BIOL2010 Translation Lecture 2 2024 PDF
Document Details
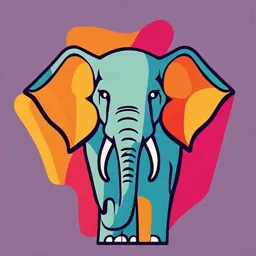
Uploaded by JoyousHawkSEye599
Southampton General Hospital
2024
Kif Liakath-Ali
Tags
Related
- Translation in Protein Synthesis PDF
- Molecular Biology and Cytogenetics - Translation (PDF)
- Molecular Biology and Cytogenetics - Translation PDF
- Molecular Biology and Cytogenetics - Translation PDF
- Molecular Biology and Cytogenetics Translation PDF
- Chapter 4 Translation - Fundamentals of Cell Biology PDF
Summary
These lecture notes for BIOL2010, The Flow of Genetic Information, cover lecture 2 on translation in 2024. The material specifically details the structure and function of ribosomes and the processes involved in protein synthesis. The notes also include discussion on translation errors and quality control.
Full Transcript
Translation – Lecture 2 Module: BIOL2010 The Flow of Genetic Information 13/12/2024 Kif Liakath-Ali PhD www.splicelab.co.uk Translation Lectures Lecture 1 – 6/12/2024 Lecture 2 – 13/12/2024 - Introduction...
Translation – Lecture 2 Module: BIOL2010 The Flow of Genetic Information 13/12/2024 Kif Liakath-Ali PhD www.splicelab.co.uk Translation Lectures Lecture 1 – 6/12/2024 Lecture 2 – 13/12/2024 - Introduction - Ribosomes - Steps, mechanisms - - 10 mins Break - Building blocks: tRNA and genetic - mRNA surveillance code - Translational control Lecture 3 - Review of Translation (KLA) 08/01/2025 (10-11 am, B100/1001 L/T A) - Overview of exam (DAD) 08/10/2025 (B32/1015) Learning outcome Ribosome Illustrate how ribosomes assemble at the start codon and the role of initiation factors in prokaryotic and eukaryotic translation. Explain peptide bond formation and the role of elongation factors (e.g., EF-Tu in prokaryotes, eEF1A in eukaryotes) in codon-anticodon pairing and proofreading. Errors and Quality Control > Explain how translation errors (e.g., mistranslation, frameshifts) are minimized by proofreading mechanisms. > Briefly introduce ribosome quality control pathways (e.g., recognition of stalled ribosomes). Applications and Implications Relate translation to real-world examples, such as the effects of antibiotics targeting ribosomes. What do we need for protein synthesis? A template that has sequence (clue/code) on where to start decoding A supply of building blocks Something to assemble the building blocks into chains A way to supply the correct building block at the appropriate time Rules to define HOW to decode (including where to start and stop) Energy to drive the process What do we need for protein synthesis? Ribosomes are the synthetic machinery (rRNA and proteins) The template is a mature mRNA (capped and tailed in eukaryotes) All 20 amino acids are activated as aminoacyl-tRNAs Requires a large number of other enzymes/proteins (factors) and energy in the form of GTP and ATP Adding each amino acid ‘costs’ 4x ATP Ribosomes, the cellular factories that manufacture proteins Rickgaur et al., Dec 2, 2024 – Mol. Cell Ribosomes synthesise proteins ribosomes on the Endoplasmic Reticulum making proteins that will be secreted or have roles in membranes A single cell typically contains millions of ribosomes making 10 million peptide bonds per second per cell (in eukaryotes) The components of ribosomes Ribosomes have Mr of over 2.5 million Da In eukaryotes, ribosomes assemble in the nucleolus Bacterial Eukaryotic 70S = 30S+50S 80S = 40S+60S 34 proteins 21 proteins >49 proteins >33 proteins Similar to organellar ribosomes The structure of the bacterial ribosome How does the ribosome catalyse peptide bond Haloarcula marismortui formation? Nobel Prize for Chemistry 2009 Venkatraman Tom Steitz Ada Yonath Ramakrishnan Key features of the bacterial (E. coli) ribosomal subunits 30S subunit – 16S rRNA + proteins S1- S19 Contains the “decoding centre” (DC) Helix 44 of 16S rRNA forms the A and P tRNA binding sites 3’ of 16S rRNA complements the Shine-Dalgarno Key features of the bacterial (E. coli) ribosomal subunits 50S subunit - 23S and 5S rRNAs + proteins L1– L31 23S rRNA forms 6 domains (I-VI) Contains the peptidyl transferase centre (PTC) Contains the peptide exit tunnel The ribosome catalyses peptide bond formation (aa)n-tRNA + aa-tRNA’ (aa)n+1-tRNA’ + tRNA Peptidyl Incoming Extended ‘Spent’ tRNA -tRNA amino acyl-tRNA peptidyl-tRNA [deacylated] Originally thought that rRNA was just a scaffold for the proteins However, biochemical and genetic data pointed to a key role for domain V of 23S rRNA in the peptidyl-transferase function Final confirmation that the ribosome is a ribozyme came from the crystal structure of the 50S subunit from the archaebacterium Haloarcula marismortui Ribozyme = an RNA molecule capable of acting as an enzyme Evidence that the Ribosome is a Ribozyme Peptidyl- transferase centre (PTC) No ribosomal proteins located