BIOL 217 W24 Topic 10 (Fall 2024) PDF
Document Details
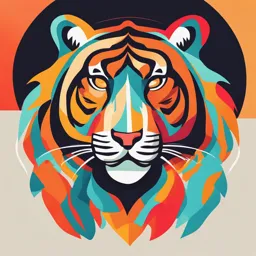
Uploaded by SpeedyObsidian9531
Red Deer Polytechnic
2024
Tags
Summary
This document is a set of lecture notes from a topic on DNA structure and replication. It covers key concepts including historical experiments, base pairing rules, structural models, and different replication mechanisms.
Full Transcript
BIOL 217 Topic 10 Fall 2024 Learning Objectives – Topic 10 DNA Structure and Replication – Chapter 16 Understand how key experiments lead to the understanding that DNA was the genetic material Explain Chargaff’s rules and how they helped Watson and Crick build their model for DNA Underst...
BIOL 217 Topic 10 Fall 2024 Learning Objectives – Topic 10 DNA Structure and Replication – Chapter 16 Understand how key experiments lead to the understanding that DNA was the genetic material Explain Chargaff’s rules and how they helped Watson and Crick build their model for DNA Understand how Watson and Crick used Franklin’s research to build their model for DNA Describe the important structures of DNA double helix: antiparallel phosphate backbone, nucleotide interactions, complementary base pairing Understand how DNA replicates, being able to distinguish between conservative, semi conservative and dispersive replication models 2 Learning Objectives – Topic 10 DNA Structure and Replication – Chapter 16 Compare prokaryotic and eukaryotic DNA replication, origins of replication Describe the molecular mechanism for DNA replication, including: replication fork, helicase, single stranded binding proteins, topoisomerase, DNA polymerase III and I, RNA primer, primase, DNA ligase, leading strand, synthesis in 5’ > 3’ direction. Explain how telomeres protect the ends of DNA Explain how DNA is packaged within a cell 3 DNA Functions Hereditary information is encoded in DNA and reproduced in all cells of the body DNA is copied during DNA replication Cells can repair their DNA 4 Figure 16.1 DNA is the genetic material Molecules of inheritance: DNA In 1953, James Watson and Francis Crick introduced double helix model for DNA Prior to this, identifying molecules of inheritance was a major challenge 5 Figure Page 334 Series of famous experiments showing that DNA is the genetic material Genetic Material: DNA or Protein? Frederick Griffith in 1928 Alfred Hershey and Martha Chase in 1952 Erwin Chargaff in 1950 6 Griffith’s Experiment: Transfer of Material – DNA? Two strains of bacteria, one pathogenic and one harmless Transformation: change due to the introduction of foreign DNA People still thought protein was genetic material – a lot of heterogeneity and specificity of function 7 Figure 16.2 Hershey and Chase’s Experiment More evidence for DNA as genetic material Studied viruses that infect bacteria, known as bacteriophage (phage) A virus is DNA or RNA enclosed by a protective protein capsid 8 Figure 16.3 Additional Evidence That DNA Is the Genetic Material DNA is a polymer of nucleotides: Consisting of: Nitrogenous base Sugar Phosphate group The nitrogenous bases can be adenine (A), thymine (T), guanine (G), or cytosine (C) Figure 5.23 9 Figure 16.5 Chargaff’s Rules In 1950, Erwin Chargaff reported that DNA composition varies from one species to the next Two findings became known as Chargaff’s rules: The base composition of DNA varies between species In any species, the number of A = T, and the number of G = C 10 PublicDomainPictures (2013). https://cdn.pixabay.com/photo/2013/07/18/10/59/dna-163710_1280.jpg Building a Structural Model of DNA Maurice Wilkins and Rosalind Franklin Using X-ray crystallography to study molecular structure Franklin produced a picture of the DNA molecule using this technique Franklin’s X-ray crystallographic images of DNA allowed Watson to deduce that DNA was helical 11 Figure 16.6 Building a Structural Model of DNA Watson used the X-ray images to deduce: Helical structure Width of the helix Spacing of the nitrogenous bases The pattern in the photo suggested: DNA molecule was made up of two strands, forming a double helix 12 Figure Page 340 Building a Structural Model of DNA Watson and Crick built models of a double helix to conform to the X-rays and chemistry of DNA Franklin had concluded that there were two outer sugar- phosphate backbones, with the nitrogenous bases paired in the molecule’s interior Watson built a model in which the backbones were antiparallel Pairing a purine (A or G) with a pyrimidine (C or T) resulted in a uniform width consistent with the X-ray data 13 Figure Page 339 Building a Structural Model of DNA At first, Watson and Crick thought the bases paired like with like (A with A, and so on), but these pairings did not result in a uniform width A paired only with T, G paired only with C The Watson-Crick model explains Chargaff’s rules: in any organism the amount of A = T, and the amount of G = C 14 Figure 16.8 DNA Double Helix Right-Handed Helix Antiparallel Sugar Phosphate backbone Nitrogenous bases Major / minor groove Hydrogen bonds 15 Figure