Biology 1B - Atoms, Bonds, Water, and Membranes PDF
Document Details
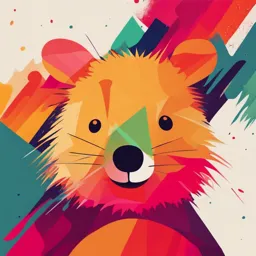
Uploaded by GroundbreakingSaxophone2873
Tags
Summary
These notes cover basic concepts in biology, focusing on atoms, bonds, water, and membranes. It explains atomic structure, different types of bonds, water's properties as a solvent, and the role of cell membranes. It also discusses the function of various organelles and membrane transport.
Full Transcript
At oms , bon d s , w a t er a n d membr a n es What are atoms? What are bonds? Which are important for life? Why is water a ‘universal solvent’? What are membranes? Where are they found? How do membranes form cell boundaries? How do they support bioenergetics?...
At oms , bon d s , w a t er a n d membr a n es What are atoms? What are bonds? Which are important for life? Why is water a ‘universal solvent’? What are membranes? Where are they found? How do membranes form cell boundaries? How do they support bioenergetics? What are organelles and what are their roles? Biology, Campbell&Reese 8th ed., pp. 74-77, 94-135 Wh a t a r e a t o m s ? Wh i c h a r e e s s e n t i a l ? Atoms are the smallest particles that retain the properties of an element (eg. carbon, oxygen, etc.) Atoms are made of sub-atomic particles called protons, neutrons, electrons Elemental properties of an atom are determined by its atomic number (=number of protons) Most atoms have equal numbers of protons and neutrons, but some isotopes have additional neutrons (eg. carbon 14, 14C has 6 protons and 8 neutrons; its breakdown is used in so-called ‘carbon dating’ materials) Wh a t d e t e r m i n e s c h e m i c a l b o n d i n g ? Physical chemistry of an atom is determined by its complement of electronic charge (e-) and their distribution Electrons distribute around the atomic nucleus in spaces described as ‘shells’ and ‘orbitals’ which define the probability distribution of an electron (or electrons) Electrons move between shells only on input/release of energy Wh a t d e t e r m i n e s b o n d f o r m a t i o n ? Bonds arise w h en at om s sh are or ex ch ange electrons in order to fill or empty shells The most stable atomic states are ones * with paired electrons * with filled electron shells Atoms with unpaired electrons and partially-filled outer shells react t o f ill or em pt y th e shell. E lectro n sh ells d efine th e P erio d ic T ab le o f E lem ents s or ll ac e do e e rs pt e - c ti v e - c ti v sh fi l b le no ce le d a a a Re Re St Wh i c h b o n d s a r e i m p o r t a n t f o r l i f e ? Ionic bonds arise when atom s exchange electrons Bond capacity is also known as the atomic valence (Don’t confuse atomic valence with ionic valence! here Na has an atomic valence of 1 and Cl of 7 but Na+ has an ionic valence of +1 and Cl- of -1; N.B. atomic and ionic valence are related. How?) Wh i c h b o n d s a r e i m p o r t a n t f o r l i f e ? Covalent bonds arise when atom s share electrons Compound structure is determined by the valence shells in the bonds... e.g. determines protein structure!! Wh i c h b o n d s a r e i m p o r t a n t f o r l i f e ? Weaker interactions are also important Hydrogen bonds - partial charge interactions between polar molecules Van der Waals interactions arise from locally induced dipoles between atoms in very close proximity Wh y i s w a t e r a ‘ u n i v e r s a l s o l v e n t ’ f o r l i f e ? Water (H2O) is a polar molecule and forms hydrogen bonds Hydrogen bonding explains * cohesive and adhesive properties * high specific heat (hence a good insulator) A d h e sive /co h e sive fo rce s in w e tting and cap illary actio n Water (H2O) is a polar molecule and forms hydrogen bonds Hydrogen bonds give water its crystal structure and ridgidity in ice and Cohesive tension limits tree size enable plant transpiration to approx. 120 m http://news.bbc.co.uk/2/hi/science/nature/3643899.stm Wh y i s w a t e r a ‘ u n i v e r s a l s o l v e n t ’ f o r l i f e ? Water (H2O) is a polar molecule and forms hydrogen bonds The polarity of water allows * solvation interactions with charged (ionic) compounds (forms ‘hydration’ shells) * solvation interactions with uncharged polar compounds * high reactivity with many other transition metals/atoms Wh a t a r e m e m b r a n e s ? Membranes are phospholipid polymers of fatty acids, glycerol, phosphate and a terminal amine or alcohol group Glycerol ester linkage Fatty acid Phosphatidyl choline comprises choline, a phosphate linkage to glycerol which is esterified with two fatty acids Hydrophillic head group Hydrophobic tail groups Wh a t a r e m e m b r a n e s ? Membrane phospholipids are amphipathic molecules – in water they spontaneously form monolayers and bilayers [Simple detergents are salts of fatty acids (lye soap) which retain this amphipathicity and are able to interact and dissolve other organic compounds] Wh y a r e m e m b r a n e s i m p o r t a n t ? Cellular membranes function … * to compartmentalize metabolic activities * to separate/protect cellular components * to provide a ‘scaffold’ for signalling * as a medium for cellular energy generation Compartmentalization, protection and energy generation arises from the immiscibility of water and membranes Sucrose diffusion across membrane bilayers is 1,000,000-fold slower (10-6 less) still Wh a t d o d i f f u s i o n r a t e s m e a n ? How long does it take sucrose to diffuse a distance x? So the physical properties (diffusion) of compounds in water limits membrane dimensions and cell size! B i ol ogi c al membr an es hol d c har ge Diffusion of ions (charges) is driven by both chemical and electrical gradients Selective diffusion across semi-permeable membranes generates a small ion (charge) imbalance The Nernst Equation describes the equilibrium between chemical and electrical forces on a charged molecule (ion) Ex = -(RT/zF).ln([X]in/[X]out) Ex = -60mV.log10([X]in/[X]out) B i ol ogi c al membr an es hol d c har ge Ion (charge) gradients across membranes are used to generate biological energy, a process of bioenergetics The voltage across the cell membrane can be measured using microelectrodes implanted in cells Note: voltage across endomembranes (eg. mitochondria, lysosomes) can be measured with voltage-sensitive dyes More on membrane voltage and bioenergetics tomorrow Wh e r e a r e m e m b r a n e s f o u n d ? Delimiting all organelles in eukaryotic cells Wh e r e a r e m e m b r a n e s f o u n d ? mitochondria chloroplast vacuole nucleus Golgi endoplasmic reticulum Electron microscopy gives a static picture of the cell Fl uor es c en c e – a n ew wi n dow on the c el l Jablonski diagram How does it work? Light absorbed by a pigment ‘excites’ electrons Energy is released as light as the electron relaxes back to the ground state (lowest empty electron shell) Gr een Fl u or es c en t P r ot ei n ( GFP ) Aquoria victoria (jellyfish) includes genes for intrinsically-fluorescent proteins Aequoria victoria SYP121 is a trafficking protein GFP normally secreted in secGFP- transfected leaves (here stained with propidium iodide) GFP accumulates in ER and Golgi when co-transfected in leaves with SYP121ΔC Plasma membrane Syp121 Exocytosis Golgi Endocytosis Anterograde Endoplasmic reticulum Retrograde Vacuole Nucleus Cell wall Geelen, et al. (2002) Plant Cell 14, 387 How autonomous are c hl oropl as ts ? Chloroplasts and mitochondria – endosymbiont progenitors suggested by double membranes Chlroplasts and mitochondria undergo independent division/replication Me m b r a n e s t r u c t u r e a n d f u n c t i o n Membranes are phospholipid polymers which are amphipathic and spontaneously assemble mono/bilayers in water Membranes serve a number of roles in cells (you’ll hear more about this tomorrow) as physical barriers and structures Organelles are membane-delimited compartments in eukaryotic cells Cells and organelles are highly dynamic The Inner Life of the Cell Animation (youtube.com) Prof. Anna Amtmann SMB, CMVLS Membrane transport Not: the transport of membranes. Deals with the transport of molecules (in particular ions) across biological membranes. ‘Trans’- membrane transport, ion transport, nutrient transport Why is membrane transport important? Necessity of life to protect