Biology I Semester 1 Manual 2021 PDF
Document Details
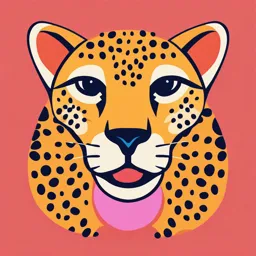
Uploaded by UsefulAltoFlute6041
Sefako Makgatho Health Sciences University
2021
Tags
Summary
This Biology I manual from Semester 1 of 2021 covers key topics in biology including evolution, environmental factors, cytology, genetics, and taxonomy. The manual provides background information, the mechanisms of evolution, and the origin of life. The document originates from Sefako Makgatho Health Sciences University.
Full Transcript
DEPARTMENT OF BIOLOGY BIOLOGY I SEMESTER 1 Theory Notes MBLA010/MBLB010/MBLC010/MBLD010 1. Evolution 2. Environmental 3. Cytology 4. Genetics 5. Embryology 6. Taxonomy & Systematics SEFAKO MAKGATHO HEAL...
DEPARTMENT OF BIOLOGY BIOLOGY I SEMESTER 1 Theory Notes MBLA010/MBLB010/MBLC010/MBLD010 1. Evolution 2. Environmental 3. Cytology 4. Genetics 5. Embryology 6. Taxonomy & Systematics SEFAKO MAKGATHO HEALTH SCIENCES UNIVERSITY FACULTY OF SCIENCE DEPARTMENT OF BIOLOGY FOR INTERNAL USE ONLY Biology I : Evolution Table of Contents: Section 1: Background Information: The “Evolution” of Evolutionary thinking: Page 2 Section 2: The Origin of the Earth, and of Life. Page 5 2.1 The Primitive Earth Page 5 2.2 The Origin of Life Page 5 2.2.1 Conditions favorable for the origin of life Page 5 2.2.2 Stages during the origin of life Page 6 2.3 The geological time scale Page 8 2.4 Development of complex, multicellular life Page 10 2.5 Our wandering continents Page 11 Section 3: The Mechanism of Evolution: Page 15 3.1 Evolution and genetics 3.1.1 Introduction Page 15 3.1.2 Mendelian Inheritance Page 15 3.1.3 Hardy-Weinberg Equilibrium Page 17 3.2 Causes of Microevolution: Page 20 3.2.1 Mutation Page 20 3.2.2 Gene flow Page 20 3.2.3 Non-random mating Page 21 3.2.4 Genetic drift Page 21 3.2.5 Natural selection Page 22 Section 4: Natural Selection Page 24 4.1 Types of natural Selection Page 24 Section 5: Speciation Page 25 5.1 The Species concept Page 25 5.2 Reproductive Isolating Mechanisms Page 25 5.3 Types of Speciation Page 26 Section 6: Evidence for Macroevolution Page 28 6.1 Fossil evidence Page 28 6.2 Anatomical evidence Page 29 6.3 Biogeographical evidence Page 30 6.4 Biochemical Evidence Page 30 1 Section 1: Background Information: The “Evolution” of Evolutionary thinking Biblical / Pre-Darwinian Post-Darwinian The earth is young (created in the afternoon, on 23 October 4004 BC) The earth is old (4 to 4.62 billion years) Species are related by descent; they are also not Each species was created as is; species do not static over time; they can change or diverge or change, and species do not become extinct become extinct Species are adapted to the environment because Species are adapted to the environment of natural selection acting on random genetic because they were divinely created so variation, under certain climatic conditions Early history: - Even though Charles Darwin is generally believed to be the “Father” of Evolution, the idea of evolution certainly predates Charles Darwin by more than 2,000 years. The ancient Greeks already speculated on the possibility of evolutionary change in animals many centuries before the birth of Christ. As early as 504-433 BC, Empedocles suggested that (1) higher forms of life gradually develop from lower forms, (2) plants were present before animals, (3) animals with characteristics which are suited for a particular environment replace those that are less well-adapted. (In this last point we can see early visions of Charles Darwin’s description of Natural Selection). - Aristotle (384-322 BC) proposed that animals are arranged in a “ladder of nature”, starting with inanimate matter, rising up towards plants, then low animals, more advanced animals, and, finally, humans. - Aristotle also suggested that biological matter can arise spontaneously from dead matter. This seemed obvious since – not having any knowledge on microbiology, and not having the technology with which to study micro-organisms – it seemed the most likely explanation for maggots “miraculously” appearing in rotting meat, for example. - For several centuries, this idea had much support, until St. Augustine (354-430 AD) proposed the Biblical account of creation as an alternative. - This view was accepted throughout the Middle Ages, and until the Renaissance when Francesco Redi and Spalanzani managed to disprove this view on spontaneous generation simply by covering rotting meat with fine cloth (1688). This prevented flies from laying their eggs in the meat, and no maggots could “spontaneously be created” inside the meat. - Antoni van Leeuwenhoek later designed the microscope, and discovered the microscopic world, and the exceedingly tiny and apparently primitive organisms that inhabit it (1683). The idea of “Spontaneous Generation of living organisms from non-living matter” was rekindled for many people by the discovery of such primitive animals. -The discovery of the microscopic world set in motion further discoveries on the role of micro- organisms eg. as cause of disease. Louis Pasteur demonstrated in 1861 that many of the examples of “Spontaneous generation” could be explained at the hand of non-sterile techniques used in medicine, food-preparation, storage etc. 2 The Pre-Darwinian Period: An in-depth study of the debate on the origin on life, and the variety of life could fill a book on its own, and would fall outside the scope of this book. Nevertheless, some discoveries / views can be highlighted. - Linnaeus (1700s) - Carolus Linnaeus believed in the fixity of species (This means that species were divinely created to look the way they do, they never change their shape, they never become extinct, and new species are never created). - Species were created ideally suited to their habitat. - They had a specific spot on what he called the Scale of Nature on which the most primitive organisms are placed low on the scale, and with increasing complexity; animals are sequentially assigned higher positions on this ladder of life (similar to Aristotle’s “Ladder of Life”, except that Linnaeus never believed that animals could “spontaneously be created’). - Humans, of course, were placed on top of this ladder. They are the pinnacle, or the top, of all animals. - Linnaeus is well known for devising the Binomial system of Classification by which each species is given a scientific name consisting of two parts (e.g. Homo sapiens, Pan troglodytes, Panthera leo etc.). - Cuvier’s Catastrophism (late 1700s) - Fossils proved that many animals once existed that were no longer alive – particularly animals of kinds unlike any of those living on earth. This means that they had to have become extinct. - This challenged the view of the Fixity of Species, since it seemed that animals could indeed sometimes become extinct, and this contradicted many opinions on the fixity of species. - Since layers in the earth even showed a succession of life forms, the fossil record even seemed to imply that species could change over time. - Different ways existed to try and explain this pattern. Many people looked at fossils of large animals, and believed that they were animals who died in the Great Flood of Noah. Other people looked at fossils (of dinosaurs, for example) and believed them to be creations of the Devil, etc. - Cuvier explained this at the hand of what was later called Catastrophism (periodic local extinctions, followed by repopulation, by closely related species from surrounding areas. In other words, sudden geological or climatic changes may sometimes wipe out the species in an area, following which new species are then created to replace them. A cycle of periodic extinctions in an area, followed by repopulation from surrounding areas, could give an appearance of change occurring over time. - Lamarck (Late 1700s) - The central tenet of Baptiste Lamarck revolved around the inheritance of acquired characteristics. Physical characteristics developed during the life of an animal will be transferred to the children. According to Lamarck, for example, body builders should have more muscular children than non-body builders. - His famous example was the origin of the Giraffe. Giraffes have to stretch their necks to reach the upper branches of trees, to get to more nutritious leaves not reachable by shorter animals. By many generations of stretching their necks, each generation will progressively have slightly longer necks. - The more a giraffe stretches its neck during its lifetime, the longer the necks of its offspring will be. By their efforts, they can therefore bestow an advantage on their offspring. For a long time (even today), this appealed to many people on a philosophical level since it implied that people have the ability to better themselves through their efforts. Parents who train their bodies or minds will bestow these characteristics on their children and given them a head start over other children whose parent did not do so; parents who read books will have children who are smarter than those who do not, etc. -Unfortunately, absolutely no evidence exists that Lamarck was correct in his mechanism of evolution, even though many people today – non-Biologists – tend to think of evolution in this manner. 3 - Even though he was wrong, Lamarck deserves much credit since he was the first biologist to both believe in evolution and the adaptation of animals to the local environment. - Charles Darwin / Alfred Wallace - Three main persons were very important in shaping the thinking of Charles Darwin and Alfred Wallace who – independently from each other, and around the same time, suggested their mechanism through which evolution could have occurred – natural selection (Charles Darwin published his famous book, “On the Origin of Species” in 1859. (1) Erasmus Darwin, grandfather of Charles Darwin already discussed the mechanism of Natural Selection with the “lunatic society” early in the 19th century. (2) The second person shaping the thought of Charles Darwin was Charles Lyell, who proposed the idea of Uniformitarianism (an opposite view to the view of Catastrophism held by Cuvier, as mentioned above) in which he suggested that the same processes occurring in the world today, also acted on the world throughout history. Geological processes are extremely slow, and geological structures are formed only very gradually. Therefore, the earth had to be very, very old. This provided the huge amount of time that Darwin’s theory required for evolution to have taken place (the biblical idea of the Earth being only 6,000 years old could not have been long enough for complex animals to have evolved through natural selection). (3) Thomas Malthus published “An Essay on the Principle of Population”. In this he suggested that food production by humans increased at a linear rate, while the human population increased at an exponential rate. The human population must have (and may still) outstrip the ability of agriculture to provide food for everybody. This means that many (of even most) people must die through famine, war and pestilence, and that these factors curb the human population, and prevent overpopulation. This lead Darwin to ponder the possibility that some individuals may be better at surviving than others. - Darwin and Wallace essentially suggested that animals change over time to become adapted to their environment. They also believed that organisms are all related through common descent. (see later). - Today The Talk Origins Archive http://www.talkorigins.org Copyright © 1998-2007 Talk.Origins Archive - There is still debate on whether or not evolution is true. Creationism (sometimes misleadingly referred to as “Scientific Creationism” (the Biblical idea that the Earth was formed over 6 days, 6,000 years ago) is still adhered to as a religious principle, but it is not widely accepted as a scientific fact, since it relies on belief and faith, whereas science relies on testing of hypothesis, and confirming or refuting through experimentation or observation. - Attempts are made to fuse the ideas of Scientific Evolution and Religious Creationism by means of modern philosophies as Intelligent Design, which state that Evolution did occur, but it occurred by an Intelligent Designer (God) directly guiding it in a specific direction. - New Darwinism - While details on the mechanism of evolution are still being considered, debated and researched, few biologists doubt that Evolution occurred, and occurred through Natural Selection. The theory of Natural Selection as proposed by Darwin has, however, become altered and updated as new knowledge and new technologies became available. In Darwin’s time, for example, no knowledge existed of genetics. Nobody knew about genes, how they work, or are inherited. The rapid advances in the field of molecular genetics over recent decades provided a powerful new tool in the field of Evolutionary Biology and was incorporated into theories on how evolution occurred, and is commonly referred to as “Neo-Darwinism” 4 Section 2: The Origin of the Earth, and of Life. 2.1) The Primitive Earth -There is evidence that the universe started about 15 billion years ago with the “Big bang”. Our solar system gradually formed over the last 10 billion years - The primitive earth was fully formed by ± 4.5 billion years ago - At that stage, the early earth was molten, and slowly cooled into several layers. Heavier elements accumulated at the bottom (i.e. iron, nickel) to form a denser, molten, liquid core. A less dense layer of silica minerals became the semi liquid mantle floating on top of the core. Slight cooling of the outer layer of this mantle, combined with ongoing volcanic eruptions produced the thin crust, a layer about 100km thick, on which we live and go about our lives. (The deepest mines today penetrate several kilometers (over 4km) into the Earth’s crust. As one goes deeper into the earth’s crust, however, the temperatures rise sharply. - The gravitational attraction exerted by the earth was big enough to retain some of the gases produced by degassing of the earth so that an atmosphere formed (unlike smaller planets in our solar system without an atmosphere). The lightest elements escaped into space while the earth was still being formed (e.g. Hydrogen, Helium, Oxygen, Argon). Heavier elements were retained in our atmosphere (e.g. gases like Carbon Dioxide, Nitrogen, Suphur Dioxide, and other molecules like water vapour, Ammonia and Silicon dioxide). - As the earth slowly cooled, water vapour condensed into dense rain which created the oceans, although high temperatures and continued bombardment of the earth by meteorites prevented them from being the stable water bodies we think of today. - At first, the earth’s atmosphere contained no free Oxygen and would as a result not have possessed an outer layer of Ozone (which absorbs harmful radiation coming from surrounding space, especially ultraviolet radiation from our sun). During the Archaean Period (± 3,8 billion years ago) a slight ozone layer formed. - Gradually the earth cooled to temperatures more amenable to supporting life. 2.2) The Origin of Life 2.2.1 Conditions favorable for the origin of life We can speculate that – for life to have evolved on earth – four conditions must have occurred. 1) Oxygen must have been absent, or occurred only in very minute quantities. Oxygen breaks down simple chemicals through oxidation (think, for example, of the necessity for Oxygen to break down chemical energy during our own respiration). When a fire burns, it uses oxygen. This condition was probably fulfilled with most of the oxygen having escaped from the primitive earth, and the remainder having been tied up as Oxides. 2) An external source, or sources, of energy must have existed to power the process. Fortunately, the primitive earth had no lack of these. a) Solar radiation b) Heat (volcanoes, meteorites impacting the earth) c) Lightning d) Radioactivity 3) Chemical molecules must have existed for the above energy sources to act upon (see later). 4) A huge amount of time must have been available for all of these to work together to produce the first life forms. The earth is generally believed to be about 4.5 billion years old. The earliest fossil evidence we have for life is about 3.8 billion years old. This means that several hundred millions years may have elapsed between the formation of the earth and the first tangible signs of life. 5 How could this life have begun? 2.2.2 Stages during the origin of life Monomers evolved - Oparin (1938): Suggested that simple organic compounds (e.g. nucleic acids, amino acids) could have spontaneously been created by the earth's atmosphere, under the influence of strong energy sources (see point 2 above) - Stanley Miller (1953) demonstrated that this could be possible by performing an experiment in which he combined the gases which would have existed in the earth's atmosphere (water vapour, Hydrogen, Methane, Ammonia), sealed them in a chamber, and added electricity (to simulate lightning). A collection of organic molecules was formed. - As more information became available in later years on the probable composition of the early earth’s atmosphere, this experiment was repeated many times, and it was demonstrated that many the basic building blocks important in modern, living organisms could be created in the lab. - Nucleotide bases of RNA and DNA - All 20 amino acids - Lipid molecules - Certain sugars - Adenosine triphosphate (the molecule responsible for energy transfer in modern organisms) -On the primitive earth, there would have been a gradual accumulation, over a very long period time, of these organic molecules in the shallow oceans, to produce an “organic soup”, or a “primeval soup”. Polymers evolve -The next step in the evolution of life entailed these simple compounds combining into longer chains – forming polymers. - Sidney Fox showed experimentally that amino acids polymerise abiotically when exposed to dry heat (or, put in other words, amino acids spontaneously produce long strings of amino acids – polypeptides). Subsequent experiments have confirmed that simple organic compounds can combine into polymers on hot rock or hot clay surfaces (especially if these surfaces contain minerals like Iron or Zinc, which may act as catalysts). - This could have happened on the early earth as well. Amino acids, plentiful in the early ocean, would have collected in shallow puddles where they could have been cooked on clay surfaces by the sun to produce simple polypeptides (proteinoids – small polypeptides with some catalytic properties: in other words, polypeptides which have the ability to function as primitive enzymes). This is not implausible if one considers that the important thing which gives an enzyme its highly specific function is the shape (which is, in turn), simply a reflection of the order in which its constituent amino acids are linked in a long chain. Coacervates form - Returning these proteinoids to water, they formed microspheres (protein balls with some properties of a cell). Some of them can divide into two (similar to binary fission). Some of them have internal environments different from that of the surrounding environment (a primitive type of “homeostasis” being maintained). Some of them can absorb molecules from the surrounding environment. - If lipids were to become available to these microspheres, they became incorporated into it as a simple membrane. - Oparin (previously mentioned) showed that certain dense concentrations of macromolecules can, under appropriate conditions of temperature, ionic composition and pH give rise to units called coacervate droplets, a special type of microsphere. - Coacervates can absorb and incorporate molecules from the surrounding environment. These "protocells" will have absorbed “nutrients” from the surrounding environment, and they have been 6 shown to have a limited catalytic ability to break down such nutrients. (e.g. when adding Glucose phosphate to the environment, the coacervates can absorb it, and convert it to Maltose). - Coacervates have the ability – by absorbing molecules from around them – to grow. - Eventually, a semipermeable membrane may form around the coacervates if lipids are absorbed from the surrounding environment. - They also have the ability to reproduce (to produce copies of themselves. Small buds can pinch off from a larger coacervate to produce a smaller coacervate. This type of reproduction is seen in some bacteria). They cannot do so spontaneously, however. This has to be induced experimentally. This means that they can not be called alive. Primitive cells develop - Somehow, a jump must have occurred from coacervates to the first true cell. Possibly this occurred due to the ability of coacervates to absorb molecules from the external environment. If self-replicating, or catalytic RNA strands were absorbed by a coacervate, the coacervate would have turned into a primitive prokaryotic “cell”. - This is plausible, since RNA nucleotides, as well as longer RNA-strands, would have existed in the surrounding oceans. Furthermore, RNA does often have self-replicating capabilities. We call such self- replicating strands of RNA ribozymes (from a combination of RNA+enzyme – refers to RNA which has enzymatic abilities). - The RNA strand, once incorporated into the coacervate, would have given the coacervate the ability to reproduce, while the coacervate would have protected the RNA strand from external damage or degradation, giving it more stability. - With nucleic acids having been incorporated into a “proto-cell”, biological information about an entity could then have been stored. - With biological information being stored, some of these structures could possibly replicate themselves, and transfer their own phenotypes (physical characteristics) to their “offspring. - Some of them would have been better at doing this than others. They could produce more of their own kind. They had a Selective advantage, and could out-compete others which were not as successful. The origin of complex cells - As described above, the first protocell possibly developed from a coacervate that enclosed a self- replicating strand of RNA. - We can not be sure when life originated on earth, but the oldest definite, unambiguous fossils suggest that complex cells have existed by 3.5 billion years ago. - The first cells would probably have been prokaryotic heterotrophs (organisms unable to synthesise their own organic compounds). These cells would have had to absorb these compounds from the surrounding environment). They would probably have used up the available sources of free nutrients, leading to competition between them. - Later, chemosynthetic and photosynthetic autotrophs would have evolved. They obtained energy from oxidising inorganic compounds like hydrogen sulphide, obtaining energy from the sun, and releasing sulphur into the atmosphere. - The cyanobacteria were the first bacteria to evolve “modern” photosynthesis (as we know it), using not hydrogen sulphide for photosynthesis (and releasing sulphur into the atmosphere), but water instead (as modern plants do), and releasing oxygen into the atmosphere. Traces of chlorophyll, found in rocks, suggest that this could have happened between 3 and 3.5bya (Billion years ago). - With the evolution of