Biology 2 PDF - Nervous System Basics
Document Details
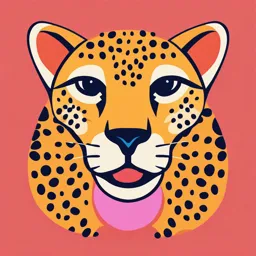
Uploaded by GraciousVignette
The University of Nottingham
Meritxell Canals
Tags
Summary
This document provides a detailed explanation of the fundamentals of the nervous system, including ion distribution across cell membranes and the principles of membrane potential. The text defines and elucidates the roles of critical components such as the Na+/K+ ATPase and the action potential.
Full Transcript
Biology 2 Basics of the Nervous System 2 Meritxell Canals, PhD Learning Objectives To be able to describe: The distribution of ions across cell membranes and the equilibrium potential The origin of the membrane potential (Vm) Relationship between equilibrium potential (E) and...
Biology 2 Basics of the Nervous System 2 Meritxell Canals, PhD Learning Objectives To be able to describe: The distribution of ions across cell membranes and the equilibrium potential The origin of the membrane potential (Vm) Relationship between equilibrium potential (E) and membrane potential (Vm) Relationship between membrane potential (Vm) and ion concentrations ([ion]) Role of the Na+/K+ ATPase Action potential (AP) generation and propagation along nerves How local anaesthetic (LA) drugs work and may be used therapeutically Excitable cells Excitable cells use potential energy to do work Muscle cells (skeletal, cardiac and smooth) Contraction Immune cells move migrate Nerve cells (Neurons) transmit signals over relatively long distances The differences in ion concentrations across the nerve cell membrane provide the potential energy required to transmit nerve impulses Action potentials Membrane potential (Vm) Ions are present in different concentrations inside and outside the cell, and the membrane of excitable cells has differing permeabilities for different ions. The membrane potential (Vm) is due to the separation of electrical charges across the cell membrane. Variations in Vm All cells have a resting membrane potential (Vm) The resting potential varies with cell types -30 mV -70 mV -90 mV In some cells, the resting potential is static Homeostasis Used as an energy source for substrate transport In other cells, the membrane potential changes with… Signal transduction Action potentials Na+ / K+ ATPase The Na+/K+ ATPase (“sodium pump”) actively transports Na+ and K+ in different directions across the membrane. Requires metabolic expenditure (ATP) to push ions against their gradients Is so efficient that maintains the concentration gradients of Na+ and K+ (guidetopharmacology.org) Na+ / K+ ATPase Ion distribution Ions are present in different concentrations inside and outside the cell, and the membrane of neurons has differing permeabilities for different ions. Extracellular Relative Ion* Intracellular (mM) (mM) permeability Na+ 145 10 1 K+ 5 145 100 Cl- 145 5 10 A- (organic anions, 10 150 0 (impermeant) proteins) Ca2+ 1.5 0.0001 Very small * other ions have been omitted for simplicity Ion distributions Inside the cell Outside the cell * other versions of bananas and sports drink exist - these are for illustration purposes The resting membrane potential Overall, inside and outside the cell there is electrical neutrality: positive charges = negative charges Outside - Na+ is balanced mainly by Cl- Inside - K+ is balanced by organic anions (A- - e.g. proteins, phosphates) The resting membrane potential Overall, inside and outside the cell there is electrical neutrality: positive charges = negative charges Outside - Na+ is balanced mainly by Cl- Inside - K+ is balanced by organic anions (A- - e.g. proteins, phosphates) The resting membrane potential is possible due to the relatively high membrane permeability of K+ K+ leak channels are always open, i.e not “gated” The action of the Na+-K+ ATPase means that there is a K+ cycle The resting membrane potential The resting membrane potential is possible due to the relatively high membrane permeability of K+ K+ leak channels are always open, i.e not “gated Negatively charged species line up on the other side of the membrane This local separation of charges constitutes the