biology 1.2.PDF
Document Details
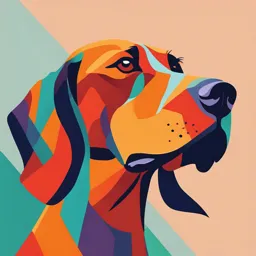
Uploaded by ModestPraseodymium
Related
- PCSII Depression/Anxiety/Strong Emotions 2024 Document
- A Concise History of the World: A New World of Connections (1500-1800)
- Human Bio Test PDF
- University of Santo Tomas Pre-Laboratory Discussion of LA No. 1 PDF
- Vertebrate Pest Management PDF
- Lg 5 International Environmental Laws, Treaties, Protocols, and Conventions
Full Transcript
Chapter 1: DNA $tructure, $ynthesis, and Repair Lesson 1.2 DNA RePlication Foracompleteandaccuratecopyofgeneticinformation(DNA)tobepassedtodaughterce|lsduringcell phase division, DNA must first be copied lrepticateo;. DNA replication tak...
Chapter 1: DNA $tructure, $ynthesis, and Repair Lesson 1.2 DNA RePlication Foracompleteandaccuratecopyofgeneticinformation(DNA)tobepassedtodaughterce|lsduringcell phase division, DNA must first be copied lrepticateo;. DNA replication takes place during the cell cycle process of DNA replication' as well as special known as S phase. This lesson expands on in" mechanisms for beginning and ending DNA replication' 1.2.01 Semiconservative Repllcatlon strand is rne ,epricat o*o is semiconservative, which me.ans tha.teach new (ie' daughter) the ". "i synthesized using one of the originar (parent or parental) strands as a temprate. During replication, and each parent strand is used as a template parent strands are separated from ealh other (denatured), (Figure 1'B)' When replication is complete' each to synthesize a new, complementary oaughier'strano (the template) and one newly synthesized daughter new double helix is composed of one parent strand strand. 3'. Parent strand 5' 3' 5', Figure 1.8 Semiconservative DNA replication 1.2.02 Origin of RePlication called origins of replication' which are specific DNA replication begins at sites within the DNA replicati6n attach to the origin of replication' which sequences of nucteotider. Enryt", that initiate a t*plication L:ul-rble' Replication proceeds in both tiiggers separation of the strands and formation of directions from the origin. with a single origin of replication' and Prokaryotic organisms generally have circular chrgmoson:es chromosome is copied' In eukaryotic organisms' replication occurs in Oo[h directions untilthe entire so of origins of replication, which open simultaneously chromosomes are linear and may have hundreds I Chapter 1: DNA $tructure, $ynthesis, and Repair replication can occur more quickly. As in prokaryotes, replication in eukaryotes proceeds in both directions from each origin (Figure 1.9). Eukaryotee Ori Orl Ori I I I I I I I I I i V Y ft#H$s&$&fi$fiH$$fi$fr*H$$$ \$r" Ori s origin of re$ication. Figure 1.9 Origins of replication in prokaryotes versus eukaryotes. 1.2.03 Mechanism of RePlication The mechanism of DNA replication is very similar in prokaryotes and eukaryotes. For simplicity, the prokaryotic model of DNA replication is discussed in this concept. At the beginning of DNA replication, the enzyme helicase unwinds the parent DNA helix at the origin of replication, and single-stranded DNA-binding proteins hold the two strands apart (Figure 1.10)' This creates a replication bubble and two replication forks. Synthesis of the daughter strands proceeds from the forks in both directions from each parent strand, lengthening the replication bubble as DNA synthesis continues. Chapter 1: DNA Structure, Synth*sis, and Repair Single-stranded DNA-binding protein 5', 3', '% "",:, t," Helicase "l-irii, \irii:r" Figure 1.10 Formation of the replication fork. As helicase unwinds DNA at the replication fork, the DNA ahead of the replication fork becomes ovenvound (negatively supercoiled). Topoisomerase reduces the strain caused by iiNA stri:*rtcilin1l in front of the replication fork by transiently cleaving one DNA strand' Because all DNA polytnerases can synthesize DNA only in the 5') 3'direction, one daughter strand is synthesized continuously toward the replication fork (leading strand). The other strand must be synthesized discontinuously in, a direction away from the replication fork (lagging strand), with new segments being added as mor"e DNA is unwound, as shown in Figure 1.'11. Primase I Single-stranded DNA-binding protein Leading strand Topoisornerase *{ 5' 4*r ,.i" ?'" lfr fork 3' -Jk?