useful for detecting elusive/endangered species. 2. Measures & monitors biodiversity, indicator of ecosystem health & sustainability. 3. Test food for microorganism contamination 4. Identify species from partial specimens, diff. life cycle stages & stomach contents. 5. Agriculture: identify the species of an insect pest for effective pest control measures. A3.2 - Classification and Cladistics (HL) Classification Why is a classification system needed (Advantages)? : Better organize & understand the world by grouping similar things/ideas. Better communication by providing a common language to discuss or refer to certain groups of things. Be more efficient - being able to quickly, easily find & categorize things & information. Allows for further research & analysis to identify patterns & trends to understand classified systems & phenomena Carl Linnaeus: developed the system of classification & nomenclature Intermediate taxa (subfamilies & superorders) (account complexities in relationships btw groups) - Species were classified based on their morphological characteristics Challenges of the traditional hierarchy of taxonomy Traditional taxa are arbitrary (does not reflect the gradation of variation) & don’t reflect evolutionary patterns (divergence) Similar traits can appear in unrelated groups due to convergent evolution (bats & insects have wings, but their evolutionary origins differ) Genetic evidence lead to reclassifications of species based on DNA evidence (e.g: Initial relation - similar diet & habitat - genetic studies show giant pandas belong to the bear family (Ursidae) & red pandas are related to raccoons & skunks (superfamily Musteloidea) Advantages of Genetics-Based Classification (comparison - nucleic acid/amino acid seq.) (paradigm shift): 1. Cladistics better reflects evolutionary relationships, grouping organisms in clades based on shared ancestry. 2. Reflects - process of evolution, showing branching speciation patterns (phylogenetic trees) 3. Enables predictions about biochemistry, behavior/ecological roles based on evolutionary relationships 4. Develops understanding of biodiversity by contextualizing evolutionary & distribution history Cladistics Finds evolutionary relationships btwn. species based on shared traits & genetic evidence - Comparison of molecular seq. (DNA)/physical traits of group (organism) - evolutionary history of diff. species (evidence - species belong in which clades) (e.g: Chimpanzees are more closely related to humans than gorillas) - Compare base sequences of the cytochrome c gene Molecular evidence more reliable & unbiased classifications than physical traits by avoiding issues from phenomena; convergent evolution & mimicry Clades: group of species with a single common ancestor & a set of common traits/characteristics. - Hierarchical - subdivided into smaller groupings E.g: clade of mammals are divided into subclasses (Prototheria & Theria) with further divisions into infraclasses (Metatheria & Eutheria) Comparison of base & amino acid seq./traits of organisms determine traits from - common ancestor Gradual sequence differences for estimating clade divergence - The greater the time since 2 species diverged from a common ancestor, the greater the difference in sequence - b.c there would be more time for mutations Molecular clock: measures the time since 2 species shared a common ancestor based on the gradual accumulation of genetic differences over time. - Estimates b.c mutation rates have other determinants: - Population size - Selection pressures - Genome size - Degree of randomness - E.g: Using the molecular clock it is estimated humans & chimpanzees diverged 6 million years ago, with a possible margin of 1–2 million years (estimate - no fossil of recent common ancestor) Worked example 1 Q: How long ago did two species share a common ancestor if their mitochondrial gene differs by 13 bases, given a mutation rate of 3 every 5 million years A: 3 mutations occur per 5 million years - 1 mutation occurs ddper 3/5 million years. 13 ÷ (3/5 million) = 21.7 million years ago Worked example 2 Q: If the mutation rate in non-coding DNA is 2 mutations every 150,000 years, how long ago would species A & species B have shared a common ancestor if their DNA differs by 19 bases? A: (2/150,000 years) = 1/75,000 mutations per year Uu19 ÷ (1/75,000) = 1,425,000 years ago Calibration points are needed - molecular clock estimates (known time when 2 species diverged) - E.g: Oldest fossil of a species is a calibration point - allows the molecular clock to estimate the divergence time of other species based on this reference Base sequences/amino acid sequences are used to create cladograms Used to compare the traits of various organisms & explore evolutionary