origin of resting membrane potential (Vm) The electrochemical equilibrium Membrane selectively permeable to K+ ions Equilibrium potential (E): Voltage at which the electrical force (INSIDE) (OUTSIDE) A- A- K+ K+ K+ experienced by an ion is equal and opposite to the chemical force A- K+ produced by the concentration gradient. A- K+ C K+ No net force, no net ion flow A- A- e The ion is in electrochemical equilibrium K+ K+ K+ K+ A- A- Each ion has an equilibrium potential (Eion) An equilibrium is For K+, the equilibrium potential is called EK established where the For a membrane solely permeable to K+ Vm = electrical gradient compensates the EK concentration gradient The Nernst equation Mathematical expression of the equilibrium potential for a particular ion. The equilibrium potential for K+ described by the Nernst equation is: R = Gas constant + [ ] ≈ ( )= 10 + T = Temperature in K [ ] F = Faraday’s constant + The voltage across the membrane is proportional to the ratio of [ ] ( ) = 61.5 10 + the ion concentration on either side of the membrane [ ] (*assuming that the membrane is permeable to those ions*) 𝑖 𝑖 𝐾 𝐾 𝐹 𝐾 𝐸 𝑖 𝑛 𝑚 𝑉 𝐿 𝑜 𝑔 𝑉 𝑚 𝐸 𝑖 𝑛 𝑚 𝑉 𝐿 𝑜 𝑔 𝑜 𝑜 𝑅 𝑇 𝐾 𝐾 Applying the Nernst equation Extracellular Intracellular Relative Ion* + (mM) (mM) permeability [ ] ( ) = 61 10 + Na+ 145 10 1 [ ] K+ 5 145 100 Cl- 145 5 10 Equilibrium potential for K+ 5 = 61 10 = -89 mV 145 Equilibrium potential for Na+ ENa = +71 mV Equilibrium potential for Cl- ECl = -89 mV Vm is actually governed by multiple ion concentrations (not only K+), so the Nernst equation is a simplification 𝑖 𝐾 𝐾 𝐾 𝐸 𝑖 𝑛 𝑚 𝑉 𝐿 𝑜 𝑔 𝐸 𝐿 𝑜 𝑔 𝑜 𝐾 The Goldman equation A more realistic approximation of membrane potential takes into account the permeability of the membrane to other ions Goldman equation + + − [ ] + [ ] + [ ] = − 58log +] + − [ + [ ] + [ ] where Pion is the permeability of the ion (measured using radioactive ions) As long as the overall concentrations of the ions don’t change (they don’t!), the membrane potential (Vm) is controlled by changes in ion permeability due to opening and closing of protein ion channels in the membrane. 𝑜 𝑢 𝑡 𝑜 𝑢 𝑡 𝑖 𝑛 𝐾 𝑁 𝑎 𝐶 𝑙 𝑃 𝐾 𝑃 𝑁 𝑎 𝑃 𝐶 𝑙 𝑚 𝑉 𝑖 𝑛 𝑖 𝑛 𝑜 𝑢 𝑡 𝐾 𝑁 𝑎 𝐶 𝑙 𝑃 𝐾 𝑃 𝑁 𝑎 𝑃 𝐶 𝑙 Resting Vm and polarization The resting membrane potential (Vm) of cells is negative (-50 to -90 mV) A cell depolarizes when Vm becomes less negative A cell hyperpolarizes when Vm becomes more negative. Clinical Case Symptoms: History: Bloods: Weakness and fatigue Confusion, muddled Recent episode of excessive [K+] in blood ~ 3mM Arrhythmias (disruption of heart vomiting and diarrhoea (normal range 4-5 mM) rhythm) How do we explain the Hypokalemia symptoms? hypo = less kal = potassium emia = blood Understanding the basis of Vm and its relationship with ion concentrations Clinical Case Nausea and diarrhoea Loss of K+ in stomach and intestines [K+]o = 4 mM [K+]o = 3 mM Vm ~ -60 mV Vm ~ -64 mV [K+]i = 145 mM Cells hyperpolarize (Vm more negative) [K+]i = 145 mM Cells are less excitable Less contractions of muscles Fatigue Less neurons firing Confusion Neuronal signalling - Action Potentials Signal propagation in the nervous system From Year 1: Neuronal signalling Signalling in the nervous system is binary Neurones are either off or on Signals may have to travel long distances along nerve axons Neuronal signalling involves rapid changes in membrane potential (Vm) Action potentials (APs) As a binary system, ”strength” of the signal will be determined by the frequency of APs Voltage-ion channels (VGIC) are responsible of the rapid changes in Vm Action Potentials (APs) 1. An initial change in membrane potential (depolarization) is required 2. When the stimulus reaches a threshold depolarization, an action potential is generated the cell “fires” APs are binary (all or nothing) 3. APs are very short lived (~1-2ms) 4. After repolarization, an hyperpolarization period follows refractory period AP steps 1 Initial depolarization 2 Depolarization Stimulus needs to reach a When depolarization reaches threshold threshold voltage-gated Na+ channels open, increasing Stimulus can be due to opening depolarization. of small