autotrophs, using energy from the sun to produce organic compounds, it was inevitable that organisms would evolve that could feed on them, and feed on the energy-rich compounds produced by them (between 3.5 and 2.5 bya). Because oxygen still occurred only in very low levels in the atmosphere, these Heterotrophs had to use alternative sources to reduce compounds and release energy (unlike most heterotrophs today which use oxygen). These Heterotrophs include methanogens (reduced carbon dioxide to methane) and sulphate reducers (reduced sulphate to hydrogen sulphide) 7 - By 2.5-2 bya, Autotrophs were producing increasing quantities of oxygen, and it eventually become an increasingly important component of the atmosphere. This can be observed in the geological record from 2.5 to 1.5 bya, when iron oxides were observed in rocks in increasing quantities (before then, free iron dissolved in water since there was no oxygen to cause iron to precipitate). - Increasing levels of Oxygen led to several important changes, one of which was the formation of a protective layer of Ozone surrounding the atmosphere, reducing the levels of ultraviolet radiation from the sun. This allowed animals to live closer to the surface of the oceans (no organisms were living on land, by this stage). Secondly, organisms using Oxygen for reduction in their metabolisms were much more efficient than Methanogens or Sulphate reducers, and would have gradually started to dominate the earth. - These cells didn't have a cell nucleus; possibly only a self-replicating RNA strand. They were therefore still prokaryotes. Eukaryotes developed - Cells possible developed a nucleus by invagination of the cell membrane to enclose the genetic component of the cells. This produced the first Eukaryotes. The fossil record suggests that this may have occurred at least around 1.5 bya, and possibly much earlier. - Organelles then developed in these Eukaryotes according to the Endosymbiont hypothesis - The Endosymbiont hypothesis (Schwartz and Dayhoff 1972) states that chloroplasts were once free living, photosynthetic autotrophic prokaryotes, and mitochondria were once free living, aerobic heterotrophic prokayotes that were ingested but not digested by a nucleated cell and a complex symbiosis developed between the cell and its food. 2.3) The geological time scale Most of the rocks exposed at the surface of the Earth are sedimentary--formed from particles of older rocks that have been broken apart and transported by water or wind. The gravel, sand, and mud settle to the bottom in rivers, lakes, and oceans. These sedimentary particles may bury living or dead animals and plants on the lake or sea bottom. With time, the sedimentary particles become cemented by lime, silica or iron and they turn into rock again. Gravel becomes a rock called conglomerate, sand becomes sandstone, mud becomes mudstone or shale, and the animal skeletons and plant pieces can become mineralized to form fossils. In the late eighteenth and early nineteenth centuries, the English geologist and engineer William Smith and the French paleontologists Georges Cuvier and Alexandre Brongniart discovered that rocks of the same age may contain the same fossils even when the rocks are separated by long distances. They published the first geologic maps of large areas on which rocks containing similar fossils were shown. By careful observation of the rocks and their fossils, these men and other geologists were, for example, able to recognize rocks of the same age on opposite sides of the English Channel. Geologists didn’t, at first, know how old the different layers were in absolute terms. They knew the relative order in which they occurred, and they knew that they had to have been formed over a huge amount to time. It is only with the advent of Radiometric dating, that we were able to assign specific ages to these individual rocks. A time scale became established during the 1700s and 1800 based upon observation of fossils in the rock layers in England and Europe. On this time scale, time is divided into units such as Eras and Periods characterized by specific animals that lived at the time. These time units have been named based upon the remains / fossils found in sedimentary rocks, and for this reason, the names of Eras and the corresponding rocks tend to end in –zoic (e.g. Mesozoic). Prefixes such as Palaeo- means “old”, Meso- means “middle” and Ceno- means “Recent”. Proterozoic (Precambrian), for example will refer to “First Animals” (Protero- + -zoic). From this it becomes easy to remember the relation of these time periods to each other. 8 9 2.4 The development of complex life: Little fossil evidence is available of life that occurred before the Cambrian because, many fossils have been destroyed by volcanic activity since they were formed. Furthermore, since the rocks that were formed during the pre-Cambrian time are so extremely old, they occur, for the most part, only in extremely deep layers of the earth’s crust and fossils consist of imprints of generally primitive soft bodied forms without hard components (shells or skeletons). Since those are the parts of animals most likely to fossilise, we are left with a relatively unrepresentative sample such as the Ediacarian fauna of what organisms lived during most of the earth’s existence. Even so, we know that multicellular life was present around 1 bya, and, towards the end of the Proteozoic Eon (570 my ago), and the start of the Phanerozoic, animals capable of producing hard, calcareous shells appeared. - The Palaeozoic Era began roughly 570 million years ago, and is divided into 6 periods. The first of these, the Cambrian, is characterised by a huge “explosion” of marine invertebrates. This explosion of life continued into the Ordovician, when some fossilised spores of terrestrial plants tentatively seem to suggest that life had moved onto land (in the form of algae). - Only during the Silurian do we get the first definite signs of plants establishing themselves on land (macrofossils); mostly mosses. Since this provided food and shelter, it allowed the first air-breathing animals to follow them onto land. By the end of the Silurian, the terrestrial fossil record shows evidence of fungus-eating Arthropods, and predatory scorpions and millipedes. In the sea and freshwater, many types of fish have developed. - During the Devonian Period, both the plant diversity and animal diversity increased. Primitive amphibians appeared during the late Devonian. - The Carboniferous Period is named for the huge swampy forests which covered much of the world, filled with ferns and gymnosperms. In the Carboniferous the first Amniotes appeared – the first Reptiles, (amniotes are vertebrates which lay eggs with shells; such as reptiles, birds and mammals…although, of course, Mammals and Birds had not yet appeared). Amphibians were still the dominant terrestrial carnivores at this stage. - Amphibians were still plentiful and diverse during the Permian Period, but the reptiles diversified and became the dominant terrestrial carnivores and herbivores. During the Permian, the therapsids (mammal-like reptiles) appeared which eventually gave rise to the mammals and the ancestors of the dinosaurs, birds, tortoises, lizards and snakes evolved. - For reasons which are not known, 90% of all marine species, and 70% of the terrestrial vertebrate genera became extinct at the end of the Permian, and within a very short period of time. This mass- extinction ended the Palaeozoic, and gave rise to the Mesozoic Era. Possible explanations include climatic changes, associated with decreased oxygen levels, and increased levels of Carbon dioxide in the atmosphere. - The Mesozoic Era is generally referred to as the “Age of Reptiles”. During the three Periods of the Mesozoic, the Triassic, the Jurassic and the Cretaceous, reptiles showed huge differentiation. - During the Triassic Period, the two dominant groups of reptiles on land were the therapsids (eventually to give rise to mammals) and the thecodonts (eventually to give rise to birds, crocodiles and dinosaurs). Of importance is the origin of mammals at the end of this period, at first consisting of small, nocturnal insectivorous mammals, but diversifying in the Jurassic and Cretaceous and thereafter to give rise to many extinct and the present day marsupial and placental Mammals. - The dominant vertebrates of the Jurassic and Cretaceous periods were represented by a diverse group of reptiles descendant from thecodonts known as the Dinosaurs. They dominated the earth until they finally went extinct at the end of the Cretaceous (65my ago). - During the Jurassic Period and the Cretaceous Period many lizards and crocodile species appeared, as well as the earliest birds. Archaeopteryx is the earliest known bird from the fossil record (~150mya) and evolved from reptiles during the Jurassic in southern Germany. It combined many of the features of reptiles with those of birds (e.g. possessing teeth and a long, bony tail). Flowering plants appear in the fossil record toward the end of the Cretaceous Period. 10.- The Cenozoic Era saw the diversification of flowering plants, mammals, insects and birds. Of great importance is the appearance of human ancestors appearing during the late Miocene Epoch (5my ago) and early Pliocene. Much research on this topic is still continuing, and many aspects of this evolution are still being debated, sometimes very vigorously. A complete discussion of this, sometimes contentious, topic falls outside the scope of this course, but some generalities are given here. - The genus Australopithecus appeared around 4mya, fossils of Australopithecis anamensis being known from East Africa. This species gave rise to several other species, including Australopithecus africanus, first discovered in South Africa. - The genus Australopithecus gave rise to the genus Homo about 2.3mya, with several fossil of Homo habilis (handy man) being known. - Homo erectus probably originated in Africa around 1.7mya, and spread to Europe and Asia. - Archaic Homo sapiens (sometimes placed in a separate species, Homo heidelbergensis) descended from Homo erectus and lived in Africa, Europe and Asia between 800,000 and 100,000 years ago. They gave rise to modern Homo sapiens around 300,000 years ago in the Middle East and Africa. - They then spread to the rest of the world, and here we are today. 