relationships 1. Identify the group of organisms 2. Choose traits (morphological/molecular) - to be compared & used to determine evolutionary relationships 3. Info about traits is used to build a matrix 4. Matrix is used to create a cladogram - visualizes & infers evolutionary relationships DNA is evident for common ancestry btwn. species b.c: - All living organisms use DNA as their source of genetic information, the genetic code is universal & mutations occur gradually over time. E.g: A cladogram of vertebrates - morphological traits compares among species in a matrix to determine evolutionary relationships & visualised by a cladogram - Vertebrate cladograms show mammals with fur are placed in a group - are more closely related to each other than to other vertebrates. Organisms are placed based most probable evolutionary relationships (required fewest evolutionary changes) The more "evolutionarily efficient" relationship is selected, where fewer genetic/trait changes are needed to explain the diversity within a clade. - E.g: Monotremes (echidna, platypus) lay eggs are placed in the mammal clade. - Logical placement b.c it requires fewer sequence changes/new characteristics to explain their placement in the mammal clade than in a separate or bird/reptile clade. another clade such as birds or reptiles that also lay eggs. - Avoids unnecessary reclassification & maintains the most efficient evolutionary path Applying cladistics (HL) Hypothetical ancestors & descendants - branching diagram Root - most ancient common ancestor Node - hypothetical common ancestor Terminal branch - extant species (exists) Cladogram analysis - Assumptions 1. If extinct species included - cladograms rely on morphology due to no DNA to sequence 2. More nodes between species - more distant evolutionary relationships 3. Mutations in DNA & proteins occur at a constant rate. E.g: 1 base change per 10^9 years 4. Cladograms are drawn to scale - length of branches proportional to the divergence time E.g: Human & chimps - most related Lemurs are least related Cladograms can estimate when species diverged & when the common ancestor existed - Set speciation to a timeline after analysing & comparing molecular diffs. to fossil records - E.g Humans & chimpanzees diverged 6-7 million years ago. Molecular data in cladistics - reclassification of group evolutionary relationships Figwort family: - Flowers initially classified based on morphology belonging to 1 clade - Molecular data: genetic analysis of chloroplasts genes showed many diff. species within the group - not all come from 1 common ancestor - reclassified into 5 families *Some figwort genera moved to other families - plantain & broomrape. Less than half of the plants once classified as figworts remain in the figwort family due to new DNA evidence (info. - DNA similarities & diffs.) Three-domain classification of organisms using rRNA sequences 1. Eubacteria 2. Archaea 3. Eukarya A4.2 Conservation of biodiversity 3 levels of biodiversity (interconnected & impacts each other): Genetic :variation in the genes of a species population biodiversity - Mutations result in diff. versions of the same genes (alleles) Higher genetic variation in the gene pool the probability of adaptive alleles in changing environments - for evolution & reproduction Differential reproduction - genes are passed down generations for the species survival E.g: DDT (insecticide) - initial decreased mosquito pop. DDT-resistant mosquitoes evolved due to genetic variation, which included DDT-resistance genes & passed on the gene to offspring → population of DDT-resistant mosquitoes. Low genetic diversity reduces survival chances (endangered species) Inbreeding in isolated populations reduces genetic diversity & leads to genetic uniformity - reduces allele diversity in the gene pool Conservation efforts increased the Bison population, but genetic diversity is low due to the genetic bottleneck effect. Species :variety of species in a habitat diversity Depends on: 1. Species richness 2. Species evenness (relative abundance) Ecosystem Ecosystem: community of organisms interacting with their abiotic envir. diversity Ecosystem diversity includes terrestrial and aquatic ecosystems within a geographical area. E.g: Rainforests have ecosystems like canopy, understory, & forest floor. Comparing biodiversity Fossils provide evidence of species diversity over time - current species diversity is higher than in the past. Biodiversity cataloguing is slow due to inaccessible habitats - undiscovered organisms & species are becoming extinct before discovered Causes of anthropogenic species extinction (sixth mass extinction) 1. Overexploitation due to overhunting/overharvesting - E.g: Dodo bird - slow, unable to fly & had no