metabolic reactions within the cell against the environment. Necessity of life to communicate and exchange materials between the cell and the environment. Transport proteins in the cell membrane allow for the ‘controlled’ interaction of the cell with the environment. Membrane permeability High permeability for small hydrophobic molecules and gases (e.g. O2) Limited permeability for water. Very low permeability for ions (e.g. K+) and large solutes (e.g. glucose). Transport proteins create a hydrophilic passage. create a filter. provide possibility for energy coupling. provide possibility for regulation. ‘Facilitated diffusion’ Transport proteins create a hydrophilic pore Molecules diffuse through this pore Example: water channel (aquaporin) Driving forces for solute transport 2 types of forces drive the movement of molecules across membranes: Chemical gradient = concentration gradient Electrical gradient = charge gradient Only relevant for charged molecules (ions). Cations: Anions: e.g. e.g. Proton H+ + Chloride Cl- + _ Potassium K+ - Nitrate NO3- Ammonium NH4 + Glutamate Histidine Malate Spermidine Pyruvate The electrochemical gradient = the net driving force for the movement of a molecule resulting from the combination of the chemical and electrical gradient. + + + – – + + + + + – + – + + in – + out – + Case 1: Uncharged molecule. Case 2: Cation. + _ + _ _ – _ _ – _ + – + _ _ _ – _ _ _ – _ – + – + – Case 3:Anion: Direction of the net driving force depends on the relative sizes of chemical and electrical gradients. Test your understanding + _ _ – _ + _ _ – _ _ – + Inside Outside The net driving force for the anion is directed inward because A) The chemical gradient is larger than the electrical gradient. B) The electrical gradient is larger than the chemical gradient. C) I haven’t got a clue! Energy requirements of transport Energy Energy investment gain The electrochemical gradient determines the energy requirement of transport + + + – – + + + + + – + – + + in – + out – + Case 1: Uncharged molecule. Case 2: Cation. + _ + _ _ – _ _ – _ + – + _ _ _ – _ _ _ – _ – + – + – Case 3:Anion Active and passive transport Active transport moves substances against the electrochemical gradient. It requires the input of energy. Passive transport moves substances down the electrochemical gradient. It requires no input of energy. ?! Note however that energy was invested to establish the electrochemical gradient. This means that active transport is a prerequisite for passive transport. Active and passive transport Transport proteins for active transport: Pumps Co-transport systems Transport proteins for passive transport: Channels Carriers Pumps Sodium/potassium pump: Energy coupling ATPases: transport is coupled to the hydrolysis of ATP Pumps can also be driven by light energy e.g. bacteriorhodopsin Conformational change Examples: Sodium/potassium pump Proton pump Calcium pump Pumps Pumps establish electrochemical gradients – EXTRACELLULAR + FLUID ATP – + H+ H+ Proton pump H+ – + H+ H+ – + CYTOPLASM These gradients can be used to drive the active transport of other molecules Co-transport systems Co-transporters couple the downward movement of one ion (driver) to the uphill movement of another solute (substrate). Symport: Driver ion and substrate move in the same direction (‘piggyback’ principle) Co-transport systems Co-transporters couple the downward movement of one ion (driver) to the uphill movement of another solute (substrate). Antiport: Driver ion and substrate move in the opposite direction (‘revolving door’ principle) Transport coupling is ubiquitous The functional linkage of primary pumps with co-transport systems - is inherent to all life forms - occurs in different cells and organelles Test your understanding The salt bush (Atriplex) survives in saline environments because salt bladders on its leaves actively remove Na+. In a plant exposed to high salt, which transport system is needed to remove the toxic Na+ from the cells? A) A Na+/H+ symporter. B) A Na+ channel. C) A Na+/H+ antiporter. Passive transport Passive transport moves substances down the electrochemical gradient. It requires no input of energy but relies on previously established electrochemical gradients Transport proteins for passive transport: Channels Carriers Passive transport A channel provides an aquous A carrier undergoes a conformational pore for the passage of ions. change that exposes ion binding sites to different sides of the membrane. Both pathways facilitate the movement of solutes down their electrochemical gradients. Ion channels are not just pores They exert a tight control of passage. They are selective e.g. some K+ channels have a 100fold higher permeability for K+ than for Na+. They are ‘gated’ = they can open and close upon specific stimuli, e.g. voltage, chemical ligands (remember: action potential). How can we measure ion channels? We can measure how single channel proteins gate using a method called patch clamp! current amplifier suction pipette open Channel current closed time Test your understanding Which piece of equipment is NOT usually required for a patch clamp experiment? A) A suction pipette. B) An amplifier. C) A helmet. Summary Ion transporters in the cellular membrane allow for the ‘controlled’ interaction with the environment. The electrochemical gradient is the combination of the chemical and the electrical gradient. Active transport moves substances against the electrochemical gradient. It requires the input of energy. Passive transport moves substances down the electrochemical gradient. It requires no input of energy. Pumps establish electrochemical gradients. These drive the active transport of other solutes through co-transport systems and the passive transport of solutes through channels and carriers. Channels exert control of passage through selectivity filters and ‘gating’ mechanisms. Stem Cells and Reproductive Ethics Dr. Kenneth Watterson [email protected] Intended Learning Outcomes Identify the essential characteristics of stem cells Understand stem cell potencies and how these relate to research applications Explain stem cell use in research Consider the ethical implications associated with stem cell research Understand the potential of stem cell derived products for therapeutic use What are Stem Cells? Unspecialised cells that can i) reproduce indefinitely ii) differentiate into one or more specialised cell types (potency) Embryonic and Adult cells Can generate tissues, organs or organisms Adapted from http://www.iscr.ed.ac.uk/outreach/about-stem-cells.html Stem Cell Potencies Totipotent – generate all the tissues of the embryo and extra-embryonic tissues, such as the placenta. eg: the zygote/ fertilized ovum Pluripotent – generate cells all three germ layers but not the extra-embryonic tissues. eg: embryonic stem cells STEM CELLS Multipotent – ability to differentiate into multi-lineages but not to all germ layers eg: haemopoietic stem cells, mesenchymal stem cells Unipotent – ability to differentiate along only one lineage. eg: most adult stem cells in differentiated undamaged tissues, in normal conditions. Hierarchy of Stem Cell Potency Embryonic Adult Stem Cells can be isolated from most tissues Adult tissues & organs - biopsy - bone marrow Umbilical cord blood - at birth Foetal tissues & organs Bone marrow - after pregnancy termination Autologous vs allogeneic - Autologous: take from an individual and return to same person - Allogeneic: take from an individual and return to a different person Umbilical cord http://www.acmc.uq.edu.au/Projects/Virtual_Placenta.html blood Reproductive Cloning Reproductive Cloning – Somatic Cell Nuclear Transplantation differentiated animal cells – don’t divide in culture or develop into multiple lineages removed nucleus of an egg (enucleated egg) Replace it with nucleus of differentiated cell (nuclear transplantation or somatic cell nuclear transfer) If nucleus from donor maintains full genetic potential, leads to development of the recipient cell into all tissues/organs of an organism Nuclear Transplantation in Xenopus Laevis John Gurdon and colleagues, Oxford (1970s) Nucleus from a differentiated frog cell can direct development of tadpole Efficiency decreases as the donor cell becomes more differentiated