-ePrication Dlr'lA polymerase I Helicase DNA polymerase lll DNA ligase Lagging strand Figure 1.11 Synthesis of DNA from the leading strand' The initiation of DNA synthesis requires the synthesis of an RNA primer on both the leading and lagging strands because all DNA polymerase enzymes are able to attach new nucleotides only to an existing strand. This short RNA primer is synthesized by the enzyme primase in the 5' ) 3'direction. 11 Chapter 1: DNA $tructure, $ynthesis, and Repair Once the RNA primer is in place, DNA polymerase lll attaches uncoupled deoxynucleotide triphosphates (dNTPs) to the growing DNA strand. Each dNTP is composed of a nitrogenous base (eg, A, G, C, T) and three phosphate groups. Based on the complementary A-T/G-C base pairing rules, a free dNTP enters the catalytic site of DNA polymerase lll and forms hydrogen bonds with a complementary nucleotide on the parent (template) DNA strand. The 3'OH from the last nucleotide of the growing strand "attacks" the 5' PO+- group of the incoming dNTP. A pyrophosphate is released from the dNTP, and the nucleotide is attached to the growing strand via a condensation reaction (Figure 1.12). Condensation is an exergonic prncess, and the energy released from this reaction is used to form a covalent p":hosphorliester bond between the growing strand and the incoming dNTP. New strand 5' 3', 5', 3' \- L) ,ry fr l 6ndensation O-_l Phosohodiester reaction o=b-o-V Y\ OH bonds rl 3' s o-J 5', 9Hz I r./ I r* ?- ?- ?' -o-fi-o;fi*o;fi-o*cH2 5' c o- I V o- OH 3', 5', o / -o*[*o*[*o- tl o o 3'OH /- oCI lncomin$ dNTP Pyrophosphate Figure 1.12 Coupling of free nucleotides during DNA replication. When DNA synthesis is complete, DNA polymerase I removes RNA primers and replaces them with DNA nucleotides, and DNA ligase catalyzes the formation of phosphodiester bonds to join all DNA fragments. The replication of the lagging strand is problematic due to the antiparallel orientation of the two DNA strands (Figure 1.13). Because DNA polymerase enzymes are able to synthesize DNA only in the 5' ) 3' direction, new RNA primers must be added periodically as the replication fork advances. As a result, replication on the lagging strand is discontinuous, and short fragments of newly synthesized DNA called Okazaki fragments are formed. RNA primers must be removed from each Okazakifragment and replaced with DNA before the fragments can be joined by DNA ligase. 12 *ha6:t*r l: llf*A $tructur*, $ynth**is, and R*pair DNA polymerase lll Primase i $ingle-stranded I.l DNA-hinding prntein L*ading slrand 5', 3', Lagging strand Okazaki fragments Primase Figure 1.13 Synthesis of DNA from the lagging strand. The rate of DNA replication in prokaryotes is -1,000 nucleotides per second, but in eukaryotes it is much slower, at -50-100 nucleotides per second. While synthesis of the leading and lagging strands occurs simultaneously, synthesis of the lagging strand occurs at a slightly slower rate, as the template strand must be continuously revealed atthe replication forkfor continued replication of the lagging strand. The various proteins involved in DNA replication are summarized in Table 1.'1. Table 1.1 Proteins involved in DNA replication. Protein Function Helicase Unwinds DNA double helix to form