natural predators - overexploitation by sailors and habitat destruction → extinction 2. Habitat loss from deforestation, agriculture, & urbanization 3. Invasive species outcompete or prey on native species 4. Habitat degradation due to pollution 5. Climate change Case studies of anthropogenic species extinction: Moas Fossil studies & bone DNA - overexploitation of human hunting. The Polynesians found flightless moas to be an easy food source, hunting, rats (ate chicks) & diseases - led to their extinction. Caribbean Monk Fishermen killed seals - oil lamps & machinery grease. Docile & Seal non-agressive - easy hunting for blubber. Overfishing decreased food sources. Splendid Poison Declined due to habitat loss from logging, agricultural conversion, Frogs urbanization, trafficking for the pet trade further & fungal disease that affecting tropic amphibians - extinction. Tasmanian Wolf Hunted by European settlers due to its predation on sheep. Dingoes, outcompeting the wolves for food (invasive species. Pets & exported to zoos. Causes of ecosystem loss Ecosystem services (process/outputs of the ecosystems humans benefit from): Supplying resources Providing basic services essential for survival Case studies on ecosystem loss: Dipterocarp Keystone species (vital for biodiversity & ecosystem services): forests - Support forms of life (Southeast Asia) - Provide food for herbivores through leaves, fruits, & seeds - Decompose - their leaves enrich the forest floor - Roots stabilize soil - Offer shelter to arboreal animals, birds, & epiphytic plants - Canopy shelters the forest layers from torrential rain - Store carbon - help stor CO₂ helping combat climate change Threats: - Destruction from logging for timber - Palm oil plantations (CO₂ emitted from burning land) puts endangered species (elephants & orangutans) at risk. Coral reefs Coral reefs benefits: - Sustain food webs - Provide people with food - Sources for new medicines - Protect the coastline from erosion & storms Threats: - Pollution from fertilizer runoff, hot water from power plants rubbish, plastic, & oil spills - Destructive fishing practices (overfishing, explosives & cyanide use) - Habitat loss due to coastal development - Damage from unsustainable tourism (careless diving) - Climate change causing rising ocean temperatures & acidification due to increased absorption of CO₂ leading to coral bleaching - vul. to diseases & affects other species Evidence for the biodiversity crisis IPES (intergovernmental org.) reports - asses & strengthen biodiversity & ecosystem services IUCN - Red lists of threatened species & ecosystems - inform conservation actions & policies Expert & citizen scientists - local data collection to answer research Qs. Horseshoe count - A census is conducted by citizen scientists to monitor the number of organisms & prevent overharvesting. Biodiversity surveys gather info on resource use & species conservation (repeated in an area) Population growth - demand for resources - pressure leads to overexploitation of natural resources & habitat destruction Hunting & Overhunting & fishing deplete species faster than they can replenish. overexploitation Hunting sport, body parts & poaching - despite protective laws. Destruction of Demand for space leads to habitat loss. Deforestation, river damming, & habitats wetland filling alter ecosystems - species can’t survive in org. habitats. Habitat Urbanization divides natural habitats into smaller patches, restricting animal fragmentation movement, reducing resources, & increasing extinction risks. - Building wildlife corridors can help animals move safely between fragmented habitats. Invasive Human colonization introduced invasive species - disrupting ecosystems by species outcompeting native species, spreading rapidly & altering food chains. Pests & Global transport of animals and products has facilitated the spread of pests diseases and diseases. (e.g: trade in amphibians has spread a harmful fungus & the chestnut blight, by a fungus from Asia decreased chestnut trees) Pollution E.g: Acid rain, caused by sulfur dioxide & nitrogen oxides from industrial emissions, harms plants and animals. Plastic pollution in oceans - threat to marine life ingesting plastic. Pollution also provides breeding grounds for pests - rats & cockroaches. Conservation of biodiversity - maintaining biodiversity - protection & sustainable resource use. In situ conservation Ex situ conservation Protecting species in their natural habitats Species are conserved outside their natural through national parks, wildlife sanctuaries, & habitats in zoos, botanical gardens & aquariums marine reserves. Species too small to sustain/high poaching risk - Preserves natural behaviors and - Scientists establish gene backs