Paved the way for stem cell technology Cambell Biology Chapter 19, Figure 19.16 Mammalian Reproductive Cloning – Dolly the Sheep 1997 Roslin Institute - Cloned lamb from adult sheep by nuclear transplantation from differentiated cell From several hundred embryos, one successfully completed normal development (Dolly) Confirmed chromosomal DNA identical to nucleus donor Range of mammals subsequently cloned (mice, rats, cats cows, horses, pigs, dogs and monkeys) Mammalian Reproductive Cloning – Challenges Small percentage of cloned embryos develop normally to birth Cloned animals don’t always look/behave identically Aged 6, Dolly developed lung condition associated with older sheep – euthanised Speculated that Dolly’s cells were not as healthy as normal sheep (incomplete reprogramming of the original transplanted nucleus) Cloned mice prone to obesity, pneumonia, liver failure and premature death Human Reproductive Cloning – Banned Reproductive BANNED in humans 14d max time in culture Never implant into humans However, interest in cloning related to the ability to generate Stem Cells for Therapeutic Cloning Non-Reproductive (therapeutic)human cloning Human embryonic stem cells (hESC) Derived from inner cell mass (ICM) of blastocyst (IVF; d5 post-fertilisation) Self renew and expand indefinitely in culture Pluripotent - able to derive all cells from all three dermal layers Lung Blood Neural Liver Cardiomyocyte Skin Therapeutic Cloning Therapeutic Permitted Very difficult The application of SCNT to produce To make autologous ES cells Produce models of disease Patient-specific cell lines isolated from an Embryo Designed to replace injured/diseased tissues Not intended for in utero transfer http://www.nature.com/nrg/journal/v4/n11/box/nrg1205_BX1.html Stem Cells for Therapy Regenerative Medicine Repair Replace Restore Regenerate Replacement of cells or tissues after injury or disease with stem cell derived tissue Applications of Embryonic Stem Cells Basic research Drug testing - disease and patient-specific cells Toxicology - normal, human cell supply Drug discovery Therapy Data fabricated Unethically sourced oocytes http://stemcellbioethics.wikischolars.columbia.edu/The+Cloning+Scandal+of+Hwang+Woo-Suk Human cloning to derive hESC finally achieved in 2013 Shoukhrat Mitalipov Laboratory Oregon Stem Cell Center Ethical Objections to hES cells Derived from an embryo Very early stage, when is it a life? Range of policies for use of hESC across the world Sometimes based on religious belief Permissive – various techniques of derivation including SCNT Flexible – donation from IVF clinics, exclusion of SCNT, often under restrictions Restrictive or no established policy Teratoma An often benign tumour containing tissues of more than one germ layer, arising from totipotent cells, often occur in the ovary or testes. Teeth Hair Might arise if pluripotent cells were left in therapies of differentiated cells Current concerns in the clinical use of hESC Mainly mixed differentiation still, need to refine methods Potential for teratoma formation from undifferentiated hESC Small experimental scale, need to scale-up Off-the-shelf therapy would still require huge bank to tissue match, otherwise immune rejection issues Animal products used in culture, infection and immune risks Mouse Embryonic stem (ES) cells Isolated from the blastocyst stage embryo Murine ES cells are used to make: Transgenic mice Sir Martin J. Evans of Cardiff University in Wales. Mario R. Capecchi of the University of Utah in Salt Lake City; Oliver Smithies of the University of North Carolina in Chapel Hill; First reported in 1981, Nobel Prize for Medicine Oct 2007 http://www.nobelprize.org/nobel_prizes/medicine/laureates/2007/ Genetically modified animals Transgenic fluorescent marker gene expression introduced into ES Cells Knock-out mouse technologies, along with transgenic mice (over expressed genes) have massively changed the fields of basic and medical research over the last 20 years. All based on mES cells. Embryonic Adult Stem Cells Stem Cells Adapted from http://www.stemcellresearchfoundation.org Potential applications of adult Stem Cell Bone Liver Heart Blood CNS Cartilage Pancreas Hair Blood vessels Eye Skin Peripheral nerves Hierarchical systems proliferation Common Myeloid Common Lymphoid Precursor Precursor differentiation What are Hematopoietic Stem Cells (HSCs) used for? Bone marrow transplant – the original cell therapy Transplant into patients who need their blood system replaced: Leukaemia Sickle cell anaemia and thalassaemia Immune deficiencies Others? – only for blood/immune replacement eg: MS replace/reset auto-reactive immune system Other cancers – recovery of blood system after extreme chemo Currently all established uses of “bone marrow” transplant in the UK are for blood/immune system replacement not other tissues. ASC v. hESC in regenerative medicine Neither is ideal Adapted from http://www.stemcellresearchfoundation.org Somatic cells can be reprogrammed into induced pluripotent stem (iPS) cells Based on work of Yamanaka (2007) Re-program differentiated cells to act like Embryonic Stem cells Introduce four “stem cell” master regulatory genes (Myc, Oct3/4, Sox2, Klf4) Efficiency improved by addition of Nanog and LIN28 Deprogrammed cells know as induced pluripotent stem cells (restore pluripotency) http://www.rndsystems.com/cb_detail_objectname_cb09i2_induced_pluripotent_stem_cells.aspx The dual potential of induced pluripotent stem (iPS) cells for treatment 2 major potential uses for human iPS cells Cell therapy Patient’s own cells reprogrammed into iPS cells - replace nonfunctional tissues (insulin-producing cells of the pancreas) Research and Drug discovery Reprogrammed to become iPS cells - provide cellular model of the disease to understand the disease/develop treatments Nobel prize in physiology or medicine 2012 John B Shinya Gurdon Yamanaka Gurdon showed that Yamanaka achieved cells could be reprogramming of reprogrammed into a mature mammalian more immature state cells into stem cells in 1962 using by introducing a few amphibians genes http://www.nobelprize.org/nobel_prizes/medicine/laureates/2012/ Research potential of iPS cells Amyotrophic lateral sclerosis (Lou Gehrig's disease, Motor Neurone disease) Compare to normal cells Understand disease Look for drugs to revert condition Treat disease? Therapeutic potential of iPS cells – Type 1 Diabetes An exciting advance but..... Advantages Can now make person-specific cell lines No embryos damaged – ethical alternative to human embryonic stem cells Make lines from people with genetic disease and study lineages eg: ALS (Lou Gerig disease, Motor neurone disease) Problems Genetically modified Potential oncogenesis or damage to host genome Differentiation = hESC with similar limitations Stem Cell Regenerative Therapy - The key questions 1. Is cell replacement a practical solution? 2. Can cells be delivered effectively? 3. Mechanism of repair? Direct or Indirect Effect? Host or donor cell recovery? Ways to obtain stem cells (as of January 2014) Donated embryos Cloning ? Lymphocytes Low pH ? Embryonic stem cells Induced pluripotent Adult stem cells Stress Triggered Acquisition stem cells of Pluripotency (STAP) cells Reports in Jan 2014: Stimulus-Triggered Acquisition of Pluripotency 1 week old mouse cells Many questions, needs to be repeated and proven with human cells but very exciting! As of Jan 2015: Fraudulent. Resignations and worse… The papers garnered much excitement but immediately came under attack by social media that noted apparently manipulated images. Investigations by the RIKEN Center for Developmental Biology in Kobe, where Obokata works, found that she had committed scientific misconduct, and the papers were retracted. Cautionary tales! If it is safe and scientifically sound there will be an approved clinical trial Trials are closely regulated for the good of the patient If you have to go abroad and pay for the treatment there are many things to consider first Stem Cell Research…. Great excitement, great promise and great responsibility Regenerative Medicine Repair Replace Restore Regenerate You will think more about the possibilities and ethics associated with SC research and therapy in your Biology 1B group