capacitance ligand- gated cation channels (graded responses can reach a threshold - can summate) 3 Repolarization 4 Hyperpolarisation (undershoot) Voltage-gated K+ channels soon Voltage-gated K+ channels open to reverse the change in remain open after the Vm has Vm. reached resting level refractory period At the same time, voltage-gated Na+ channels close (are inactivated) Ion movements vs permeability The actual number of ions that move during an AP is very small relative to the total number in the neuronal cytosol Radioactive Na+ can be used to follow ion movements in single axons during an AP ~ one K+ ion per 3,000-300,000 in the cytosol (0.0003 - 0.03% depending on the size of the neuron) is exchanged for extracellular Na+ to generate the reversals of membrane polarity It is the change in membrane permeability that underlies AP, there are no significant changes in the “bulk” concentrations of ions Reversal of the AP (repolarization) does NOT rely on “pumping out” excess Na+ Pharmacological tools to study APs Tetrodotoxin (TTX) blocks neuronal voltage-gated Na+ channels (VGSC) + TTX Abolishes AP (but not initial depolarization) Tetraodontidae (pufferfish) Tetraethylammonium (TEA) blocks neuronal voltage-gated K+ channels + TEA Slows repolarization (due to inactivation of VGSC only) No hyperpolarisation Ouabain is a Na+/K+ ATPase inhibitor Reduces the size of the AP progressively, until the membrane Na+ gradient is reduced to the point where APs can be initiated, but fail APs - Refractory periods 50 50 50 50 25 25 25 25 Membrane potential (mv) Membrane potential (mv) (mv) potential (mv) 0 0 0 Membrane potential -25 -25 -25 threshold potential threshold potential threshold potential Membrane -50 -50 -50 -75 -75 -75 -100 -100 -100 0 10 20 30 40 0 10 20 30 40 0 10 20 20 30 30 40 40 Time (ms) Time (ms) Time Time (ms) (ms) Stimulus 50 25 Relative Stimuli DON’T summate Membrane potential (mv) 0 Refractory Cells DO show refractoriness Period -25 threshold potential -50 -75 -100 0 10 20 30 40 Time (ms) Information flow through APs Action potentials encode information by frequency not amplitude Upper frequency limit imposed by refractory period 40 20 Membrane potential (mv) 0 -20 -40 -60 -80 0 20 40 60 80 100 Time (ms) Frequency vs amplitude APs - Refractory periods The refractory period is due to the inactivation of voltage-gated Na+ channels RRP Propagation of AP along an axon At rest the membrane is polarised Ion channels in the axon are voltage-gated. Depolarisation due to AP sets up local circuit currents in both directions. Depolarisation at one axon segment triggers the opening of Na+ channels in the next segment. In the “backwards” direction, Na+ channels are in refractory phase (inactivated) so that another AP cannot be generated The AP moves forwards Direction of travel of AP Speed of conductance The speed at which APs travel along nerve axons is regulated by: Temperature The capacity of ions to move across the plasma membrane is limited by the temperature Axon diameter The fatter the axon the lower the longitudinal resistance the faster the conductance of the APs Hodgkin & Huxley (1963 Nobel Prize in Physiology / Medicine) and the description of the ionic mechanism of AP. Rapid conduction speed due to their increased diameter 0.5-1 mm diameter conduction ~ 25m/s (100m in 4s) Myelination Presence of a phospholipid layer that surrounds particular nerve fibers Myelination The myelin sheath is formed by proteins and phospholipids forms an insulating layer around the axon membrane to allow electrical impulses (APs) to transmit quickly and efficiently. Myelination is a more efficient way to increase AP conduction than increasing axon diameter Myelination is provided by glial cells (or Glia) CNS: Astrocytes, microglia and oligodendrocytes (oligodendrocytes wrap multiple CNS axons with myelin) PNS: Schwann cells apply myelin to single peripheral axons Myelination - saltatory conduction Direction of travel of AP Ion channels are only distributed at the Nodes of Ranvier Saltatory conduction refers to the rapid propagation of the AP from each node to the next (the AP seems to “jump” between