Page 339 Portrayals of DNA 16 Figure Page 339 Comparing DNA and RNA Structure 17 DNA replication and Repair The relationship between structure and function is evident in the double helix Watson and Crick noted that the specific base pairing suggested a possible copying mechanism for genetic material 18 Figure Page 339 The Basic Principle: Base Pairing to a Template Strand The two strands of DNA are complementary Each strand acts as a template for a new strand in replication In DNA replication, the parent molecule unwinds, and two new daughter strands are built based on base-pairing rules 19 Figure 16.9 Competing Models of DNA Replication Conservative model - the two parent strands rejoin Dispersive model - each strand is a mix of old and new Watson and Crick’s Semiconservative model of replication: When a double helix replicates, each daughter molecule will have one old strand (“conserved” from the parent molecule) and one newly made strand 20 Figure 16.10 Prokaryotic DNA Replication The copying of DNA is rapid and accurate Numerous proteins and enzymes contribute to DNA replication Replication begins at sites called origins of replication (ori) Where the two DNA strands are separated, opens up a replication “bubble” 21 Figure 16.12 Eukaryotic DNA Replication A eukaryotic chromosome may have hundreds or even thousands of origins of replication Replication proceeds in both directions from each origin, until the entire molecule is copied 22 Figure 16.12 Origins of Replication 23 Replication Bubble Replication fork: at the end of each replication bubble Y-shaped region where new DNA strands are elongating Paired (one on either side of replication bubble) Helicase: enzyme that untwist the double helix at the replication forks 24 Figure 16.13 Replication Bubble Single-strand binding proteins: bind to and stabilize single- stranded DNA Topoisomerase: relieves the strain of twisting of the double helix by breaking, swiveling, and rejoining DNA strands 25 Figure 16.13 Synthesizing a new DNA Strand DNA Polymerases: enzymes that catalyze synthesis of new DNA at replication fork Require a primer (so they can add nucleotides) and a DNA template strand 26 Figure 16.15 Leading Strand Synthesis Along one template strand of DNA, the DNA polymerase synthesizes a leading strand continuously, moving toward the replication fork (5’ –> 3’) Requires: RNA primer, primase, DNA Pol III, template DNA, helicase, ssBPs 27 Figure 16.15 Leading Strand Animation 28 DNA Replication Overview 29 DNA Replication 30 Figure 16.17 31 Proofreading and Repairing DNA DNA polymerases proofread newly made DNA, replacing any incorrect nucleotides DNA can be damaged by: Exposure to harmful chemical or physical agents such as cigarette smoke, X-rays, or UV rays Spontaneous changes In mismatch repair, repair enzymes correct errors in base pairing In nucleotide excision repair, a nuclease cuts out and replaces damaged stretches of DNA 32 Figure 16.19 If errors are not repaired The error rate after proofreading and repair is low but not zero Sequence changes may become permanent and can be passed on to the next generation Mutations are: The source of the genetic variation upon which natural selection operates Ultimately responsible for new species Some mutations can be deleterious 33 Katzenfee50 (2018). White fallow deer. https://cdn.pixabay.com/photo/2018/03/01/23/56/white-fallow-deer-3192013__480.jpg Telomeres and telomerase If chromosomes of germ cells became shorter in every cell cycle: Essential genes would eventually be missing from the gametes they produce An enzyme called telomerase catalyzes the lengthening of telomeres in germ cells The shortening of telomeres might protect cells from cancerous growth by limiting the number of cell divisions There is evidence of telomerase activity in cancer cells, which may allow cancer cells to persist 34 Figure 16.21 Chromosomal Packaging DNA is combined with proteins in a complex known as chromatin Double helix DNA sequence Histones: Proteins that help with first level of DNA packing Histone tails involved in gene expression regulation Nucleosome: DNA wrapped around histones Beads on a string, 10 nm fiber 35 Figure 16.23 Chromosomal Packaging 30 nm fiber: Continued condensation of DNA Looped Domains: 30 nm fiber loops, making a 300 nm fiber Metaphase chromosome: Compacted chromatin we see as metaphase chromosome 36 Figure 16.23 Chromosome location in cells Interphase chromosomes occupy specific restricted regions in the nucleus The fibers of different chromosomes do not become entangled Homologous chromosomes are not next to each other 37 Figure 16.24 Chromosome location in cells Most chromatin is loosely packed in the nucleus during interphase and condenses prior to mitosis Euchromatin: Loosely packed chromatin, more accessible for gene expression Heterochromatin: Highly condensed chromatin, less accessible for gene expression Dense packing of the heterochromatin makes it difficult for the cell to express genetic information coded in these regions 38 Figure 16.24