project Reputable sources of information Regulated clinical trials (Worldwide) ClinicalTrials.gov Basic Science Commercial treatments National Institute of Health website International Society for Stem Cell Research (ISSCR) http://stemcells.nih.gov/info http://www.closerlookatstemcells.org//AM/Template.cfm?Section=Home Cellular Energetics Dr Denise Hough Lecturer in Physiology, Ageing and Welfare School of Biodiversity, One Health and Veterinary Medicine, MVLS From Course Information Document ILO’s: – Explain the concepts of free energy and entropy – Use thermodynamics to explain why enzymes make metabolic pathways so effective – Describe the role of ATP in coupling processes that release energy with those that require energy – Explain the importance of oxidation-reduction reactions in energy generation – Define the purpose of glycolysis, krebs cycle, electron transport chain, chemiosmosis, oxidative phosphorylation and cellular respiration. – Name the main pathways and their location in the cell by which ATP is generated under aerobic and anaerobic conditions What is bioenergetics? Resource: Biology: A Global Approach Campbell text book, 12th Ed. Chapter 6: Energy and Life, p.141-153 Chapter 10: Cellular Respiration, p.236-241 How cells produce and use energy Is based on the laws of thermodynamics Relates to energy … capture (respiration, photosynthesis) storage (ATP, NADH, proton gradient) conversion (light, chemical, kinetic) Energy conservation and conversion 1. First law of thermodynamics: – No energy can be lost within the Universe – Energy can be converted from one form into another 2. Second law of thermodynamics: – Every energy transfer increases the entropy (chaos) of the Universe – Takes the environment into account Waste/chaos: Fuel: Heat & sound Chemical energy energy Work: Kinetic energy Energy conservation and conversion Not all forms of energy can be converted into biologically relevant work (e.g. movement/growth) An organism loses some of its energy to the environment (typically heat) An organism fixes some of its energy irreversibly (e.g. secondary metabolites in plants for taste/odour) Living organisms require constant energy input Irreversible fixing: Food: e.g. hair & secondary metabolites Chemical Waste/chaos: energy Heat Work: Fat storage: Kinetic Chemical energy energy Energy balance Ecosystem & Plants Individual – Energy conversion – Energy loss/heat Plants & Animals Cell’s energy currency Cellular respiration: metabolic process with which an organism obtains energy by oxidizing nutrients and releasing waste products Metabolism & ATP energy currency ATP acts as energy reservoir within the cell Energy used for cellular work (chemical, transport, movement ) Metabolism = totality of organism’s chemical reactions – Anabolism: use energy to build complex molecules – e.g. protein synthesis from amino acids – Catabolism: release energy through molecule breakdown – e.g. breakdown of glucose in glycolysis N e t Anabolism uses energy E n e r Catabolism releases energy g y Progress through metabolic pathway Gibbs free energy Universe = system + surroundings J. W. Gibbs 1878: Free energy of the system, G = the portion of the system’s energy that can perform work Change of free energy when the system changes (e.g. metabolic reaction, transport) DG = H - T S Change in the total energy Change in the disorder Temperature of the system (enthalpy) of the system (entropy) Rule: A reaction or transport process only occurs spontaneously if it decreases G (if G is negative). Chemical reactions require or A release energy A: Exergonic reaction: Amount of energy released G free energy i released b G0 s fr e Reactant Product e s s Enzymes regulate metabolism Metabolic pathways are made up of a group of enzyme- catalysed reactions Enzyme lowers activation energy (EA) required to kick- start reaction Enzymes are often pH- dependent Enzymes are regulated by gene expression and protein modification (e.g. phosphorylation ) Enzymes are often inhibited by the end product in pathway (negative feedback) ATP is used as an energy currency Adenosine-triphosphate (ATP) releases energy when the outermost inorganic phosphate (Pi) is cleaved off and yields adenine-diphosphate (ADP) The (chemical) energy is stored in the outermost Pi because the negative charges repel each other H2 O Energy Pi + ADP G= -7.3 kcal/mol (-30.5 How is ATP formed? A: Substrate-level phosphorylation B: Oxidative phosphorylation (transfer of phosphate group) (Chemiosmosis) e.g. glycolysis: e.g. ATP synthase in inner mitochondrial phosphoglycerokinase membrane Chemiosmosis: movement of ions down their electrochemical gradient across a semipermeable membrane Another energy source: Redox potential & electron transport chain 2 H (e.g. in sugar) Another form of energy is stored in the redox potential Reflects the different affinity of 2 H+ + 2 F e– atoms to incorporate or release re E Elec e electrons into/from their outer le tron e shell ct tran n spo Energy ro er = electronegativity. n rt g e chai y, Relocating electrons from g n G sugars (weakly electronegative) at 2 e– iv to oxygen (strongly it 2 1/2 y O2 electronegative) releases H+ energy. H2 O NADH is used as an electron currency Nicotinamide adenine dinucleotide Redox reaction: becomes oxidized Xe- + X + Ye- Y becomes reduced Similar: NADP+ NADPH, FAD FADH2 Many molecules of NADH are produced during the breakdown of food. How can we transform electron currency into energy currency? Energy harvest: From electrons to ATP CHEMIOSMOSIS – proposed by Peter Mitchell around 1960 Energy can be stored in a transmembrane proton (H+) gradient This energy can be used to make ATP Establishment of H+ gradient is a separate process from ATP synthesis A directional movement of H+ ions across the membrane is accompanied by a chain of reactions involved with electron transport Intact membranes are an essential requirement for chemiosmosis. Energy harvest: From electrons to ATP NADH used in oxidation-reduction (REDOX) reactions to transfer H+ with electrons in electron transport chain H+ gradient used to produce ATP What is cellular respiration? Cellular respiration: metabolic process with which an organism obtains energy by oxidizing nutrients and releasing waste products Resource: Biology: A Global Approach Campbell text book,12th Ed. Chapter 10: Cellular Respiration, p.236- 258 Involve pathways concerned with energy metabolism Organised across different subcellular locations What is the energy balance of respiration? Metabolism Metabolic pathways interact in a network Catabolic route: energy release Anabolic route: energy required The yellow highlighted area shows the pathways that we will discuss for cellular respiration: The breakdown of glucose to capture energy as ATP Cellular respiration can be divided into 3 stages Cellular respiration can be divided into 3 stages Cellular respiration can be divided into 3 stages Glycolysis Glycolysis Starts with glucose, a 6-carbon molecule – C6H12O6 1st step commits molecule to pathway and needs energy in the form of ATP Incorporates phosphate group * Enzyme name tip: – Kinase: adds phosphate Glycolysis Step 2 shifts the chemical bonds to form an isomer molecule * Enzyme name tip: – Isomerase: converts molecule from one isomer to another Glycolysis Step 3 incorporates another phosphate group and needs energy in the form of ATP * Enzyme name tip: – Kinase: adds phosphate Glycolysis Step 4 splits the 6-carbon molecule into two smaller 3-carbon molecules (enzyme type: lyase) Step 5 converts the one molecule, yielding 2 identical 3-carbon molecules Glycolysis Step 6 is the first redox reaction, where electrons are captured and carried by NADH * Enzyme tip: dehydrogenase enzymes oxidizes a substrate by reducing an electron acceptor Glycolysis Step 7 is the first payoff reaction A phosphate group comes from the 3-carbon molecule and gets added to ADP to make energy rich ATP * Enzyme = kinase Glycolysis Step 8 moves the chemical bonds around again in preparation for another substrate phosphorylation Glycolysis Step 9 cleaves off water * Enzyme tip: Enolase is a hydro-lyase type enzyme Glycolysis Final step: phosphate group taken to produce ATP, makes pyruvate Preparing pyruvate for citric acid cycle Also known as the “link reaction” Does not proceed when there is no oxygen Citric acid cycle Also known as: Tricarboxylic acid (TCA) cycle Or Krebs cycle Citric acid cycle One cycle with 1 Acetyl CoA: 3 NADH 1 FADH2 1 ATP Two cycles with 2 Acetyl CoA: 6 NADH 2 FADH2 2 ATP Recall that the link step which is the conversion of Pyruvate to Acetyl CoA generates 1NADH (happens twice) Aerobic respiration through glycolysis & citric acid cycle = 8 NADH and 2 FADH2 and 2 ATP Electron harvest and ATP production during respiration Electron transport chain and ATPsynthase Electron transport chain and ATPsynthase Note: the actual number of ATP produced through chemiosmosis is variable, see textbook page 178 for details or see if you can find out why (not examinable). (Nice video resource: www.khanacademy.org) Depending on whether oxygen is present or not, glycolysis feeds into respiration or fermentation Energy balance of fermentation (no or low oxygen) Fermentation produces 2 ATP per glucose (Compared to 32 ATP produced by oxidative phosphorylation!!!) O2 and oxidative phosphorylation led to an ‘explosion’ in evolution The basic principle of oxidative phosphorylation is chemiosmosis Summary Living organisms require constant energy input Energy can be converted from one form into another e.g. light energy chemical energy kinetic energy Exergonic reactions release energy that can be used for chemical work (endergonic reactions), transport and movement ATP is formed by substrate-level and oxidative phosphorylation of ADP and acts as an energy currency NAD+ captures electrons from redox reactions and in the form of NADH delivers them to the electron transport chain The electron transport chain establishes a proton gradient across the inner membranes of mitochondria and chloroplasts H+ flow back through membrane-bound ATP synthases which produce ATP The trans-membrane H+ gradient acts as a ‘high-energy’ intermediate between redox potential and ATP synthesis Summary Metabolism = totality of an organism’s chemical reactions, can be anabolic or catabolic Biochemical reactions are organised in metabolic pathways, which form complex metabolic networks Cellular respiration can be divided into three stages: glycolysis, citric acid cycle and oxidative phosphorylation Overall reaction for cellular respiration: C6H12O6 + 6 O2 → 6 CO2 + 6 H2O ∆G= -2870kJ/mol Cellular respiration produces approximately 30-32 ATP per glucose Under anaerobic conditions, glucose is fermented and only produce 2 ATP per glucose Metabolism is regulated by and through enzymes To do: Interactive Revision Use this Revision QuizBot to develop and test your knowledge on cellular energetics: Access via link/QR code: https://mizou.com/login-thread?ID=Gca_oOaKiF1WTjnWTrdwlVC9iww1mBNDnC6TObnWpzs-50720 It is in a chatbot style, which means that you can also ask for explanations, focus on particular topic or adjust difficulty level. It has speech-to-text and text-to-speech options Want to share your thoughts on this activity? Let me know here: https://forms.office.com/Pages/ResponsePage.aspx?id=KVxybjp2UE- B8i4lTwEzyHXNWIz1HMVOhmelIvZFwDtUM1lWUkdEWU41REEzRU NZS09COTFETTk3RS4u 1/20/21 Photosynthesis 1 – light reactions What are ’light’ and ‘dark’ reactions? How is light energy captured for biochemistry? What light is important? Where does photosynthesis take place? What is the ‘Z’ scheme? Biology, Campbell&Reese 7th ed., Chap. 10 1 What is photosynthesis? Photosynthesis: a process that converts atmospheric CO2 and H2O to carbohydrates Solar energy is captured in chemical form as ATP and NADPH ATP and NADPH are used to convert CO2 to hexose phosphates Phototrophs: photosynthetic organisms (some bacteria, algae, higher plants) 2 1 1/20/21 Photosynthesis divides between ‘light’ and ‘dark’ reactions 3 Light and Dark reactions Light (-dependent) reactions... Are membrane-bound Release O2 from splitting 2H2O molecules, with H+ from H2O used in the Chemiosmotic synthesis of ATP, and Hydride ion (H:-) from H2O reduces NADP+ to NADPH Dark (light-independent reactions... Occur in solution Reduce gaseous CO2 to carbohydrate Require energy of NADPH and ATP 4 2 1/20/21 Chloroplast structure Chloroplasts 4-8 mm diameter organelle two surrounding membranes internal membrane stacking 5 Chloroplast structure Chloroplasts 4-8 mm diameter organelle two surrounding membranes internal membrane stacking EM of chloroplast Freeze-fracture EM of grana 6 3 1/20/21 Chlorophyll and other pigments capture light Chlorophylls are most abundant and most important in light harvesting... contain tetrapyrrole ring (chlorin) similar to heme, but contains Mg2+ chlorophylls a (Chl a) and b (Chl b) in plants bacteriochlorophylls a (BChl a) and b (BChl b) are similar and major pigments in anaerobic photosynthetic bacteria 7 Chlorophyll and other pigments capture light ‘Antenna’ pigments include carotenoids (carotene pigments), phycocyanin and phycoerythrin 8 4 1/20/21 Chlorophyll and other pigments capture light Antenna pigments extend the range of light capture, especially in the green (where chlorophyll absorbs poorly) Antenna pigments and chlorophylls exchange light energy (excited electrons) until captured 9 Light absorption is organised between two Photosystems Photosystems I (PSI) and II (PSII): contain many proteins and pigments embedded in the thylakoid membrane PSI and PSII operate in series, connected by cytochrome bf complex Electrons are conducted from H2O to NADP+ Z-scheme 10 5 1/20/21 Light absorption is organised between two Photosystems PSI and PSII each contain a reaction centre (site of the photochemical reaction) chlorophylls in each reaction center are paired to capture light PSI paired for P700 (absorb light maximally at 700nm) PSII paired for P680 (absorb light maximally at 680nm) Z-scheme 11 Physical layout of light reaction steps 12 6 1/20/21 The Z-scheme Z-scheme: path of electron flow and reduction potentials of the components in photosynthesis Absorption of light energy converts P680 and P700 (poor reducing agents) to excited molecules (good reducing agents) Light energy drives the electron flow uphill NADP+ is ultimately reduced to NADPH Light is captured by antenna complexes For 2 H2O oxidized to O2, 2 NADP+ are reduced to 2 NADPH 13 Chemiososmosis and energy conversion Mitchell’s Chemiosmosis Theory – conversion of energy from electron transport via formation of a transmembrane electrochemical gradient Requires e- (H+) transfer across a ‘coupling’ membrane to generate DµH DµH = DY + DpH (DµH also sometime called the ‘proton motive force’) DµH used to drive protons through the ATP synthase (H+-ATPase) to make ATP 14 7 1/20/21 Photophosphorylation - H+-ATP synthase Photophosphorylation: synthesis of ATP which is dependant upon light energy Chloroplast ATP synthase consists of two major particles: CFo and CF1 CFo spans the membrane, forms a pore for H+ CF1 protrudes into the stroma and catalyzes ATP synthesis from ADP and Pi 15 Photophosphorylation - H+-ATP synthase Mitchell’s Chemiosmosis Theory – conversion of energy from electron transport via formation of a transmembrane electrochemical gradient Requires e- (H+) transfer across a ‘coupling’ membrane to generate DµH 16 8 1/20/21 Photophosphorylation - H+-ATP synthase Mitchell’s Chemiosmosis Theory – conversion of energy from electron transport via formation of a transmembrane electrochemical gradient Requires e- (H+) transfer across a ‘coupling’ membrane to generate DµH 17 Cyclic electron flow and phosphorylation For 4e- transferred to 2 NADPH, 2 ATP are produced from the proton gradient However, for each CO2 reduced to (CH2O) in carbohydrate synthesis, 2 NADPH and 3 ATP are required Cyclic electron transport yields ATP but not NADPH, thus balancing the need for 3 ATP for every 2 NADPH Ferridoxin donates e- not to NADP+, but back to the PQ pool via a specialized cytochrome Cyclic flow increases the protonmotive force and increases ATP production, but no NADP+ is produced 18 9 1/20/21 Summary Photosynthesis uses light energy to fix carbon into sugar Photosynthesis takes place in the chloroplast Light reactions capture and use light energy to reduce NADP and phosphorylate ADP Light drives photosynthetic electron transport to generate µH The µH is used by H+-ATP synthase to phosphorylate