to store ecosystems, & supports public biodiversity by (storage of germplasm - awareness through responsible tourism. seeds, sperm, & DNA) - Seed banks - wild strains of food crops - conserve genetic info - Tissue banks - tissue samples from plants Conservation strategies: Captive Helps prevent a species extinction by breeding animals in controlled breeding environments (captive training & encouraged to breed then reintroduced into the wild) - Small populations inherently have a lower genetic diversity - chances of passing unfit genes. Rewilding Restoring habitats to their natural state by reintroducing species that were lost due to human activities, aiding ecosystem recovery. Reclamation of Land restoration strategies, supported by local communities, aim to recover degraded ecosystems damaged by human activity - deforestation & soil erosion. ecosystems EDGE species :Conservation program - prioritizes evolutionarily unique & globally endangered species. (only surviving members of their genus/even higher taxa & unique in appearance, behaviour & genetic makeup) Species are selected according to their (ED score): Evolutionary Distinctiveness (ED) score - determined phylogenetic tree position - Higher for species that are evolutionarily more distinct - fewer closer relatives. Globally Endangered (GE) score - calculated by the IUCN Red List - more endangered - higher score. Worked example 1 Q: Identify the species that would have the highest ED score on the hypothetical evolutionary tree ? (Branches - represents millions of years of evolution) A: Species A has a higher score - alone on the branch B & C have close relatives B1.1 - Carbohydrates & lipids Carbon-carbon - longer chain - more strong & stable mol. (more cov. bonds) C bond with non-metallic elements Macromolecules : large molecules - made of monomers (subunits - link together to form polymer) Formation of macromolecules by condensation reactions : polymerisation reaction where 2 molecules join releasing a water molecule - 1 molecule loses a hydroxyl group (−OH) & the other loses a hydrogen atom (−H) releasing a H2O molecule & forming a covalent bond. E.g: 2 glucose mol. Join to form carbohydrate mol. - −OH group on carbon 1 of one glucose molecule attaches to the −OH group on carbon 4 of the other glucose molecule - cov. bond btwn. 2 molecules: 1,4-glycosidic bond & release of 1 water mol. Disaccharide: 2 monosaccharides bonded together Polysaccharide: 2+ monosaccharides bonded together (e.g: starch & glycogen store glucose in plants & animals ) Breakdown by hydrolysis reactions :H2O molecules break cov. bonds btwn. monomers in a polymer - Releases monomers, making them usable for energy/new macromolecule synthesis. E.g: Disaccharide sucrose undergoes hydrolysis to break down into monosaccharides (glucose & fructose) - −OH group from a water molecule attaches to 1 monosaccharide, & the −H attaches to the other, breaking the glycosidic bond Condensation & hydrolysis allow for recycling of monomers & synthesist of new polymers needed for cellular processes Carbohydrates Monosaccharides: single sugar unit - classified by num. of C atoms Properties & uses of glucose: Glucose has 2 alpha-glucose (α-glucose) & beta-glucose (β-glucose) isomers Difference is the orientation - hydroxyl (–OH) group on the first carbon atom: - In α-glucose –OH group is oriented downwards - In β-glucose –OH group is oriented upwards. - Β - if the glycosidic bond is upwards /o\ & α mol. - downwards \o/ The type of isomer determines the structure of polysaccharides: - Starch & glycogen are composed of α-glucose. - Cellulose is composed of β-glucose. Soluble molecule Glucose is soluble (hydrophilic) due to its polarity b.c - molecular structure: - Contains several polar -OH groups - form H-bonds with water - O₂ atom has a partial (-) charge linked to C-H groups with a (+) charge In water - glucose is in equilibrium btwn. its cyclic (ring) & open-chain forms (C1 atom rotates & alpha and beta are randomly assigned) - Only in polymer formation does the C1 atom become fixed in the α or β configuration. Solubility enables glucose to dissolve in blood plasma(-OH groups can form H-bonds with water in the plasma) & be transported to cells for cellular respiration. Stable molecule The cyclic structure & arrangement of -OH groups in axial regions make glucose chemically stable. Stability is crucial for: - The structural role of the polysaccharide cellulose in plants. - Energy storage in starch (plants) and glycogen (animals). Can be oxidised Oxidation: loss of electrons from an atom/mol. Reduced: mol. that gains elections Production of ATP - cellular respiration: - Glucose (C₆H₁₂O₆) is oxidised (losing electrons to O₂) to produce carbon dioxide (CO₂) and water (H₂O). - Energy released is used to generate ATP. Polysaccharides as energy storage compounds (composed of α-glucose): Starch (plants) Glycogen (animals) Structure Amylopectin - Branched Branched polymer of glucose molecules polymer of glucose, with (coiled) α-1,4-glycosidic bonds in - Linear chains of glucose linked by linear regions & α-1,4-glycosidic bonds form a α-1,6-glycosidic bonds at backbone branch points (coiled) - Branch points occur every 8–12 glucose units with α-1,6-glycosidic bonds. Amylose - Linear polymer of glucose monomers linked by α-1,4-glycosidic bonds (branching allows for a 3D structure) Properties Coiling & branching during Highly branched structure forms a polymerisation - efficient compact, coiled molecule - efficient storage in limited space. for storage & mobilisation. Insoluble due to large molecular Insoluble due to large molecular size, helping maintain osmotic size, helping maintain osmotic balance in an organism. balance in an organism. Storage & Energy storage - seeds & roots Liver - glycogen maintains blood glucose function levels - liver breaks down glycogen & releases glucose into the bloodstream when levels drop. Muscle - glycogen is quickly mobilised & broken down into glucose mol. used in cellular respiration - energy for muscle contractions during exercise & maintaining energy levels. Breakdown Hydrolysis breaks down starch into Broken down through glycogenolysis glucose when plants need energy. - Glucose released is used as energy for growth & photosynthesis. Polysaccharides as structural compounds: cellulose :polysaccharide composed of β-glucose mol. (cell wall) - β-glucose mol. alternate in orientation forming long, unbranched, straight chains Cellulose chains are grouped into microfibrils - H-bonded together btwn. adjacent cellulose mol. Cross-linked chains & H-bonds btwn. the chains creates a strong stable lattice structure which provides high tensile strength - crucial for maintaining plant cell wall integrity - Provides rigidity & resistance to osmotic pressure. Role of glycoproteins in cell–cell recognition :proteins attached to amino acid residues in carbohydrates (cellular structures: extracellular matrix, cell membranes & secreted proteins) Function of glycoproteins: 1. Cell–cell Act as cell-surface markers for cellular identification - interact recognition appropriately. Enable immune cells to recognize & attack foreign cells with diff. glycoproteins (e.g: viruses/bacteria) 2. Receptors Act as cell-surface receptors to receive signals from cells /molecules in the environment. - E.g: Insulin binds to glycoprotein receptors, triggering events that lead to glucose uptake by the cell. 3. Ligands Act as ligands - glycoproteins bind to specific receptors on other cells to initiate signalling pathways. 4. Structural Contribute to cell & tissue integrity - forming part of the extracellular support matrix. ABO blood groups - Based on which glycoproteins & glycolipids (A & B antigens) are present - surface of RBCs Blood type/group & their corresponding glycoprotein: Type A Type B Type AB (universal Type O (universal recipient) donor) Has A antigen Has B antigen Has both A & B Has neither A nor B antigens antigen Compatibility of blood types is based on recognition & interaction of specific glycoproteins on the surface of RBCs. - Incompatible blood types mixed - immune system can recognise the other glycoproteins as foreign molecules & attack - leads to the clumping of RBCs (organ failure & death) Lipids - Non-polar molecules with low water solubility (hydrophobic) - Insoluble in aqueous solutions but dissolve in non-polar solvents due to similar polarity. Types of lipids: Triglycerides Wax Steroids Consumed & synthesized by the Water insoluble & high melting 4 C rings fused together liver point - solid at room temp. (butter, lard & gee - solid at (leaf surfaces - waterproof layer room temp.) reducing rate of transpiration) Formation of triglycerides and phospholipids (lipids) by condensation reactions Triglycerides Phospholipids Formed by condensation reactions btwn 1 Composed of a glycerol molecule, 2 fatty acid glycerol mol. & 3 fatty acid mols. & 1 phosphate group - 3 fatty acids attach to 1 glycerol mol. - 2 Fatty acids attach to 1 modified forming 3 ester bonds, releasing 3 water glycerol phosphate mol. forming 2 ester mols. bonds, releasing 2 water mols. Result: A triglyceride mol. with 3 ester bonds. Result: A phospholipid with 2 ester bonds Difference between saturated, monounsaturated and polyunsaturated fatty acids Type of Fatty acid Structure & examples Properties Saturated - No double bonds btwn. C atoms - Straight, linear shape allows tight in the hydrocarbon chain. packing. (Animal fats & tropical oils) Monounsaturated - 1 double