2.5 “OUR WANDERING CONTINENTS” Not only did life originate and diversified on earth over the past 3,5 by, but the anorganic earth itself has also gradually changed over time. Powerful changes take place in the mantle and crust of the earth associated with continental drift, i.e. the movement of tectonic plates. The splitting up of large supercontinents to form the geographically isolated present day ones had a big influence on the biogeography and evolution of plants and animals. Before 1915, it was believed that the earth’s crust was immobile, and that the continents had always looked the way they do today. Many anomalies to this belief were evident, however, such as the separation of related terrestrial animals species on different continents, with no explanation of how they managed to cross oceans. Other anomalies also became evident as people obtained more information. Coal seams were discovered inside the Arctic circle, and evidence of earlier plant life was found in Antarctica. This could not be explained by the present position of Antarctica at the cold South Pole. Furthermore, it had always struck people how complementary in shape the East coast of South America was to the West Coast of Africa. The rock formations between these coastlines were also found to closely match up. How was this to be explained? Following the work of geologists such as Wegener and Alex du Toit, we know today that the continents are, in fact, not stable. They are actually moving, relative to each other, a process that has been ongoing since the primitive earth nearly 4 bya. The interior centre of the earth consists of a molten core of molten magma and metal. This core is heated by, amongst others, radioactivity and pressure. This core spins continually and is responsible for the earth’s magnetic field. Without this magnetic field, compasses would not work and since the magnetic field also protects us against many cosmic rays from the sun, it is essential for the survival of life on earth. Around this core of molten magma we find a semi-liquid layer of cooler magma referred to as the mantle, which flows by means of convection and gravitational effects, at extremely slow rates. The crust is of the earth is fragmented into a series of large plates called tectonic plates. There are about twelve large plates, and roughly 20 smaller ones, ‘floating’ on the outer layer of the mantle and forming the six continents, some islands and the seafloor. The crust averages about 40km in thickness, but it varies from place to place. It is, for example, much thinner on the floor of the ocean (maybe as little as 5 - 10km), and is thicker under the continents (as much as 65 km under very high mountains). As the semi-liquid mantle moves, the continental crust (and thus, the continents), are carried along. As these plates move, some plates are torn apart, or shoved together, resulting in earthquakes. You may have heard of the San Andreas fault in California. Here, two tectonic plates form a boundary, and these two 11 plates grind against each other. This sometimes leads to earthquakes. In places the molten magma breaks through weaknesses in the overlying crust resulting in volcanic eruptions. During the Palaeozoic Era (Carboniferous Period) the landmasses drifted together to form a large landmass in the Permian Period called Pangaea (fig. 1). (Pan- means “all” while Gaea means “earth”. All the continents of the world were joined together in one single super-continent. Fig. 1) A reconstruction of what Pangaea looked like during the Permian. All the continents we know today were united in one, big, “supercontinent”. At the start of the Jurassic, about 200 million years ago, Pangaea began to break up (a.) to form two landmasses, Laurasia to the North (today North America, Greenland, Europe, most of Asia) and Gondwanaland to the South (today Antarctica, South America, Africa, Arabia, Madagascar, India, Australia and New Zealand). (b.) As the Jurassic wore on, India broke free from present day Antarctica and drifted northwards (fig. 2). (c.) Towards the end of the Cretaceous the continents composing Gondwanaland gradually broke free from one another, while (d.) India steadily moved north as an island finally making contact with Asia about 35 million years ago. India crashed into Asia, and as it pushed into Asia, it created the mountains known today as the Himalayas. This process is still continuing today. Fig. 2) Laurasia and Gondwana separated, India drifting northwards 12 (e.) Laurasia finally broke up about 20 million years ago during the Miocene, and North America, Greenland and Eurasia become separated, (f.) and Australia broke free from Antarctica (fig. 3 & 4). Fig. 3) North America and Greenland separating from Eurasia, Australia free from Antarctica. Fig. 4 Continents are they are positioned today. North & South America are connected, India is a part of Asia, and Arabia has split off from Africa to form part of Asia The continents are, of course continuing to move, and with sophisticated technology it is actually possible to measure the rate at which continents do so. It is predicted that Africa will eventually split into two along the fault line that is known as the Rift valley in East Africa, and most of Africa will crash into Europe; the Mediterranean Sea will disappear and a mountain range will form across Europe towards India. Australia will crash into Asia or perhaps slide along the eastern edge of Asia, pushing the islands of Japan ahead of it until it eventually crashes into Siberia. The Atlantic ocean will increase in size as North America drifts West until the Atlantic is bigger than the Pacific Ocean. Antarctica will move North into the Indian Ocean. All of this will, of course, take many millions of years. America is drifting West, while Australia is drifting North, at a rate of no more than 4 cm per year. In one person’s lifetime, therefore, the continents will move no more than one or two metres. The influence of continental drift on the distribution of animal and plant species was moderated by the oceans rising and dropping during the ages. During cyclical (in other words, they occur at regular intervals) cold periods known as ice ages, much of the earth’s water is caught up as ice, spread across the globe in the form of glaciers. With so much water in ice-form, the ocean levels are much lower than they are during non-Ice ages, as we are living in today. The last Ice-age ended about 10,000 years ago. When the last ice-age was still present, much of Northern Europe and North America was covered with ice. Because the sea level was much lower than today, people could walk between France and England on dry land, or from Alaska to Russia. North- and South America, now 13 connected through Panama, were previously separate. Africa has been in contact with Europe in the past across the Strait of Gibraltar a number of times. The changes in sea level made it possible for organisms to move from one continent to another at different time during the ages. In other cases organisms were isolated for long periods of time, e.g. the marsupials of Australia. The moving continents also resulted in climatic changes associated with latitudinal changes. Continents, once tropical became deserts. Other continents, once close to the Arctic Circle moved across the equator, with resulting temperature changes. As the climate changed, organisms could not adapt, and many become extinct, leaving space in which other organisms could develop and radiate. 14 Section 3: The Mechanism of Evolution: 3.1 Evolution and Genetics 3.1.1 Introduction When evolution happen, it is the population that change and not only a single individual. Evolution affects both the phenotype (what the organisms of the population look like) and the genes that control the phenotype (the genotype) of the members of the population. All the genes of a population together is called the gene pool. In a previous section on genetics you have studied how genes normally interact to produce the phenotype. In this section we look at the mechanisms how the composition of the gene pool can change. The relative abundance of different genes in the gene pool will not change during reproduction but only by the mechanisms of evolution. In this section we will see that normally, the genes of a population remain the same generation after generation unless evolution takes place. We will then look at the mathematical effect of evolution on the genes in the gene pool and lastly look at the factors driving the mechanism of evolution. 3.1.2 Mendelian Inheritance Gregor Mendel was an Austrian monk who revolutionized the study of Genetics in the early 1860s (although nobody realized this until the beginning of the 1900s). At that time, most breeders of plants and animals only knew that both parents contribute equally to the appearance of offspring, and that offspring fall someplace in between either parent in appearance. They mistakenly believed that this worked in a way similar to the mixing of paint. Mixing red and white paint results in pink paint. Mixing genes for pink flowers and white flowers in the parents will result in the offspring having pink flowers. This belief implied that the original characters/attributes of the parents have been changed permanently. In the same way, one cannot reconstitute red paint and white paint once they have been mixed. This is referred to as a Blending Theory of Inheritance (i.e. parental characters are blended together in producing offspring). The contribution that Gregor Mendel made was to show that the original characters controlling the phenotype are not destroyed during breeding. They remain intact, and can recur in later generations. When a red flower and a white flower produces pink offspring, that pink flower still has the ability to produce pure red or white flowers.This is referred to as the Particulate Theory of Inheritance (i.e. the “Units of Inheritance” which determine offspring appearance (which we now refer to as Genes), remain distinct units). Remember from the previous section on genetics that because you have two Homologous Chromosomes of each type of chromosome in each cell in the body, it means that you also have two genes occurring on these two chromosomes at corresponding positions (loci) that control the same characteristic in the phenotype. Originally, when you were a zygote, one gene came from your Father, and one from your Mother. Because the genes have different origins, they may or may not be identical. - A character like “eye colour” for example, has a large number of possible “gene states” in which it can be found in people; e.g. blue, brown, green. These different forms of the same gene are called Alleles 15 - “Blue eyes”, “Green eyes”, “Brown eyes” are thus Alleles for eye-colour. “Short stem” or “Long Stem” are possible Alleles for Plant length etc. Those are the possible genes floating around in the genepool of the population. You can only have two of them at any one time, however, because you only have 2 copies of each chromosome. Like you heard in the section on genetics, if you have two genes that are the same, you are called Homozygous for that Character. If they are not the same, you are Heterozygous for that character. Note as well that it is possible to be Homozygous for one gene (e.g. eye colour) but Heterozygous for a different gene (e.g. hair colour). What happens during Meiosis? Homologous Chromosomes separate! What does this mean? It means that one copy of each gene goes towards one sperm cell / egg cell, while another copy goes to another sperm cell / egg cell. Example 1 A male has the gene for “Blue eyes” on one copy of Chromosome 1, while he has the gene for “Brown eyes” on its’ Homologous Chromosome (thus being Heterozygotic for eye colour). Meiosis occurs. After Meiosis 1, he will have two sex cells, one of which will contain the gene for “Blue eyes” while the other contains the gene for “Brown eyes”. After Meiosis 2, in the male, you will have two sperm cells with the gene for “Blue eyes” and two sperm cells that contain the gene for “Brown eyes”. The individual will therefore produce sperms with a 50:50 ratio for Blue-eyes : Brown-eyes. In other words – after fertilization, he will have a 50:50 chance of producing offspring containing either gene. During fertilization, and the creation of a zygote, it is completely random whether the sperm that is involved in fertilization is one containing the “Blue-eyes” gene, or one containing the “Brown-eyes” gene. Fertilization, of course, requires an egg as well. Imagine the female in our example is Homozygous for this attribute. She received a “Brown-eye” gene from each of her parents, meaning that each of her Chromosome 1’s has a “Brown-eye” gene. Following Meiosis 2, all her egg cells will therefore carry the “Brown-eye” gene, since there is no way for any of her egg cells to carry anything else. 100% of all of her offspring will therefore receive a “Brown-eye” gene from her. While the father therefore has a 50:50 possible ratio for Blue eyed vs. Brown eyed offspring, the mother has a 100% chance of producing Brown-eyed offspring. What will the offspring look like? - Naturally, there is no way in the world that you can predict which gametes, out of all the millions produced by you, will be the exact one involved in producing a zygote. You can only determine the likelihood / probability of a certain outcome. In this case, (because each parent contributes an equal amount to the next generation), the offspring will have the following possible appearances. 