ADP ATP and NADPH energy is used for cellular reactions 19 10 1/20/20 HANDS-0N SUMMER-BREAK RESEARCH DOBBIE SMITH PRIZES University of Glasgow – Institute of Molecular, Cellular and Systems Biology and the Plant Science Research Group £1000 prize bursary and 4-8 weeks’ summer research experience Consult an approved member of the MCSB staff, agree a project, then … submit a letter of application to the Undergraduate School. Open to all first-year students in the Natural Sciences and allied studies at the University of Glasgow. Details available on the MOODLE site or from Prof. M. Blatt ([email protected]) The deadline for submissions is 12:00 noon Friday, 20.March 2020 1 Photosynthesis 2 – dark reactions What are the ‘dark’ reactions? How is carbon captured and fixed into sugar? What is RuBisCO? What is photorespiration? What is C4 photosynthesis? Biology, Campbell&Reese 7th ed., Chap 10 2 1 1/20/20 How were dark reactions identified? Fast CO2 injection Rapid ‘quench’ (perchloric acid) to stop reactions 2D chromatography improves separation of intermediates 3 How were dark reactions identified? Fast CO2 injection Rapid ‘quench’ (perchloric acid) to stop reactions 14CO 2 Chlorella Injection suspension Port Perchloric Acid Light Source & Heat Filters 4 2 1/20/20 14CO pulse-chase analysis of the C3 cycle 2 2D chromatography improves separation of intermediates 5 s labelling Time sequence of product 14C labelling indicates sequence of transitions 30 s labelling 5 Photosynthesis divides between ‘light’ and ‘dark’ reactions Calvin-Benson cycle identified in 1948 – led to Nobel prize in Chemistry Time sequence of product 14C labelling indicates sequence of transitions Key enzyme is RuBisCO (Ribulose-bisphosphate- caboxylase/oxygenase) 6 3 1/20/20 Light and Dark reactions Gaseous CO2 and the 5-carbon sugar ribulose 1,5-bisphosphate form two molecules of 3-phosphoglycerate (PGA) Reaction is metabolically irreversible RuBisCO makes up about 50% of the soluble protein in plant leaves, and is the most abundant enzymes in nature RuBisCO = 8 large subunits + 8 small subunits Large subunits nuclear-encoded; small subunits chloroplast-encoded 7 Chlorophyll capture light RuBisCO also use O2 to catalyze a competing oxygenation reaction Normally carboxylation is 3 times greater than oxygenation Photorespiration recycles the (toxic) products of the oxygenation reaction; it consumes NADH, ATP to give glyoxylate, serine, glycine and CO2 8 4 1/20/20 Alternative Photosynthetic Strategies Many plants, algae and photosynthetic bacteria (cyanobacteria) have evolved methods to get around the limitations of RuBisCO Cyanobacteria form Carboxysomes to concentrate CO2 around RuBisCO Some algae form Pyrenoids to concentrate CO2 around RuBisCO Some Angiosperms have evolved C4 photosythesis to ’feed’ RuBisCO Some Angiosperms have evolved CAM photosythesis to ’feed’ RuBisCO 9 Carboxysomes and Pyrenoids Cyanobacteria form Carboxysomes to concentrate CO2 around RuBisCO Carboxysomes are semi-crystalline protein structures incorporating RuBisCO Cyanobacteria express transport proteins to accumulate CO2 and HCO3- inside Pyrenoids are membrane-bound structures found in the chloroplasts of some algae Pyrenoids, like carboxysomes, include RuBisCO and concentrate CO2 10 5 1/20/20 C4 Photosynthesis C4 photosythesis evolved over 68 times in angiosperms (also known as the Hatch-Slack pathway) C4 photosythesis fixes HCO3- with pyruvate into malate, then transport malate to a second cell type for decarboxylation and refixation by RuBisCO Vein Vein Discovered in 1950’s 1 HCO3- CO2 Pyr 5 PEP PEP 2 Pyr RuBisCO OAA C4 photosynthesis is more efficient CO2 3 Pyr Mal Mal OAA 4 because it fixes HCO3- first (avoids O2) Bundle Sheath Mesophyll 11 C4 Photosynthesis Mesophyll Cell Rice Maize Bundle Sheath Cell Vein Vein C4 photosythesis depends on spatial separation of the C4 and C3 (RuBisCO) fixation reactions 1 HCO3- CO2 Pyr 5 PEP PEP 2 The only major C4 crop is maize Pyr RuBisCO OAA CO2 3 Pyr Mal Mal OAA 4 Bundle Sheath Mesophyll 12 6 1/20/20 CAM Photosynthesis CAM = Crassulacean Acid Metabolism CAM photosythesis depends on temporal separation of the C4 and C3 (RuBisCO) fixation reactions CAM plants store C4 acids (malate) in the vacuole for decarboxylation and refixation by RuBisCO in the day 13 CAM Photosynthesis CAM requires fine control of gene expression over the day-night cycle CAM uses an ‘inverted’ cycle of stomatal opening/closing to save water and concentrate CO2 in the leaf 'Inverted’ stomatal diel cycle 14 7 1/20/20 CAM Photosynthesis Like C4 photosynthesis, CAM has evolved multiple times across the angiosperms The only major CAM crop is pineapple 15 CO2/O2 limits the efficiency of the C3 cycle In C3 plants photorespiration accounts for ~30-50% of RuBisCO turnover CAM achieves much more savings in water use 16 8 1/20/20 Is CAM better for engineering? CAM will require engineering of approximately 35-40 gene targets with their promoters 17 Summary Dark reactions use NADPH and ATP with RuBisCO to fix CO2 and regenerate RuBP C3 photosythesis depends on RuBisCO selectivity between CO2 and O2 C4 and CAM photosynthesis get around the CO2/O2 limitation by introducing a ‘pre-fixation’ step to capture C as a 4-carbon acid C4 and CAM have a metabolic cost, but they are well-suited to growth under high light (extra energy) and reduced water availability 18 9 How the information carried in DNA is used to specify the structures of protein products. Lectures 8,9,10 DNA, RNA, Proteins Professor George Baillie ([email protected]) INSTITUTE OF CARDIOVASCULAR AND MEDICAL SCIENCES TWITTER: Baillie Lab@baillie_lab Biology 1B Semester 2, (23rd, 27th, 28th Jan 2025) Lecture 8 DNA: a store of biological information Lecture 9 RNA: how genes are expressed Lecture 10 : How cells make proteins Learning Objectives Lecture 8 (DNA) Describe evidence that DNA carries genetic information Show that DNA is an anti-parallel double-stranded polynucleotide in which base-pairing plays a crucial role Explain the Meselson-Stahl experiment to show semi-conservative replication of DNA Show how DNA polymerase and other proteins achieve DNA replication Explain that DNA replication is a very accurate process but that rare mistakes can lead to mutations Scientific inspiration. The origins of protein phosphorylation, Philip Cohen, 2002, NCB, 4, E127-E130 Carl and Gerty Cori 1947 (glycogen metabolism) Earl Sutherland 1971 (cAMP) Crebs and Fischer 1992 (PKA) Gilman and Rodbel 1994 (G proteins) Science. 2002 Oct 25;298(5594):834-6. Targeting of cyclic AMP Lefkowitz/Kobilka 2012 degradation to beta 2-adrenergic receptors by beta-arrestins. (G protein couled receptors) Baillie GS, Perry, S and Lefkowitz DNA carries genetic information Experiments of Mendel (1860s) on peas Miescher (1860s) had isolated an unusual (flower colour, seed shape and stem length acidic, phosphorus-containing, substance etc.) - led to the idea of "units of inheritance" "nuclein" (DNA) from pus cells (from (later termed genes). discarded surgical bandages). Morgan (1900s) studied Drosophila (fruit flies) - genes were located on chromosomes in the nucleus. He examined characteristics (1844-1895) (1822-1884) such as eye colour and wing size. (1866-1945) Chromosomes were known to contain DNA and proteins. Many suspected protein to be “inheritance factor” DNA carries genetic information Griffith (1928) - experiments on strains of Streptococcus pneumoniae, a bacterium that causes pneumonia in mice and other mammals 1879-1941 Transformation is a change in genotype & phenotype due to assimilation of external DNA by a cell…but at the time of Griffith the identity of the “inheritance factor” remained a mystery though widely thought to be protein. DNA carries genetic information Avery carried on Griffiths work and systematically destroyed lipids, carbohydrate, proteins and ribonucleic acid of virulent bacteria BUT transformation still occurred. Only when he used deoxyribonuclease to destroy DNA was transformation blocked. This was the first indication that DNA was the inheritance factor. Oswald T. Avery 1877-1937 DNA carries genetic information Other evidence came from studies of viruses which infect bacteria (phages). Only the DNA (32P label), not the protein ( 35S label) enters