nodes) This mode of conduction allows for faster propagation of signals Nerve fiber classification Primary afferent axons Nerve fibers are classified according to: Diameter Myelination Speed of conduction Aα Aβ Aδ C Diameter Conduction Time for 100m Fibre type Myelin (µm) Velocity (m/s) (s) Aα motor 12-20 70-120 0.8-1.4 Yes Aβ touch 5-12 30-70 1.4-3.3 Yes Aγ motor 3-6 15-30 3.3-6.7 Yes Aδ pain 2-5 12-30 3.3-8.3 Yes C pain 0.3-1.3 0.5-2.3 44-200 No Consider placing your toe in a hot bath: Detect wet before hot Neuronal transmission and Pain Pain involves neuronal transmission Targets to treat Alterations in pain modulate neuronal neuronal transmission can transmission cause pain Gated ion channels Voltage-gated Ligand-gated Tension (stress) -gated +++ +++ CLOSED --- --- + + OPEN - - Neuronal firing refers to the rapid depolarization caused by opening of voltage- gated cation channels Voltage-gated ion channels (VGIC) Structure: They are similar for different ion species (Na+, K+, Ca2+) Central pore forming subunit through which ions travel down their electrochemical gradient CaV has 4 domains of 6TM (guidetopharmacology.org) Kv has 4 subunits of 6TM (guidetopharmacology.org) Nav has 4 domains of 6TM (guidetopharmacology.org) 6TM domains/subunits Auxiliary 1TM subunits 5 hydrophobic domains 1 +ve charged voltage sensor (S4) 𝝰 VGIC structure - examples www.guidetopharmacology.org DOI: (10.1152/physrev.00052.2017) VGSC and pain transmission VGSC are key contributors to action potentials. In the context of pain: once a stimulus has been applied to a sensory terminal, a transduction element is activated, resulting in an ion flux. VGSCs are then required to amplify this signal which, once threshold is reached, triggers a regenerative action potential, transmitting this information to the spinal cord. DOI: (10.1152/physrev.00052.2017) Local anaesthetics LAs function LAs inhibit VGSC (by binding to the intracellular side) Block conduction of APs LAs are weak bases that need to cross the plasma membrane in the un-ionized form (B) but bind to VGSC in the ionized form (BH+) Local anaesthetic TTX (tetrodotoxin) (procaine, lignocaine) B Extracellular Medium pH = 7.4 Plasma membrane Cytoplasm pH ~ 6.8 B BH+ Toxicity! Cross the PM at neutral charge Become ionized intracellularly Bind strongly to activated VGSC LAs - examples and metabolism LAs - examples and metabolism N O O Proxymetacaine (pKa 9.0) N Lidocaine (pKa 8.0) N O NH O NH2 O O O O N HN Procaine (pKa 8.1) Prilocaine (pKa 7.9) O NH Cocaine (pKa 8.6) O O Natural metabolite of coca plant Led to development of other LAs Inhibits VGSC Interacts with monoamine NH2 transporters Esters are metabolised by Amides are metabolised by cholinesterases (mainly in the liver amidases plasma) implications for liver failure patients LAs are weak bases All LAs are weak bases 7.5 < pKa < 9 H+ LA can exist in the un-ionised (B) and ionised (BH+) forms B H+ BH+ pH pKa defines the pH at which both forms exist in equal amounts when pH = pKa [B] = [BH+] Changes in pH will alter the proportion of drug in ionised vs un-ionised form Henderson-Hasselbalck equation + when pKa = pH log [BH+]/[B] = 0 [BH+]/[B] = 1 [BH+] [ ] − = = [B] [ ] when pKa > pH log [BH+]/[B] > 0 [BH+]/[B] > 1 [BH+] > [B] when pKa < pH log [BH+]/[B] < 0 [BH+]/[B] < 1 [BH+] < [B] 𝐵 𝑎 𝑝 𝐾 𝑝 𝐻 𝑙 𝑜 𝑔 𝐵 𝐻 H+ LAs are weak bases B H+ BH+ At physiological pH (pH 7.4), all LAs are more ionised (BH+) than un-ionised (B) as pKa > pH [BH+] > [B] However, the proportion of B and BH+ vary between different LA drugs Lidocaine (pKa 7.9) at pH 7.4 25% is un-ionised Bupivacaine (pKa 8.1) at pH 7.4 15% is un-ionised B pH = 7.4 A LA must enter the cell (cross the PM) in order to have its effect PM The un-ionised drug will cross the PM more readily than the ionised drug pH ~ 6.8 The drug which is more un-ionised at physiological pH will reach its target B more quickly than the drug which is less so BH+ Lidocaine has a faster onset of action than bupivacaine The ionised (BH+) drug: cannot readily pass back through the membrane (is “trapped”) binds to and blocks VGSC Differential sensitivity to LAs Nerve bundles consist