50 % will be Heterozygous, with one copy each of “Brown-eyes” and “Blue-eyes” respectively, on the two homologous chromosomes. 16 50% will be Homozygous, with both chromosomes in the Homologous pair carrying the gene for “Brown-eyes”. - As you know from the section on genetics, it is custom not to write out lengthy names for Alleles. One usually refers to them with letters; the dominant one is referred to with a capital and the recessive with lower case letters. - “Brown-eyes” Allele can therefore be written as B and the “Blue-eyes” Allele as b. - In the above example, all the Offspring will have Brown eyes because of complete dominance of the B allele over the b allele. The chances of the Phenotype is therefore “100% Brown eyes”. The genotype, however, can be determined using a Punnet diagram and in this example would be is written as - 50 BB : 50 Bb. This is usually simplified to 1 BB : 1 Bb. - In simple terms this means that, while we have no way of knowing for certain which genes will be transferred to the offspring, we know that 1) all of them will have brown eyes 2) on average, we would expect half of any children to be Heterozygous and half to be Homozygous. 3.1.3 Hardy-Weinberg Equilibrium How can we observe that evolution is taking place? - We can do that by looking at allele frequencies in the gene pool, and how allele frequencies change over time, from one generation to the next. - If allele frequencies change over generations, this will change the phenotypes in the population. Small changes in the allele frequencies accumulating over a few generations we refer to this as Microevolution (e.g. Industrial Melanism). - Microevolution can accumulate and eventually result in large changes in the phenotype (i.e. Microevolution can lead to Macroevolution – large phenotypic changes leading to completely different types of animals). What do we actually mean by Allele Frequencies? Example 2: Imagine a population has 100 diploid rabbit individuals. Each of these therefore has two copies of each gene – Let us consider only one gene at a time; fur colour That means that there are 200 alleles in the population which control fur colour Let’s imagine further that there are only two alleles for fur colour: black and grey This means that the 200 genes in the population that control fur colour, all control only for two colours…either black fur or grey fur. Imagine further that in this entire population, ¼ of the individuals are Homozygous for Black; the dominant allele (i.e. BB) ½ are heterozygous (i.e. Bb) while ¼ are homozygous for the recessive allele (i.e. grey, bb). In other words, in the population of 100 individuals, 25 are BB, 50 are Bb and 25 are bb. We now determine allele frequencies as follows: Number of B alleles in the population: 17 Number of B alleles Number of b alleles BB 2B x 25 = 50 BB 0b x 25 0 Bb 1B x 50 = 50 Bb 1b x 50 50 bb 0B x 25 =0 bb 2b x 25 50 Total B 100 Total b 100 We can see that there are indeed 200 alleles. What is the frequency of the B and b alleles? Number of B alleles Number of b alleles = Total # of alleles = Total # of alleles = 100 = 100 200 200 = 0.5 = 0.5 So, 0.5 of all the alleles in the population available for fur colour are B and 0.5 are b. Let’s assume for the moment that there is no selection, and that all of them have an equal chance of being transferred to the next generation. What will the next generation look like? Draw up a Punnett diagram: (This Punnett Diagram differs from the previous Punnett Squares in that in this case we do not calculate the expected Phenotypic/genotypic ratio for the next generation for an individual crossing, but for the entire population). O.5 B 0.5 b 0.5 B 0.25 BB 0.25 Bb 0.5 b 0.25 Bb 0.25 bb This means that the next generation of the population is expected to look like this: 25 % of all individuals will be BB, 50% (25%+25%) will be Bb, 25% will be bb. What do we conclude from this? - Sexual Reproduction alone cannot change allele frequencies in a population - Dominance does not change allele frequencies. Example 3 Let’s look at another, more complex example: Imagine a population, still with 100 individuals But this time: 36 are BB, 48 are Bb and 16 are bb. 18 Calculate the allele frequencies, as well as the expected gene frequencies for the next generation: Step 1: Determine the Allele frequencies: We know that there are still 200 alleles of either B or b in the population. Number of B alleles Number of b alleles BB 2B x 36 = 72 BB 0b x 36 0 Bb 1B x 48 = 48 Bb 1b x 48 48 bb 0B x 16 =0 bb 2b x 16 32 Total B 120 Total b 80 Frequency of Allele B: 120 / 200 = 0.6 Frequency of Allele b: 80 / 200 = 0.4 Step 2: Draw up a Punnett Square to calculate the expected genotypes for the population for the next generation: O.6 B 0.4 b 0.6 B 0.36 BB 0.24 Bb 0.4 b 0.24 Bb 0.16 bb We therefore expect that the next generation will consist of 36% BB individuals, 48% Bb and 16% ll. So…what does all this tell us? What does it mean? - It is clear that being dominant or recessive in itself does not make an allele more or less likely to be transferred to the next generation. - Sexual reproduction in itself does not change the gene pool of a population. In 1908, a British called Hardy and a German called Weinberg determined, independently of each other, what is today referred to as the Hardy-Weinberg Principle. This states that the Allele frequencies in a population will not change, from one generation to the next, unless evolution has occurred. Two generations of a population are in equilibrium if the gene frequencies in them are the same. Allele frequencies will occur in an equilibrium from one generation to the next, according to the following formula p² + 2pq + q² = 1 (and simple probability theory states that (p + q = 1) p² is the frequency of homozygous, dominant individuals p is the frequency of the dominant allele q² is the frequency of the homozygous, recessive individuals q is the frequency of the recessive allele 2 pq is the frequency of the heterozygous individuals This formula allows us to calculate the expected frequencies of alleles from one generation to the next, as long as the population occurs in Hardy-Weinberg equilibrium. 19 Successive generations of a population will remain in Hardy-Weinberg Equilibrium as long as the following conditions are met: 1) No mutations occur in the population 2) No gene flow occurs (there is no migration into the population, of out of the population) 3) Random mating occurs – all individuals have an equal chance of reproducing, and mate choice is not influenced by genotypes or phenotypes 4) No genetic drift occurs. The population is very large, and no changes in allelic frequencies occur due to chance 5) No selection occurs. All genotypes have an equal chance of surviving As long as these conditions are met, a population will remain in Hardy-Weinberg equilibrium. Obviously, in real populations, these conditions are unlikely to be met. One or more will be violated. If the gene frequencies calculated by the above formula after some time has changed, the Hardy- Weinberg equilibrium does not exist; it means that evolution has occurred in the population. 3.2 Causes of Microevolution: The conditions that have to be met for a population to remain in Hardy-Weinberg Equilibrium have already been introduced. (in other words, conditions for the allele frequencies to remain the same from one generation to the next). This implies that the opposite conditions can cause the population to undergo deviations from Hardy- Weinberg Equilibrium (i.e. cause microevolution) The factors that can cause microevolution are therefore: 1) Mutation 2) Gene Flow 3) Nonrandom mating 4) Genetic Drift 5) Natural Selection Any of these factors can cause evolution: 3.2.1 Mutation: - Mutations create new alleles, and therefore constitute the source of new variation in a population. - Most mutated alleles tend to be harmful to a greater or lesser extent. Some are, however, beneficial. Some mutations are neutral (i.e. they have no apparent effect). - Furthermore, it may be that a mutation is harmful in one environment, but beneficial under eg. another set of climatic conditions. - Once alleles have mutated, Genetic Recombination (independent assortment, crossing over and sexual reproduction) is important in spreading the mutation through the population. This will happen if the new mutated gene has a selective advantage. 3.2.2) Gene flow: - Species are divided into populations. These populations are sometimes in contact with each other when migrating individuals move from one population to another. When such individuals reproduce, genes from one population can be spread to another population (e.g. a mutation created a new allele in one population, and this allele is taken to another population by a migrating animal). 20 - If it happens too often, though, it can reduce the differences occurring between populations (i.e. reduce the chance of speciation occurring...we'll touch on this topic again later). 3.2.3) Non-random mating: - Random mating occurs when individuals mate purely by chance, and their phenotypes do not play a role. This usually does not happen, and phenotype does influence mate choice. Three examples of non-random mating: - Inbreeding (between humans, animals or plants). Individuals mating with closely related individuals. This does not alter Allele Frequencies in the population, but does increase the incidence of homozygous individuals and reduces the incidence of heterozygosity at all loci. Many genetic disorders in humans are recessive, and if heterozygosity is reduced, dormant (hidden) recessive alleles will be expressed with greater frequency. -Assortive mating occurs when individuals tend to mate with individuals with a similar phenotype (e.g. in humans, tall people tend to mate with individuals who are also tall). This causes the population to subdivide into two phenotypic classes, between which gene exchange will be reduced -Sexual selection occurs when males compete for the right to reproduce, and females choose to favour males with a certain phenotype. The elaborate tail of a peacock is where female choice leads to selection The size difference in elephant seals between males and females is an example where female choice does not play a role. 3.2.4 Genetic Drift - Genetic Drift is when allele frequencies in a population change due to chance alone. This factor is probably described best at the hand of the following non-biological analogy: Imagine you have a bottle with 1000 blue marbles and 1000 red marbles. If you draw 200 marbles at random, the likelihood is that you will draw close to 100 of each. Based purely on chance, you may draw 90 of the red marbles and 110 of the blue ones, or 95 blue and 105 red. The exact proportion does not matter, and will fluctuate randomly if you repeat the experiment. The next time you will expect to draw different proportions of red and blue marbles. But, you will always expect to draw almost equal numbers. If, however, you have only 10 blue and 10 red, and you draw a total of 4, it is very possible that all will be either blue or red. You will thus have lost the other. With alleles the situation is similar. If the population is very big, the chances are that in each generation you will have a good representation of all the alleles in the population. By chance, however, it is possible that you can lose rare alleles (if one or more allele is lost, the others become fixed in the population). In a small population, it can even occur that rare alleles become fixed, and more common ones are lost. This process is completely random. Loss of alleles can, of course, be reversed by gene flow from another population, or if a mutation restores the lost allele. Without gene flow or mutation replacing the allele, however, it is lost permanently. Two main types of Genetic drift occurs. a) Bottleneck Effect: If a population suddenly undergoes a very severe population reduction (overhunting, sudden climate change, fire etc.), and then increases afterwards, the alleles that are available are probably very unrepresentative of the original alleles. 