the bacterial cell. T2 Alfred Hershey This was final proof that DNA was genetic material, now race was on to determine how structure of DNA could account for its role in inheritance Martha Chase The composition of DNA He found (1947):- · The composition of DNA differed between species – molecular diversity amongst species · Within a species the DNA from all cells had Erwin Chargaff the same composition · In all cases, the percentages were G = C, and A = T (within experimental error) For all human cells, A = 30.9%, T = 29.4%, G = 19.9%, C = 19.8% The composition of DNA In 1947, DNA was known to be a polymer of nucleotides (base-sugar (deoxyribose)-phosphate). Though nothing known of its structure. The bases are of 4 types:- adenine, A cytosine, C guanine G thymine, T A and G are purines (2 fused rings) C and T are pyrimidines (1 ring) The sugar is the 5-carbon sugar 2´-deoxyribose Each nucleotide consists of a base (T,A,CorG), the sugar deoxyribose (blue) and a phosphate (yellow) The structure of DNA From X-ray diffraction experiments (Wilkins and Franklin, early 1950s), it was known that DNA was helical in shape. Rosalind Franklin 1920 - 1958 “Picture 51” 1952 This allowed Watson to deduce width of helix and spacing of nitrogenous bases along it. The structure of DNA Watson and Crick (1953) made the crucial step in 1953 - the idea of specific base pairing within a double helical structure. Thus an A on one strand pairs with a T on the other; a G on one strand with a C on the other. The pairing is due to hydrogen bonds. They published their ideas in a 700 word article in Nature, and were * awarded the Nobel Prize in 1962. Watson and Crick The structure of DNA-Features of the Watson and Crick model 1. Sugar-phosphate backbone 2. The strands run anti-parallel to each other (5’-3’ and 3’-5’) 3. 10 base pairs per turn of the double helix 4. The bases (non-polar hydrophobic) are on inside, the phosphate (polar, hydrophilic) are on outside. 5. Space filling models show a major and a minor groove in the double helix Replication of DNA The Watson-Crick model suggested a mechanism for copying the genetic information from one cell to its daughter cells. Each strand acts as the template for synthesis of a new stand according to the base-pairing rules. The two strands need to unwind and separate to expose the bases to allow the synthesis of new DNA to occur. Replication of DNA This type of mechanism is termed "semi- conservative", as opposed to "conservative" or "dispersive" models. Evidence for the semi-conservative model came from an elegant experiment carried out by Meselson and Stahl (1958). Stahl 1929- Meselson 1930- Replication of DNA The experiment relied on:- ❑Escherichia coli, a bacterium which could grow on simple sources of N and C ❑isotopes of N (15N and 14N) to distinguish between old and new DNA ❑density gradient centrifugation to separate the two types (15N-DNA is about 1% more dense than 14N-DNA) The results were only consistent with the semi- conservative model. DNA replication is elegantly simple… BUT the biochemistry is complex. * Replication of DNA Many proteins are involved in the replication of DNA Replication starts at specific origins of replication (one in prokaryotes, many in eukaryotes). Proteins recognise the DNA sequence and bind to it, opening up the double helix. In the replication bubble, there are Y-shaped replication forks, where the new strands are being elongated. Replication starts at multiple sites where the parental strands separate to form replication bubbles. The bubbles expand laterally as DNA replication proceeds in both directions Eventually the replication bubbles fuse and synthesis of the daughter strands is complete Replication of DNA The elongation is carried out by enzymes known as DNA polymerases. They use triphosphates (ATP, GTP etc.) as the sources of nucleotide units to add to the growing chains. A two- phosphate unit (pyrophosphate) is split out as the chain is extended by each nucleotide. Rate of elongation is 50 nucleotides a second in humans. Now over 10 different DNA polymerases identified in eukaryotes. Note that nucleotides can ONLY be added to the free 3’ end..NEVER the 5’end. Replication of DNA Because the strands are anti-parallel and the enzymes can extend chains in one direction only (5´ → 3´), the 2 strands cannot be both replicated continuously. We thus have a leading strand (continuous replication) and a lagging strand (discontinuous replication). * Replication of DNA The fragments of the lagging strand (Okazaki fragments) are typically 100-200 nucleotides in length and are later joined by an enzyme (DNA ligase). DNA polymerases can only extend a pre- existing strand - so how does the process start? The answer is that the cell starts by making a short primer of RNA (RiboNucleicAcid), about 10 nucleotides long, which is added to. This primer is later replaced by DNA. Replication of DNA is an extremely rapid and accurate process. Summary of important proteins in DNA replication Lecture 8 summary slide Familiarise yourself with the ground breaking discoveries of Mendel, Miesher, Morgan, Griffith, Avery, Hershey/Chase, Chargaff, Meselson/Stahl, Watson/Crick Know that the DNA polymerase mechanism on the leading/lagging strand during DNA replication is different. Remember the names and roles of other proteins in the process of DNA replication. Biology 1B Semester 2, (27th Jan Lecture 9) Lecture 8 DNA: a store of biological information Lecture 9 RNA: how genes are expressed Lecture 10 : How cells make proteins This molecule is a A. Nucleotide from DNA B. Nucleotide from RNA C. Amino acid D. Initiating tRNA The function of Single-strand binding protein is to: A. Elongate the Okazaki fragments B. Join the Okazaki fragments C. Stabilise single stranded DNA until it can be used as template D. Unwind the double helix at replication forks Which statement is true? A. Adenine and thymine are purines B. Guanine and cytosine are purines C. Adenine and guanine are purines D. Cytosine and thymine are purines The fact DNA carries genetic information was proven in part by the pioneering work of Hershey and Chase. They used the following observation to inform their experimental design: A. Bacteria do not contain DNA only RNA B. DNA and protein contains lots of sulphur C. DNA contains sulphur and protein contains phosphate D. DNA contains phosphate and protein contains sulphur Complimentary strands of DNA are held together by A. Strong covalent bonds B. Weak hydrogen bonds C. They are not bonded together D. Sticky mucus glue In the replication of DNA, both new strands cannot be replicated continuously as : A. chains extend in one direction only 5’ - 3’ B. chains extend in one direction only 3’ - 5 C. ’ D. chains extend both directions simultaneously blocking each other E. One new strands require proof reading before another is made. Repair of DNA Replication of DNA is an extremely rapid and accurate process. The DNA of Escherichia coli contains about 5 x 106 base pairs; this can be copied within about 30 min (3000 base pairs per second). The error rate is very low indeed - about 1 in 109 base pairs. This is achieved by a combination of the specific base-pairing (A with T, G with C), supplemented by proof-reading. All the DNA polymerases have proof-reading ability, and can detect and then remove any incorrectly added bases. DNA is a stable molecule, but can be damaged by high energy radiation (e.g. UV, X-ray) or by chemicals (mutagens). These agents greatly increase the rate of mutations and can lead to proteins of altered sequence and function. The cell has many (> 100) enzymes which can detect and repair damage to DNA. One example is nucleotide excision repair to remove a thymidine dimer formed by UV radiation. How much DNA is there in a cell? The sizes of genomes and their structures are now becoming elucidated from major sequencing projects. Human DNA contains 3 x 109 base pairs. If stretched out, this would amount to about 1 metre of DNA per cell! Mitochondrial DNA Although most DNA is packaged in chromosomes within nucleus, mitochondria also have a small amount of their own DNA (mtDNA: discovered in 1963). It is found near mitochondrial membrane and is double stranded and circular (like bacterial DNA). The mtDNA codes for proteins in respiritory chain (37 genes in total). Unlike nuclear DNA, mtDNA is passed from generations via maternal lineage (ie no mixing!). This is useful for the analysis of your mother’s family history…ethnicity, migration etc Mutations in mtDNA cause over 40 different diseases, usually centered around inability to generate enough energy. Learning Objectives Lecture 13 (RNA) Describe evidence for the link between genes and proteins (studies of mutants) Describe the copying action of RNA polymerases and explain the role of the DNA template during transcription Understand how RNA polymerases find the start of a gene and make the transcript of the template strand of DNA in the correct direction Describe how RNA transcripts in eukaryotic cells are processed into their mature functional forms and transported to their correct location in the cell The link between genes and proteins This was suggested by the work of Garrod (1909) who studied patterns of inherited diseases, such as albinism and alkaptonuria. He coined the term "inborn errors of metabolism". Linked symptoms of an inherited disease with a person’s inability to make a particular protein (enzyme).Genes dictate phenotypes. 