in the PTC (peptidyl-transferase center) – nearest ones are 15-18Å away – too far to take part Ribosomes that are largely depleted of protein still have peptidyl-transferase activity Sites of the ribosome Remember that ribosome has three sites: A (occupied by Aminoacyl-tRNA) P (occupied by Peptidyl-tRNA) E (occupied by deacylated tRNA, so Exit site) To create a peptide bond, need NH of incoming amino acid (attached to tRNA) to attack CO of the growing polypeptide, which is still attached to tRNA. Ends up with extended polypeptide Normal peptide bond formation P site A site Nucleophili c attack Need to break ester bond between amino acid and tRNA Nucleophilic attack by amino group of the incoming aminoacylated tRNA The Ribosome is a Ribozyme Conserved RNA secondary structure These are the 3’ A bases from around peptidyl-transferase centre the tRNAs, not the ribosome (highlighted bases are masked/disrupted by antibiotics) Crystal structure of a transition state analog revealed several ribosomal (nucleotide) residues that may be involved Only A2486 shown for simplicity …or is it just a matchmaker? Mutation of 2’-OH of A76 of peptidyl- tRNA decreases the rate of peptide bond formation ~million-fold But mutagenesis of rRNA bases in E. coli e.g. A2451 (≡A2486), U2506, U2585, A2602, does NOT affect peptidyl- transferase reaction Therefore ribosome helps to position substrates and water molecules which aids proton transfer and stabilisation of intermediates Ribosome does not work by chemical catalysis, but instead decreases activation energy needed for peptide bond formation The Steps of Translation Elongation (EFs/eEFs) ensures correct amino acid is added sequentially to the growing protein chain by base pairing of transfer RNA (tRNA) with mRNA decodes 10 – 40 aa per sec with only 1 in 10,000 error rate already seen that accuracy of charging tRNAs with the correct amino acid is key to this, but elongation factors do check fidelity of codon:anticodon pairings mRNA AAAA AUG UAG UAA UGA Protein synthesis in bacteria - Elongation The next aminoacyl-tRNA binds to A-site aided by elongation factor EFTuGTP If codon:anticodon is correct, EFTuGTP is hydrolysed to EFTuGDP EFTuGDP is then released and recycled to EFTuGTP by EFTs EFTs is a guanine nucleotide-exchange factor (GEF) EFTuGDP + GTP EFTuGTP + GDP Peptide bond -CO-NH- formation occurs in the PTC “Translocation” then occurs: peptidyl-tRNA moves to the P site; the spent tRNA moves to the E-site and exits the ribosome This requires EFGGTP which is hydrolysed to EFGGDP The next aminoacyl-tRNA binds at A-site; cycle is repeated “Costs” 2x GTP molecules The mechanism of elongation Peptide Selection tRNA 3’- bond of correct ends formed AA-tRNA move E & P sites P E A Peptidyl EFTu transferase GTP GDP + Pi Translocation occurs in two steps – Translocation EFG first the 3’-ends of the tRNAs, and then the codon:anticodon pairs The mechanism of elongation Peptide Selection tRNA 3’- bond of correct ends formed AA-tRNA move E & P sites P E A Peptidyl EFTu transferase GTP GDP + Pi Translocation EFG Translocation occurs in two steps – first the 3’-ends of the tRNAs, and then the codon:anticodon pairs tRNA is always base-paired with mRNA: prevents frameshifting, ensures accuracy, shows why code is read triplet by triplet Spent tRNA leaves E- Elongation in eukaryotes eEF1A equivalent to EFTu eEF1B = EFTs (GEF for recycling eEF1A) eEF2 = EFG Phosphorylation of eEF2 (in response to rise in AMP, rise in Ca2+) reduces elongation rate Schneider-Posch et al (2010) Nature Chem Biol 6: 189– 198 Nascent protein exits through a “tunnel” TF (trigger factor) is a chaperone: coordinates folding/elongation rates Bacterial ribosome exit tunnel 80-100 Å long ~30-40 residues Nascent chain passes through the ribosome exit tunnel Protects new chain from inappropriate interactions Allows it to sample multiple conformations Proteins fold during biosynthesis The sequence of amino acids (primary structure) of a protein determines its folding and its final shape The N-terminal portion will start to fold before the C- terminal region is completed Many proteins are initially synthesised as pre- or pro-proteins and are post- translationally modified (phosphorylation, glycosylation etc) Many proteins bind coenzymes (NAD), cofactors (Zn2+) or prosthetic groups (haem) Protein synthesis in bacteria - Termination Elongation continues until a termination codon occupies A-site Ribosome fails to find cognate AA-tRNA Release factors RF1 or RF2 and RF3 bind (RF1/2 mimic tRNA) RF1, UAA/UAG; RF2, UAA/UGA The final aminoacyl-tRNA ester link is hydrolysed by reaction with H2O and the peptide is released from the ribosome The mRNA and the ribosomal subunits separate from each other RRF recycles the subunits Eukaryotic differences: eRF1 for all three stop codons, eRF3 is the GTPase Several recycling factors ABCE1 is key one Antibiotic binding to the bacterial ribosome Although prokaryotic and eukaryotic ribosomes are similar, most antibiotics bind specifically to bacterial ribosomes because of small structural differences Chloramphenicol - blocks peptidyl transferase (to treat eye infection) Erythromycin - blocks elongation by binding to the 23S rRNA tunnel (skin, eye infections) Tetracycline - prevents amino acyl tRNA binding (acne, cholera, plague etc Action of aminoglycoside antibiotics From: Ramakrishnan (2008) Biochem. Soc. Trans. 