21 Cheetahs for example have reasonably high population numbers, but they have very low genetic diversity. It is postulated that they have, at some point in the past, suffered a very severe reduction in numbers. The current population is the result of those few individuals who survived having reproduced. b) Founder Effect: When alleles, or certain combinations of alleles occur at a higher frequency in an isolated population than it does in the rest of the general population. When a few individuals from a large population colonize a new habitat, they very possibly may not have the same allele frequencies as the original population. e.g. In the human population, there is a recessive allele which creates a form of dwarfism (involving only the arms and legs) as well as polydactilism (more than 5 fingers on a hand). In the general population, this occurs in about 1/1000 individuals. In the Amish in Pennsylvania, however, this can occur in as many as 1/14 individuals because the original Amish (by chance) had this recessive allele in a large frequency, and since they tend not to marry outside their own small community, this allele has remained common in the population. 3.2.5) Natural Selection (very important factor) Charles Darwin and Alfred Wallace both, at the same time, but independently, proposed that evolutionary change could be explained if Natural Selection is taken as the mechanism. Natural Selection works as follows: (Preconditions 1-3 lead to consequence 4-5) 1) Organisms have heritable variations: - Members of a population differ from each other in many physical attributes. Often, these attributes are heritable -Darwin never knew about Mendel’s experiments. He never knew how variations came to be, or how they are inherited. - He just knew variations had to occur completely at random. - Today we know how variation arises - genetic mutations create new alleles and genetic recombination creates new combinations of alleles. - We also know that these occur at random. (By this, we mean that mutations do not occur with any purpose or direction or anticipation…if the environment suddenly became colder, you do not find mutations for thicker fur suddenly occurring more regularly). 2) Organisms struggle to exist - In a population, many more individuals are born than can be supported by the environment. -Thomas Malthus, in Darwin’s time, stressed the reproductive potential of humans, and the inability of food production to keep pace with human population growth. - This occurs in animals as well; if a population keeps on increasing, clearly the population numbers will eventually outstrip available resources unless many, or even most of the individuals do not survive to reproduce. Which ones survive? 3) Organisms differ in Fitness - Some of the individuals in a population have – by virtue of their heritable attributes – a greater ability to survive and reproduce than others. -Fitness is the Reproductive success of an individual relative to other individuals in a population - Animals differ in appearance or physiology. They don’t have the same colour, or fur thickness, or running speed, etc. These differences give some individuals a greater or lesser chance of surviving. 22 - Animals that run fast, for example, are more likely to escape predators, and will produce more young since slower animals don’t survive to be able to produce young. - Desert plants or animals that have greater ability to conserve water will be more likely to survive and produce offspring. 4) Inheritance of fitness - These attributes favourable for survival are inherited, which means the offspring will also carry the genes for speed, or water conservation, etc. -Their offspring will also have these attributes, so that an increasing proportion of individuals over succeeding generations also carry these attributes 5) Adaptation -Gradually, a population of animals will become adapted to its environment. - The environment is changing all the time, which means that Natural Selection occurs continuously, though not always in the same direction (the same physical attributes aren’t always selected for). - At the same time, mutations continue to create new diversity, so that there is always a pool of new genetic diversity available. -The result of natural selection is a population increasingly adapted to the local environment. 23 Section 4: Natural Selection Remember that this involves certain individuals being better at surviving, or reproducing, than others by virtue of inherited characteristics that give them some advantage over other individuals in the population. Remember how Natural Selection works? 1) Individuals in a population differ from each other and show variation 2) The environment has limited resources and opportunities for survival 3) Some animals survive and reproduce more successfully. 4) They pass their characteristics on to the next generation so that the characteristics of the population change 5) By interfering with individuals who are poorly adapted to the environment from breeding/surviving, and/or assisting individuals who are well adapted in surviving/reproducing, natural selection will cause the next generation to possess a larger proportion of well-adapted individuals in the population. Gradually, Natural Selection will result in a population that is adapted to the biotic and abiotic environment. The biotic environment is the living component of the environment: organisms which eat you, are eaten by you, compete with you for food or space, etc. The abiotic environment includes the non-living component of the environment, e.g. rainfall or temperature. 4.1 Types of natural Selection - Most physical (phenotypic) characteristics upon which Natural Selection operates results from large numbers of genes, each possessing several different alleles. - Genes interact to produce a certain phenotype, since many physical characteristics are too complex to be controlled by only one or two genes. - In a large population, if one measures a physical attribute (for example size), and plots it on a graph, the frequency distribution will have the appearance of a bell-shaped curve. Three types of Natural Selection occur: 1) Directional Selection When an extreme phenotype is favoured by Natural Selection, and the distribution curve of the population is shifted in that direction This can occur when a population is adapting to the environment (e.g. increased size of the modern horse, drug resistance of bacteria or agricultural pests, Industrial melanism – as discussed earlier). 2) Stabilising Selection When an intermediate phenotype is favoured. Extreme phenotypes are selected against. -When certain aspects of the environment remain very constant, over a long time, then animals are well adapted to the environment, and prevented from deviating too much from the average (e.g. human birth weight). Another example could be bird's wingspan. Birds can possibly have wings that are long, intermediate, or short. If their wings are too short, they can't fly. If their wings are too long, they cannot manoeuvre. Both of these types will be selected against, and only the intermediate types will be selected for. This will stabilise wingspan within a narrow range. 3) Disruptive Selection Two or more extreme phenotypes are favoured over intermediate phenotypes. Two distinct phenotypes are produced. 24 Section 5: Speciation - What is meant by speciation? Speciation is the splitting of one species into two or more, or the transformation of one species into a different species over time. - Speciation is the result of accumulated microevolution (i.e. the changing of allele frequencies as discussed in previous lectures). 5.1 The species concept But what is meant when people speak about a species? Not as obvious as one would think and largely a matter of human opinion. - We generally use the Biological Species Concept to define a species: A species comprises all the members of a population, or a group of populations who: - share a common gene pool - who can interbreed with each other - produce viable, fertile offspring - and are reproductively isolated from all other species. - Homo sapiens: although populations are very widely distributed and show great differences in physical attributes, they share a common gene pool – gene flow between them does occur (...or can potentially occur...) and they can reproduce to produce viable offspring. The human species is therefore a good biological species. - A male Donkey and female Horse can interbreed to produce the Mule, but the mule is infertile. They therefore belong to different species. 5.2) Reproductive Isolating Mechanisms It is clear that to belong to different species, populations/individuals need to be reproductively isolated from each other. In other words: for two species to be separate, no gene flow is allowed between them. This does not necessarily mean individuals have no contact with each other; it only means that they are unable to successfully produce offspring together, even if there is contact. In nature, gene flow is prevented by Reproductive Isolating Mechanisms. - A reproductive isolating mechanism is any Structural, Functional or Behavioural attribute that prevents successful mating between individuals. - We can divide these into Prezygotic and Postzygotic Isolating mechanisms. A zygote, you will recall, is the Diploid product that is formed when an Egg cell and a Sperm cell fuse together - Prezygotic mechanisms – prevent mating from occurring, or prevent successful fertilisation even if mating is attempted. - Postzygotic mechanisms – prevent the offspring of individuals from different species (we call such offspring hybrids) from surviving, or reproducing even if they survive. 