1st to recognise Mendels laws in humans. Beadle and Tatum (1930s - 1940s) studied the link in detail using mutants (produced by X-rays) of the bread mould Neurospora crassa (good model organism as it grows on simple medium..makes its own amino acids) They studied mutations in the pathway in which Arginine (an amino acid) is synthesised. “One gene-one enzyme” hypothesis Nobel Prize 1958 :”Genes act by regulating definite chemical events” The link between genes and proteins Three classes each defective in a single gene. Each mutant was unable to carry out one step in pathway for synthesising argenine. Each mutant can grow ONLY if supplied with a compound made after the defective step. As DNA is not protein..genes DO NOT build protein..the link between the two is indirect. mRNA (messenger RiboNucleicAcid) is the intermediate. Transcription and Translation * Same language Different language RNA/DNA both polynucleotides(base,sugar,phosphate) Why are proteins translated but ribose instead of deoxyribose and Uracil instead of indirectly via mRNA and not thymine (U hydrogen bonds to A, just as T does in directly from DNA? DNA). RNA has no regular 3d structure but can form double helix (usually single strand). Transcription TRANSCRIPTION can be thought of as three distinct processes ❑INITIATION ❑ELONGATION ❑TERMINATION * Transcription : Initiation Initiation is at a site known as a promoter, containing a particular sequence of bases. In prokaryotes, the RNA polymerase itself recognises and binds to the promoter site. In eukaryotes, a set of proteins (transcription factors) are involved in the binding of RNA polymerase to the promoter (TATA box). Transcription :Elongation Elongation proceeds by local unwinding of 1- 2 turns of the DNA and addition of nucleotide units (about 60 per second). The DNA rewinds as the RNA polymerase proceeds along the template strand. Many RNA polymerase molecules can be transcribing a particular gene simultaneously. This allows large amounts of protein to be made. * Transcription :Termination TYPES OF RNA Termination of transcription is not as well There are several different types of RNA in a cell. The 3 main ones in prokaryotes and understood. It appears to be signalled by the eukaryotes are:- sequence of the RNA transcript itself. In eukaryotes this sequence is AAUAAA mRNA (messenger RNA) (polyadenylation signal). rRNA (ribosomal RNA) tRNA (transfer RNA) small nuclear RNA After this terminator sequence has been transcribed, proteins bind the growing The roles of rRNA and tRNA will be dealt transcript and cut it free from the with in the next lecture. polymerase, the transcript is released and the In prokaryotes, all the types of RNA are RNA polymerase detaches from the DNA. made by a single RNA polymerase. RNA processing mRNA processing In eukaryotes, primary RNA transcript is extensively modified before it leaves the nucleus. A cap (modified form of G) is added to the 5´-end and a polyA (50-200 nucleotides) tail is added to the 3´-end. These alterations · protect the mRNA from degradation · aid export from nucleus · help the mRNA to anchor to the ribosomes NOT TRANSLATED PROTEIN NOT TRANSLATED INTO PROTEIN INTO PROTEIN mRNA splicing After capping and tailing, the mRNA is spliced to remove non-coding regions (termed introns) so as to leave the coding regions (exons). The splicing is carried out by particles known as small nuclear ribonucleoproteins (snRNPs). These consist of small nuclear RNA (about 150 nucleotides long) and various proteins. Several snRNPs and other proteins form a spliceosome complex. The snRNPs seem to play a catalytic as well as a structural role (ribozymes). mRNA splicing NEED fig 17.10 Surprisingly, non-coding sequences are interspersed between coding sequences in pre-mRNA. Short nucleotide sequences at end of introns mark the “splice sites” for recognition by snRNPs. Often more untranslated pre-mRNA sequence (5’ cap, 5’ UTR, introns, 3’ UTR and poly A tail) than translated (exons). mRNA splicing The splicing process helps to increase the range of possible protein products from a given gene (alternate mRNA splicing). * Alternative splicing of RNA leads to male and female Drosophila, and switches between classes of certain antibodies, for example. An exon may correspond to a distinct folded unit (domain) of a protein with a particular function. The splicing process may assist the creation of new modular proteins with novel activities. The final transcript which leaves the nucleus is known as the mature mRNA. mRNA splicing Which comes first? A. Translation B. Transcription C. RNA processing D. Entry of DNA to nucleus Translation is the process where: A. RNA is spliced B. The genetic information in DNA is copied to mRNA C. The genetic information in mRNA is converted to polypeptide chains on ribosome D. The RNA transcript is elongated Transcription is the process where: A. Proteins are made using tRNA B. The genetic information in DNA is copied to mRNA C. DNA is replicated D. RNA is processed before leaving the nucleus This enzyme is called: A. Topoisomerase B. RNA polymerase C. Transcription factor D. Ribosome Which statement is true? A. The RNA transcript is a double helix B. The RNA transcript is extensively modified before leaving the nucleus C. All of the RNA transcript is translated into protein D. RNA splicing is responsible for leaky cells The orange piece of RNA in this diagram is known as A. an Intron B. an Exon C. snRNPs D. Mature mRNA Lecture 9 summary slide ❑Familiarise yourself with the work of Garrod (inborn errors of metabolism) and Beadle/Tatum (one gene, one enzyme) ❑Know about the three stages of transcription (initiation, elongation and termination) ❑Learn how Eukaryotic cells modify RNA (RNA processing) ❑Know about the process of RNA splicing (snRNPs, introns and exons) TOMORROW : TRANSLATION Biology 1B Semester 2, (28th Jan) Lecture 10 Lecture 8 DNA: a store of biological information Lecture 9 RNA: how genes are expressed Lecture 10 : How cells make proteins There are differences between the structures of DNA and RNA. Which one of these statements is false? A. DNA contains deoxyribose sugars, mRNA contains ribose sugars B. DNA uses thymine, mRNA uses uracil C. DNA has a double helix, mRNA is single stranded D. DNA and mRNA always reside in the nucleus of cells RNA is extensively modified before leaving the nucleus. Which one of these statements is NOT true. A. mRNA processing helps protect it from degradation B. mRNA processing helps it exit the nucleus C. mRNA processing helps to anchor it to ribosomes D. mRNA processing helps the spicing process by attracting snRNPs Ribosomes are the site of A. Translation B. Transcription C. RNA processing D. Splicing Learning Objectives Lecture 14 (Protein) Learning objectives Explain that cells need to make polypeptides of defined length and sequence Describe the importance of base-pairing in ensuring the accuracy of protein synthesis Discuss the machinery (ribosome), raw materials (aminoacyl- tRNAs) and instruction tape (mRNA) for protein synthesis (transl