bond in the - Single kink in the chain. khydrocarbon chain. - Liquid at room temp. - Lower melting point than saturated (Oleic & Palmitoleic acid) Polyunsaturated - 2+ double bonds in the - Multiple kinks in the chain not tightly khydrocarbon chain. packed (Linoleic acid - veg. oil & Alpha-linolenic acid - fatty fish) Effects of Fatty acid saturation and structure on properties: Unsaturated - Liquid room temp & lowest melting point. Contain double bonds - the more double bonds the lower its melting point - Double bonds disrupt regular packing, making it easier to break apart & transition into a liquid. Saturated - Solid at room temp & high melting point. No double bonds - linear shape allows tight packing, requiring more energy to break apart Cis & Trans Cis: H atoms located on the same side of the double bond - creates a kink, Unsaturated making the structure less linear & more flexible. Found naturally. Trans: H atoms located on opposite sides of the double bond; forms a rigid & linear structure. Created industrially. Energy storage in plants & endotherms: Plants Endotherms - Store fats (unsaturated) in seeds - :animals - rely on metabolic reactions - heat & provides energy for germinating maintain constant internal temps. seedling until photosynthesis begins - Store fat in adipocytes - metabolized into ATP for cellular processes. Triglycerides in adipose tissue for energy & thermal insulation: - Serve as thermal insulators & energy reserves in the cold E.g: Whale blubber (composed of adipose tissues - contain triglycerides) provides insulation & energy storage - survive for long periods without food Phospholipid bilayer (Cell membrane) Phospholipids (amphipathic) A glycerol backbone attached to 2 fatty acids : - (-) charged phosphate head - polar & forms H-bonds with H2O - hydrophilic - Hydrocarbon tails - 2 non-polar fatty acid chains (saturated/unsaturated - kink) - hydrophobic. Placed in aq. environment: - Hydrophilic heads face the aq. solution - Hydrophobic tails face inwards (more attracted to themselves than water) Steroids (lipid) : org. compounds - 3 hexose rings & 1 pentose ring - Steroids - cholesterol the stability & flexibility of the bilayer - Hormonal signaling for reproductive development - oestradiol & testosterone Steroids are non-polar - hydrophobic & can pass through the bilayer - allows the cell to detect & respond to steroids signals more quickly B1.2 - Proteins : macromolecules composed of 1/+ amino acid chains Amino acids - monomers of proteins Central carbon - alpha (α) carbon & is cov. bonded to 4 diff. chemical groups: 1. Carboxyl group (–COOH) 2. Amino group (–NH2) 3. H atom (–H) 4. Unique org. side chain - R-group Condensation reactions form dipeptides & longer chain of amino acids Amino acids join through a condensation reaction to form a peptide bond - Forms btwn. the carboxyl group (–COOH) of 1 amino acid & the amino group (–NH2) of another, releasing 1 water molecule. - A peptide bond is a stable covalent bond. N-terminal end - free amino group not involved in the peptide bond. C-terminal end - unbound carboxyl group. - Additional amino acids can be added to the dipeptide - by peptide bonds btwn. an incoming amino acid & the C-terminal - Forms longer chains of amino acids - polypeptides Dietary requirements for amino acids Essential Non-essential :cannot be produced by the body & must be :produced by the body from other amino obtained through the diet. acids/protein breakdown. - Crucial for growth, maintenance & - Not required in the diet, still have repair of tissues & organs important functions A balanced diet - combination of protein to meet the body's amino acid needs. - Vegan diets - all essential amino acids - adequate amount of plant-based protein sources Infinite variety of possible peptide chains Genetic code: set of rules that translates DNA information into the sequence of amino acids in proteins. - Universal & provides the blueprint for protein synthesis - occurs through transcription (DNA to mRNA) & translation (mRNA to amino acid sequence). The genetic code consists of codons (groups of 3 nucleotides) - specify amino acids/stop signals. There are 64 codons but only 20 amino acids - genetic code is degenerate (multiple codons can code for the same amino acid) - This redundancy allows for silent mutations - changes in DNA don't affect the amino acid sequence. Variety of peptide chains - combining the 20 amino acids in diff. sequences, leads to a limitless range of proteins with diverse structures & functions. Codon table - codon GCU codes - amino acid alanine. Polypeptides: amino acid chains linked by peptide bonds Protein: 3D structure of 1/+ polypeptide chains - Protein made of 1 polypeptide chain - amino acids will fold into a functional