reolication fork Single-stranded DNA- Prevents DNA double helix from reannealinq binding protein Topoisomerase Relieves DNA supercoiling in front of the replication fork Primase Synthesizes RNA primer DNA polymerase lll Performs 5') 3'DNA synthesis DNA polymerase I Replaces RNA primers with DNA DNA ligase Joins DNA fragments after replacement of RNA primers 1.2.04 Replication at the Ends of Linear Chromosomes DNA replication machinery requires a free 3'end to incorporate a new dNTP into the daughter strand, so replicating the ends of a linear chromosome poses a particular problem. When the 3'end of the template is too short to engage with DNA polymerase, DNA synthesis cannot continue, and chromosome ends are incompletely replicated. IJ Chapter 1: DNA $tructure, Synthesis, and Repair Because these uneven, overhanging ends cannot be copied, the chromosome becomes shorter with each successive round of replication (Figure 1.14). Shortening of chromosomes is notan issue tn prokaryotes, since prokaryotic chromosomes are generally circular; however, shortening of chromosomes is problematic in eukaryotes, because eukaryotes have linear chromosomes. Leading strand DNA polymerase (replicated continuously) 3' :1lt '-" r x* r r r r r t*. r r r r_ i* r r* r r i* i* r_ r* r_ rli*.i.* i__ ,{ i i i i i i i I I 1 t I i I I I I 't 'l I i rl I [ ] $ i Okazakifragment Primer ,"".'J r\ "t *flr :r1'rTiT:f :ri*'r''r"r r{'r r* r' r r' flrtr I 1 }i ll i li I ill I I ri il i i 1l I Lagging strand Not enough room for (replicated in fragments).l DNA polymerase to bind, final fragment not produced 5' "* t* r r* r* r r t* r r* r r r r* i* t* tr- f- f* i* r r r r r- r r r r r- i* r* r- r i- *TT*{:1[1T*lirrf T"l*n-rrr*f'rr r, t*f1rfi'r 1rTYrruTilflts*,'fili 111 rttl;i tlltlli lll ii ll iillllli 3', Removal of primers ,1,.,' * f* t* t* l* t* r i-- f* l* i* r r r l* r i*' l* {* r i* i* f* ri* T f* r rr r r r r r 5' $hortened chromosome end 'tri'l* *]1": r I-*r {* r r r r r r r r r r r r r"* l-1 t* l* lT:rfl l-T:.- Ll*l-:l* L: I j j*l 1-:JJ-: i 1l*. J-Ll* i l*li* ;*|: l l* L": t' Figure 1.14 Shortening of linear chromosome ends during replication. The progressive shortening of chromosomes is addressed in eukaryotes by the addition of noncoding DNA sequences called telomeres to the ends of chromosomes (see Figure 1.15). Telomeres consist of copies of a six-nucleotide sequence (5'-TTAGGG-3') repeated 100 to 1 ,000 times at the end of each chromosome. Telomeres do not contain coding information, so the portions of the telomeres lost with each replication cycle do not result in a loss of genetic information. Chapter 1: DNA Structure, Synthesis, and Repair Gene-containing portion of chromosome Telomeric repeat 3', 5' Telomere Figure 1.15 Telomere sequences at the end of a linear chromosome. Telomeres function to protect the chromosome from loss of important DNA sequences as the chromosome becomes increasingly shorter with each round of replication. The protection of chromosome ends via looping and telomere-specific proteins also prevents activation of DNA repair mechanisms (discussed in Lesson 1.3), because overhanging ends may trigger a response that could lead to cell cycle arrest or cell death. Normal shortening of chromosomes may protect organisr)s from cancer by limiting the number of somatic cell divisions that can occur. The successive shortening of telomeres has also been linked with cellular and organism aging, since this shortening results in a finite number of possible cell divisions. In cells that must retain the potential to divide indefinitely (ie, stem cells and germ cells), and in some cancer cells, the enzyme telomerase is expressed and functions to replenish shortened telomere sequences on the ends of chromosomes (see Concepts 5.4.04 and 10.5.02). 15