of multiple fibre types Myelinated and thicker axons are more difficult for LAs to penetrate than thin, unmyelinated axons Peripheral nerve cross-section C fibres transmitting pain signals are easier to block than A fibres Diameter Conduction Fibre type Myelin LA sensitivity (µm) Velocity (m/s) Aα motor 12-20 70-120 Yes + Aβ touch 5-12 30-70 Yes ++ Aγ motor 3-6 15-30 Yes +++ Aδ pain 2-5 12-30 Yes +++ C pain 0.3-1.3 0.5-2.3 No +++++ LAs reduce pain at lower doses and more rapidly than they affect other sensations and motor function Applications of LAs - Acute Pain Surface (topical) anaesthesia Infiltration Intravenous regional Sprays for mucus membranes, Injection into tissues to reach nerves Injected distal to pressure cuff (that corneal drops Minor surgery stops blood flow) Lidocaine, tetracaine, etc. Most LAs Limb surgery Not generally effective for skin Adrenaline might be added Prilocaine, lidocaine but EMLA (eutectic mixture of LAs vasoconstrictor to prevent diffusion Danger of systemic toxicity if cuff lidocaine and prilocaine) produces of LA away from site released prematurely local anaesthesia in about 1 h. not for fingers/toes (risk of ischemia) Nerve block Spinal Epidural Injection close to nerve trunks to Injection into subarachnoid space (containing Injection into epidural space blocking CSF) to depress spinal roots and spinal cord reduce sensation peripherally Glucose sometimes added (increase density) to spinal roots Surgery, dentistry limit spread by tilting patient Lidocaine, bupivacaine Surgery on abdomen, pelvis, leg (if GA not As for spinal applications and possible) Risks of CVS effects and respiratory depression “painless” childbirth Spread to brain avoided (tilt) Post-op urinary retention common due to block of pelvic autonomic outflow Unwanted effects of LAs Unwanted effects are due mainly to escape of local anaesthetics into the systemic circulation. Main unwanted effects are: Central Nervous System CNS effects: agitation, confusion, tremors (progressing into Cardio- convulsions) and respiratory depression (exception, cocaine) vascular System CVS effects: myocardial depression and vasodilatation, leading to Allergies fall in blood pressure Hypersensitivity reactions Self test 1. What types of molecules account for most intracellular anions? 2. Why is the plasma membrane more permeable to K+? 3. What would be the effect of a drug that blocked the resting K+ permeability on the resting membrane potential of nerve cells? 4. What would be the consequence of hyperkalaemia on the membrane potential of nerve and muscle cells and on their excitability? 5. What sorts of drugs affect plasma K+? Self-test 1. How many transmembrane domains does a voltage-gated sodium channel have? a. What structural component allows it to sense Vm? 2. Sketch a diagram of the action potential a. Identify the ion channels & transporters involved in each step b. Identify the ions involved in each step c. Identify which way the ions are crossing the cell membrane (influx or efflux) 3. What are the differences and similarities between Ad and C fibres? 4. At what pH should local anaesthetics be formulated? 5. Identify three routes of administration of local anaesthetics Local Anesthetics Practical Aim: To design a valid experiment in which bias is minimized; “How can we optimise experimental design in order to identify the effects of local anaesthetics in humans?” Objectives (in consultation with a demonstrator) To design an experiment to test the efficacy of the creams against Innocuous and painful mechanical stimuli Heat Cold To measure the time course/duration of the effect Preparation Bring your course handbook with you There will a series of revision questions for you to consider when the protocol is established Bags and coats should be left on the window sills at the back of lab C1b Mobile phones should be switched off and left in your bags for the duration of the practical class Safety in the lab is everyone’s concern Look out for your colleagues! Read forms and information on Moodle Volunteers (short sleeve, groups of 4-5, all female/male group possible, need to fill in consent forms).