protein - In multi-chain proteins, polypeptides interact to form the protein structure. Single-chain polypeptide functions - linear amino acid chain (enzymes & hormones): Lysozyme Enzyme (129 amino acids) found in tears & saliva - Antimicrobial properties & disrupts bacterial cell walls, providing defense against infections. Alpha-neurotoxins Group of polypeptides in snake venom (60-75 amino acids) that target & disrupt the nervous system. - Bind & inhibit specific receptors, causing neurotoxic effects, paralysis & death. Glucagon Hormone (29 amino acids) that regulates blood sugar levels. - Secreted from the pancreas when glucose levels are low, stimulating the liver to release glucose into the bloodstream. Myoglobin Oxygen-binding protein in muscle tissue (153 amino acids) - Stores & releases oxygen to muscle fibers, particularly during low-oxygen conditions - intense physical activity. Effect of pH & temperature on protein structure Structure of proteins is critical for biological function & structural changes - loss of activity. Denaturation: process where the structure of a protein is altered, leading to loss of function, often permanently. Factors causing denaturation: pH Temperature Extreme pH changes alter the protein's High temperatures break H-bonds holding the charge, affecting solubility & shape, leading to protein structure, causing unfolding & loss of structural changes & loss of function. function. - E.g: Enzyme pepsin functions in acidic Human proteins function optimally at body environments & becomes inactive in temp.(~37 °C). alkaline conditions. - Proteins in extremophiles have proteins that only function at high-temps. Low temperatures - less severe effects to protein structure. Worked example 1 Q: Protein contains 18 amino acids - what is the min. num. of bases the gene coding for the jjjjjjprotein could consist of ? A: Protein consists of 19 amino acids, it requires 19 codons in the mRNA sequence to code for jjjjjjthem (3 base pairs) - 19 X 3 = 57 Protein structures (HL) Amino acids are linked together by ribosomes to make polypeptides - Amino acid - amine group & carboxyl groups are used to link amino acids - R-group gives each amino acid its properties Primary :specific sequence of amino acids joined to form a polypeptide chain. - Sequence determines how the polypeptide chain folds - 3D structure - Seq. mutations change - function & structure Secondary :folding patterns in the polypeptide chain - H-bonds btwn. amine & carboxyl groups of diff. amino acids in the polypeptide chain (collectively stabilize the structure) Alpha helices Beta-pleated sheets Coils formed by H-bonds btwn. the Sections of the polypeptide chain run amine H of 1 amino acid & the Parallel - H-bonds form btwn. adjacent carboxyl oxygen of another, 4 residues strands creating a pleated sheet-like away in the seq. structure. Tertiary :further folding of the polypeptide chain - Depends on interactions btwn. R-groups: H-bonding - btwn. polar R-groups - stabilises by holding distant regions of the chain Ionic bonds - btwn. oppositely charged R-groups (undergoes dissociation of H-ions +/- charge) - stability & function. Disulfide cov. bonds - cov. bonds btwn. 2 cysteine amino acid residues (sulfur atoms), Hydrophobic interactions - btwn non-polar amino acids cluster inside the protein to avoid water (polar) - structural stability Quaternary :Interaction of 2/+ polypeptide chains to form a functional protein. E.g: Haemoglobin - 4 polypeptide chains: 2 ɑ-chains & β-chains - conjugated protein, associated with haem (non-protein component - contains an iron atom - center for binding O₂) Quaternary structure enables haemoglobin to transport O₂ - 4 subunits of haemoglobin are held by non-cov. bonds. & interactions btwn. subunits allow haemoglobin to undergo conformational changes. Effect of polar and non-polar amino acids on tertiary structure of proteins Polar amino acids - hydrophilic & orient towards the aqueous environment on the protein’s surface. Non-polar amino acids - hydrophobic & cluster in the protein's core to avoid water. - The folding pattern exposes hydrophilic regions to the solvent & buries hydrophobic residues in the proteins interior - compact structure contributes to protein stability & functionality. Myoglobin (muscle) & hemoglobin (RBC) are globular proteins with hydrophobic cores. Integral membrane proteins have hydrophobic regions that help them embed in membranes. Rhodopsin - retinal membrane protein - absorbs light - has hydrophobic regions Conjugated & non-conjugated proteins: Conjugated Non-conjuated :have non-protein competents (metal :consists only of polypeptide subunits ions/carbohydrates & polypeptide subunits E.g: Collagen - 3 polypeptide subunits & - a protein’s