25 5.2.1 Prezygotic Mechanisms - Geographical isolation: Populations are separated from each other by means of impassable barriers (e.g. finches in the Galapagos islands; many islands, all of them separated from each by water). - Habitat/Ecological Isolation: Species occur in different areas, and therefore do not come into contact with each other to mate (even if they occur in the same area, they may inhabit different areas inside that area, e.g. different layers in the forest canopy. - Temporal/Seasonal Isolation: Species may occur in the same area, but may be active at different times of day, and not come into contact with each other to be able to breed. Many species also have very specific mating periods/seasons, and if two species have different breeding seasons / times, they cannot reproduce, even if they occur in the same area. - Behavioural Isolation: Many animals have complex mating calls (birds or crickets) or mating dances, or they release chemical attractants into the air (called pheromones) which are recognised only by other members belonging to the same species. - Mechanical Isolation: This can occur when animal or plant genitalia are incompatible, or do not match, e.g. male dragonflies have to grasp females before they can mate, but their grasping "arms" (claspers) can only hold females of their own species. - Gamete isolation: Even if two individuals of different species mate, it is still possible to prevent fertilisation since sperm cells often cannot survive in the reproductive tract of another species, or the sperm cell does not recognise the egg cell, or cannot fuse to it because the egg cell has an incompatible cell membrane (remember that the sperm cell head is covered by an acrosome that carries enzymes needed by the sperm cell to penetrate the egg). 5.2.2 Postzygotic Mechanisms - Zygote mortality: The sperm and egg cells fuse to form a zygote, but the zygote fails to develop further (i.e. dies without developing into a foetus). - Hybrid sterility: The hybrids survive, but are infertile and cannot reproduce, e.g. crossing a female horse and a male donkey produces the mule. Even though a mule may be healthy otherwise, it cannot produce functioning sex cells. - F2 fitness: The Hybrids survive, and are even fertile, but their own offspring in turn (i.e. the F2 generation) are weak / have reduced fitness, and may not be able to compete successfully with other animals for food, shelter or breeding mates. 5.3) Types of Speciation - Allopatric speciation – occurs when a population is geographically isolated from other populations and gene flow stops. - Variations due to mutations, genetic drift and natural selection build up causing first post-zygotic and then pre-zygotic reproductive isolation to occur. e.g. adaptive radiation - Sympatric Speciation – Occurs when a population develops into two or more reproductively isolated groups without prior geographic isolation. 26 e.g. through Disruptive selection, competitive speciation, polyploidy - Parapatric Speciation – One species has a wide geographic distribution, and because of this, not all the populations making up this species live under the same environmental conditions (e.g. some populations live in drier conditions, some populations have to adapt hunting strategies to feed on different prey, etc.). Because of this, selection for different phenotypes occurs in different populations, and populations will slowly diverge from each other, even though they are still in contact with each other, and gene flow still occurs between them. Allopatric speciation is generally accepted to be the most important means of speciation in nature 27 Section 6: Evidence for Macroevolution 6.1 Fossil Evidence Firstly, it may be useful to recap what a fossil is, and how it may be formed: Introduction Fossils are the remains of plants and animals that lived long ago. Fossils range in age from thousands of years to many millions of years. The earliest fossils of multicellular organisms date from around I billion years ago. Evidence has been found that bacteria may have existed more than to 2.5 billion years earlier. The vast majority of animals that die simply disappear when they decompose, and no physical trace remains. About 1 in a million of organisms that die in an area where the conditions are just right for fossilization. It is usually the hard parts of organisms such as bones or shells that fossilize. While it does happen, and has happened, it is much less likely for soft tissues to become fossilised. A fossil can also be an indirect trace of an animal or plant’s existence, such as footprints. They can also, however, include full skeletons of e.g. the Mesozoic dinosaurs. From such a skeleton, or even a small part of a skeleton, it is possible to deduce much about the animal. Not only is it possible to determine what the animal looked like, one can also find out much about the life-style and diet of the animal. How can an animal become fossilised? Process Naturally, the animal first has to die. Sometimes animals are killed by a flash flood that also cover them with sediment. If an animal dies in an aquatic environment it will then sink to the bottom, where it is slowly, during the course of months, covered with sand and silt. This protects the animal (or remains of an animal) from scavengers. A land animal can also undergo the same process by being caught in a mud-slide or during flooding of the overbank environment and covered with mud, or by sinking in a swamp. In this manner a shell, skeleton, or body can become covered under several metres of sand or mud. Gradually the chemicals in the shell/bone undergo changes. It slowly decays, and water passes through the remains. This water may contain dissolved rock minerals (Calcite, Iron, Silica) which slowly permeates the biological remains, and replaces the biological chemicals. Over millions of years, the original shell or bone is completely replaced by minerals. What remains is a rock-like mineralized exact replica of the original animal or plant structure. In rare instances do traces of the original biological material such as DNA become preserved inside fossils. Naturally, it also loses all traces of its original colour during this process. Following many millions of years, this object may lie under several hundred metres of sand / silt that changes into sedimentary rock. The surface of the earth does not remain static, however. Tectonic movement of the earth’s plates, earthquakes, volcanoes and erosion can elevate deeper parts of the earth’s surface. A gradual cycle of erosion will then start wearing away the upper layers of the earth, thereby exposing rock layers which had been hidden for many millions, or hundreds of millions of years. By these means, deeper layers of the earth, along with any fossils occurring in them can be exposed. Examining these fossils, the progress of evolution can be gauged directly. How does this work? - Sediments are deposited continuously, so that layers are formed in the earth. 28 - The deeper any particular layer beneath the surface, the older it is. Therefore, fossils found deeper underground are older than those found closer to the ground. - Very much in evidence is the succession of life forms from simple forms (deep geological layers) to the very complex (closer to the surface). - Fossils in different layers are observed to have changed, but the similarity in form allows us to link them in a historical sequence and place them in their proper geological context. - Put in a different way; if we were to examine rocks, beginning with the youngest rocks, and progressively examining deeper (and hence, older) layers of rock, we will initially see evidence of human civilisation and activities, evidence of recent animals, etc. Progressively examining older (deeper) layers of rock, we will come to a level where no fossils of humans are present. If we continue backwards in time (…examine deeper layers), we will successively come to levels where no fossils of flowering plants are present, no birds, no mammals, no reptiles, no four-footed vertebrates, no land plants, no fishes, no shells, and no animals at all, in that order. - So, not only do fossils provide evidence of animals changing over over immense amounts of time, it is also of interest that we find transitional fossils – fossils which combine the appearance of different animals; reptile-like birds, amphibious fish, amphibians looking like reptiles, and mammal-like reptiles (therapsids). - These intermediate fossils, for example suggests that Amphibians evolved from fish, Reptiles evolved from Amphibians and Mammals and Birds evolved from Reptiles. - The fossil record is sometimes not complete, but in many cases it is very complete, and allows us to trace the evolution of specific animals very precisely. The evolution of the horse (Equus) evolving from Hyracotherium, a small forest dwelling animal the size of a large dog (roughly 35kg) is such an example. - The evolution of the whale has also, for example, been traced with some evidence to 4-legged land-living animals, mesonychians, who gradually started adapting to swimming in shallow seas 50-60 million years ago. -Anatomical evolution of the genus Homo is abundantly documented in the fossil record in East and southern Africa. - Many extinct branches of modern groups of plants and animals are evident. An example is Glossopteris, a tree sized conifer whose fossils are found on all the Gondwanan continents but that has left no living descendants. 6.2 Anatomical Evidence - Homologous structures (homologous = corresponding) are structures whose basic anatomical structure developed once in history in a common ancestor, -e.g. the forelimb of vertebrates, which is used for flying (birds and bats), swimming (whales and seals), running (horses, cats, dogs), climbing (arboreal (tree living) lizards), swinging (monkeys), digging (moles) etc. -Despite dissimilar functions, the forelimbs all have the same bones, arranged in a similar way, as well as the same muscles. - The most plausible explanation for this is that the basic forelimb plan belonged to a common ancestor; the structure was then gradually modified by selective pressures to adapt to specific habitats/behaviours. -Analogous structures (analogous= similarly shaped non- corresponding structures), are structures serving the same function, but without a common ancestral structure. E.g. wings of birds and insects, or flippers of seals and fins of flying fish. -Analogous structures show that over time, unrelated structures of different organisms adapt to have an equal function in the same available habitat. 29 - Vestigial Structures – Reduced anatomical structures that serve no purpose, and that are not functional. They occur, however, in functional form in related species, and they presumably also served a function in the own body at some point in the distant past. - The best explanation for them is that the structures have been selected against until they no longer can function, but they are still useful in related species. Vestiges therefore show that the forms in which they occur are related by evolution to the forms where these organs are still functional. - E.g. humans have a tailbone, but no tail - Whales have very reduced thigh bones and a pelvic girdle, even though they no longer use them, nor are they visible externally. - Many snakes also still have remnants of a pelvic girdle and hind legs, even though they no longer use them. - Embryonic development - During embryonic development, stages resemble the adult condition of more primitive life forms. - All vertebrates including H. sapiens show a post-anal tail and pharyngeal pouches in early stages of development. - The most likely explanation for the presence of these structures in the embryonic development of humans is that fishes are our ancestors. - Similar to apes, we as humans usually lose our tails before we are born. - Pharyngeal gill pouches are homologous with gill slits of fish and become modified into tonsils and the thymus and parathyroid glands in humans. -The middle ear ossicles in mammals developed from elements of the lower jaw and jaw articulation- a development process seen in the embryology of mammals and the therapsid fossil record. 6.3 Biogeographical Evidence / Patterns of distribution / Parallel adaptation - Similarities in widely separated species on different continents of the same genus suggest a common ancestry, while differences between obviously related species in close proximity suggests isolation and independent evolution. - eg. Large catlike species occur all over the world, but they are separated by impenetrable barriers like oceans. e.g. the Jaguar in South America, and the Leopard in Africa and Asia and the Tiger in India. Their similarities suggest a common ancestry, but differences suggest that they have been diverging for millions of years. - Biogeography might suggest that they started diverging genetically when the African and South America continents drifted apart. - Biogeography thus looks at things like plate tectonism to explain how and when these species could have diverged. - Marsupial animals in Australia and placental animals in the rest of the world, which have been geographically isolated for millions of years, have both developed similar ecotypes in similar niches. - Similarly, islands tend to have few, but unique resident species. These species are often similar, yet obviously different from species in other, neighbouring islands (e.g. finches in the Galapagos Island where many species on islands close together appear very similar, but with slight differences as they adapted to specific habitats). 30 6.4 Biochemical Evidence / Molecular record - Almost all living organisms use the same basic biochemical pathways and organic molecules such as respiration and ATP. - DNA and proteins formed during a similar process of protein synthesis form the building blocks of all organisms. Proteins consist of the same 20 different amino acids which are arranged in sequence. - DNA controls the arrangement of amino acids in proteins, and genetic mutations change the sequence of amino acids, and hence, the structure of the protein. - A great many genes are shared by all animals – humans share many genes with very primitive animals, especially developmental genes (i.e. genes responsible for body form before birth, during gestation). - It is possible to compare the degree to which important genes, or gene products (proteins) differ. As would be expected from evolutionary theory, the more closely related animals are, the more similar their genes/proteins. The genes of humans and the genus Pan (chimpanzees and gorillas) corresponds nearly 98%, indicating a common ancestry. 31 BIOLOGY 1 FIRST SEMESTER Ecology and Environmental Health Compiled by: Prof E van Heerden & Mr MR Moshe Adapted by: Mr NM Mogale Lecturer: Mr C Mavimbela SEFAKO MAKGATHO HEALTH SCIENCES UNIVERSITY DEPARTMENT OF BIOLOGY Ecology and Environmental Health Chapter 1: Ecology 1. Introduction Ecology, put simply, is a branch of biology that examines the interactions of organisms with their environments. Ecologist study the patterns of distribution and abundance of organism in nature and how these patterns are maintained in the short run, and how they change during the course of evolution (Arms and Camp, 1987). The word ecology was coined by a German zoologist called Ernst Haeckel in 1866 and is derived from two Greek words namely “oikos” meaning home or house and “–ology” meaning the “study of”. Ecology, is defined by Begon, et al (2006) as; The scientific study of the distribution and abundance of life and the interactions between organisms and their natural environment. The environment of an organism includes its physical properties or factors, which can be described as the sum of local abiotic factors (e.g. sunlight, temperature, water, and soil), chemical factors (e.g. composition of air, soil, and substances dissolved in water) and biotic factors (e.g. other living oragnisms sharing a habitat). The interactions between organisms - as articulated in the definition above - are further expressed by Mader (1998) as determinants of the distribution and abundance of organisms at a particular locale and over the earth’s surface. 2. Levels of Ecological Organization Ecology is sub-divided into a hierarchical system involving the biosphere at the highest level of organization, which is immediately followed by the ecosystem, then community, population, habitat and lastly individual. BIOSPHERE ECOSYSTEM COMMUNITY POPULATION HABITAT INDIVIDUAL Figure 1: Diagrammatic representation of ecological organization 2.1. Individual level Ecology can be studied at a wide range of levels from a small scale to large one (Mader, 1998). At its lowest scale - the individual level - ecologist studies how an individual organism is adapted to its immediate environment. An example in this case would be a newborn ungulate calf which has to learn how walk in a few minutes to enable it to outrun predators. If adaptation does not occur, predation is a likely consequence. Another classical example is the famous peppered moths (Biston betularia) observational study in England around the 19th Century. This at the same time is an expression of evolutionary change by means natural selection. Other example includes as part of behavioural ecology – imprinting and operant conditioning. Read more on peppered moths and their differential adaptation to the environment and the significance of imprinting for adaptation and survival of individual(s) to their environment. 1 2.2. Habitat Level A habitat in this case refers to a place where an organism lives (Arms and Camp, 1987) and reproduces in its environment. Each species occupies a particular position in the community both in the spatial (where it resides) and functional sense (what role it plays). A practical example of a habitat would be the forest floor, ocean edge or stream. Every habitat is characterised by particular ranges of temperatures, humidity, soil, vegetation structure, food type, competitors, predators and other factors that make up the organisms environment (Mader, 1998). As has been mentioned above that not only does an organism occupy a particular habitat, it also has some role to play within that habitat. This functional element brings about the concept of ecological niche of an organism which is defined as the role the organism plays in its community including its habitat and its interactions with other organisms. The niche includes the resources an organism uses to meet its energy and nutrient requirements and survival demands. Also what is important to note is that because a niche of an organism is affected both by abiotic a (climate and habitat) and biotic factors (such as competitors, parasites, predators), ecologists distinguish between the fundamental and realized niches. The former implies the conditions under which an organism can potentially survive and reproduce whilst the latter deals with the actual set of conditions under which the organism exists in nature. 2.3. Population Level A population is defined by Mader (1998) as the existence of all organisms of the same species within a specified area. At this level of study, an emphasis is based on the factors that affect growth and regulation of population size. Ecology as a science not only studies the factors that affect growth and regulation of populations but also the distribution of organisms in terms of where and why they are located in a particular place at a particular time. 2.3.1 Characteristics of Populations At any one point in time, populations have a certain size. Population size in this case is defined as the number of individuals contributing to the population’s gene pool. 2.3.1.1. Factors affecting the size of a population The numerical size of a population at any one time depends upon a balance between natality (births), mortality (deaths), emigration (movement of individuals out of a defined population) and immigration (movement of individuals into a defined population). Imagine a glass of water being your population, any addition of water to the glass will subsequently increase the volume of the water. Factors acting in this way will include natality and immigration while the reverse would include the reduction of water by means of drinking or spillage, which subsequently would reduce the volume of water in the glass. Such factors would include mortality and emigration. Natality Immigration Mortality Emigration Increase population size Decrease population size Population size Figure 2: Diagrammatic representation of factors influencing population size 2 Population size at any one point in time would depend upon a balance between Natality and Immigration which adds individuals to the population and Death and Emigration which remove them. The change in the size of a population can be expressed in this form below: Change in Population size = (Natality + Immigration) – (Mortality + Emigration) Under ideal conditions, populations can grow rapidly to exponential proportions and this phenomenon is called exponential growth (see Figure 3, below). This is most likely if populations increase in numbers without any form of regulation to their growth. To realize this form of growth, populations would require such factors as superabundance of food and space and lack of interference from members of other species. These factors subsequently enhance the biotic potential of a population which is described as maximum rate of population growth. Exponential Growth of a Hypothetical Population 12000 10000 8000 Number of Individuals 6000 4000 2000 0 1 2 3 4 5 6 7 8 9 10 11 Number of Years FIGURE 3: A graph showing exponential population growth Even though exponential growth occurs in nature – and does not necessarily mean that the population is growing at its biotic potential - most of what is apparent is the outcome of the interaction between a population biotic potential and various environmental circumstances which in more ways than one, restrict the actual growth rate. An example of exponential growth in nature occurs as a consequence of species imported, intentionally or accidentally into an area of abundant resources and lack of competitors or natural enemies. Can a population grow exponentially indefinitely? No population can grow exponentially for long since this phenomenon is highly dependant on its carrying capacity. This term denotes the number of individuals of a particular species that can be supported by a particular environment. When a population reaches this size it may stabilize with fluctuations above or below the carrying capacity. The curve describing this phenomenon is called a logistic curve or an S-shaped curve. The most important question to ask in this regard is why a population cannot grow exponentially indefinitely? The answer lies in the biotic potential and the carrying capacity. If so, then which factors acts upon either the biotic potential or the carrying capacity of a population? 2.3.1.2. Factors affecting biotic potential of a population If you recall, under ideal conditions, an explosive population should have such factors as abundance of food, space and lack of interaction with members of other species. But in reality, the situation is different thus. Factors affecting or regulating the growth of any population can be classified into two broad categories namely density- dependant and density independent. Density-dependant factors are such factors, that, in order to be operational; they depend on the density (the number of individuals per unit area) of the population while the latter can take place without the density of the population. 3 Density dependant factors, as shown in Figure 4 below includes such factors as competition for resources for instance food and space, predation and disease. On the other hand, density independent factors would summarily include abiotic factors such as natural disasters. FIGURE 4: Showing the relationship between limiting factors on the size of a population Competition particularly in large populations is u