diversity & functionality Insulin - 2 polypeptide subunits E.g: Helps enzymes perform - catalytic functions Haem contains iron - enables haemoglobin to bind O₂ After synthesis, proteins fold into 3D shapes depending - amino acid seq. & cellular environment - them proteins take final form - globular/fibrous Globular & fibrous proteins: Characteristics Example Globular proteins - Spherical shape with Insulin (hormone) - composed of 2 irregular folds. polypeptide chains (ɑ-chain & β-chain) held - Soluble in water. together by H-bonds, hydrophobic - Serve as enzymes, interactions, & disulfide bonds. transporters, & regulators. Function: Regulates blood glucose levels - binds to cell receptors enabling glucose uptake for energy or storage. Structure: Compact & globular with a hydrophilic exterior (interact with water in the bloodstream). Hydrophobic interior stabilizes the protein's shape for receptor binding. Fibrous proteins - Long, narrow shape with Collagen - abundant & found in connective repeating structures. tissues - 3 polypeptide chains twisted into a - Insoluble in water. triple helix. - Provide strength & - Chains contain glycine, proline, & structural support to tissues hydroxyproline residues - allow chains like skin, tendons, & bones. to twist - held by H-bonds & van der Waals forces. Function: Strong, flexible fibres resist forces, providing support & maintaining tissue integrity. B2.1 - Membranes & membrane transport Membranes surround cells & organelles - act as barriers controlling the exchange of materials Composed of: - Lipids (phospholipids, glycolipids & sterols - cholesterol) - Proteins - Small amounts of carbohydrates (glycolipids & glycoproteins) Lipid bilayers - permeability: Non-polar, Small, uncharged Large uncharged Ions lipid-soluble mols. mols. (Water polar mols. (Hormones CO₂& O₂) ethanol) (Glucose) Easily pass through Mostly permeable - Hydrophilic in nature, Impermeable due to the hydrophobic despite the mostly impermeable the hydrophobic interior. hydrophobic barrier. barrier. Simple diffusion of molecules :movement of mols. down a concentration gradient (high to low) - Spontaneous & passive process (does not require energy) - Results in equilibrium - equal mol. concentration Hydrophobic lipid bilayer restricts simple diffusion of most molecules Non-polar mols. (O₂& CO₂) for gas exchange: Oxygen diffusion Lungs - O₂moves from the oxygen-rich alveoli to oxygen-poor blood (erythrocytes) in surrounding capillaries - blood transports O₂ to tissues. Tissues - O₂moves from RBCs to metabolically active cells where oxygen concentration is lower. Carbon dioxide Tissues - CO₂moves from cells (higher concentration) to blood diffusion (lower concentration) Lungs - CO₂moves from blood to alveoli for exhalation. Membrane proteins - facilitate entry of mols. into cells (asymmetrically oriented across the lipid bilayer): Integral Peripheral :embedded within the lipid bilayer :surface of the membrane - Amphipathic mols. - Hydrophilic in nature with no hydrophobic - Hydrophobic regions interact with the regions. bilayer's hydrophobic interior. - Interact with the hydrophilic heads of - Hydrophilic regions interact with the phospholipids/the hydrophilic regions of bilayer’s hydrophilic heads/aq. integral proteins. environment. Easily removed from membranes without Difficult to isolate - disrupt the bilayer disrupting the bilayer. E.g: Transmembrane proteins (extend across) & non - transmembrane found on 1 side of the bilayer Functions of membrane proteins: Transport proteins Recognition Receptors Enzymes Channel Proteins: Membrane proteins Act as receptors for Membrane proteins Transmembrane act as "name tags" for chem. signals & are can catalyze proteins that form cells, helping in binding sites for mols. reactions. channels/pores a cell–cell recognition. (hormones & E.g: Glucose-6 passage across the neurotransmitters) Crucial for immune -phosphatase, a membrane. system function, Binding triggers membrane Carrier Proteins: allowing the body to intracellular reactions -bound enzyme Undergo distinguish between (endoplasmic conformational self & non-self cells. reticulum) catalyzes changes to move glucose production. molecules across Aid in cell adhesion to other cells/the environment. contribute to cell motility Osmosis :diffusion of water across a selectively permeable membrane from lower to higher solute concentrations - Higher solute concentration in the cell - new movements of water into the cell Water moves until solute concentration is equal on both sides. - At equilibrium, no net movement occurs, but water molecules continue moving randomly. The membrane is impermeable to polar solutes. The hydrophobic interior of the bilayer does not allow polar water mols. to pass t