36, 567-574. Aminoglycoside antibiotics paromomycin and streptomycin bind to decoding centre and induce errors in translation: Binding of a correct (cognate) AA-tRNA normally induces conformational change in 30S subunit, to closed state, activating hydrolysis of EFTuGTP In the presence of paromomycin, even ‘near-cognate’ AA-tRNAs can induce this conformational change, so that mistakes occur more easily. Action of antibiotics A cross-kingdom antibiotic Puromycin - is an analogue of tyrosyl aminoacyl tRNAtyr and causes premature peptide chain termination - also inhibits protein synthesis in eukaryotes Can use puromycin analogues as tags to assay protein synthesis OP-Puromycin-based labelling of newly-synthesized proteins NH2 aa aa aa aa aa aa aa Imaging protein synthesis in vi Nascent polypeptide Puro- mycin 5’ E P A CH O-propargyl- mRNA Puromycin (OP-P) Ribosome Click Chemistry Puro- NH2 aa aa aa aa aa aa aa mycin CH O-propargyl- Puromycin (OP-P) OP-P Keratin Newly-synthesized proteins in DAPI mouse hair follicles Modified from Liu et al. PNAS 201 Liakath-Ali et al. Nature 2018 Don’t forget different Overall comparison Compartmentalise d in eukaryotes subunit sizes Melnikov et al (2012) Nature Struc Mol Biol 19: 560–567 What do we need for protein synthesis? A template that has sequence (clue/code) on where to start decoding A supply of building blocks Something to assemble the building blocks into chains A way to supply the correct building block at the appropriate time Rules to define HOW to decode (including where to start and stop) Energy to drive the process What do we need for protein synthesis? Ribosomes are the synthetic machinery (rRNA and proteins) The template is a mature mRNA (capped and tailed in eukaryotes) All 20 amino acids are activated as aminoacyl-tRNAs Requires a large number of other enzymes/proteins (factors) and energy in the form of GTP and ATP Adding each amino acid ‘costs’ 4x ATP Summar y Ribosomes are complex molecular machines, with rRNA forming important structural parts e.g. a peptidyl transferase centre PTC facilitates peptide bond formation between two charged tRNAs Translation elongation and termination events in prokaryotes and eukaryotes are similar Charging of tRNAs with correct amino acid ensures high fidelity Fidelity of protein synthesis is also ensured by elongation factors which check mRNA:tRNA codon:anticodon interaction Next lecture: what happens when silent or nonsense mutations occur in the open reading frame? Protein synthesis – a high-fidelity process Brandman and Hegde, Nature Structural and Molecular Biology, 2016 mRNA surveillance mRNA surveillance is a cellular quality control mechanism that detects and degrades defective mRNA molecules to ensure the fidelity of gene expression. Types of mRNA surveillance mechanisms Nonsense-Mediated Decay (NMD): Targets mRNAs with premature stop codons to prevent the production of truncated and potentially harmful proteins. Nonstop Decay (NSD): Degrades mRNAs that lack a proper stop codon, which can result in stalled ribosomes. No-Go Decay (NGD): Resolves stalled ribosomes on mRNAs due to secondary structures, rare codons, or damage. Nonsense-Mediated Decay (NMD) A surveillance mechanism to detect and destroy aberrant mRNAs containing Premature Termination Codons (PTCs) BEFORE translation Prevents accumulation of proteins with C-terminal truncations, which could create inactive or even dominant negative versions In vertebrate cells this mechanism is related to splicing Splicing and the Exon Junction Complex (EJC) AAAAAAAAAAA Splicing Proteins/factors recruited to exon junctions AUG UAA AAAAAAAAAAA Protein complex known as the exon junction complex (EJC) is deposited during splicing. It is placed 20-24 nucleotides upstream of each splice site. EJC contains various proteins, most important is UPF3 During export UPF2 binds to UPF3 in EJC Translation of normal mRNA AAAAAAAAAAA Splicing Proteins/factors recruited to exon junctions AUG UAA AAAAAAAAAAA Normal mRNA First ribosome recruited AUG UAA AAAAAAAAAAA Translation of normal mRNA AAAAAAAAAAA Splicing Proteins/factors recruited to exon junctions AUG UAA AAAAAAAAAAA Normal mRNA First ribosome recruited AUG UAA AAAAAAAAAAA Pioneer round of translation EJC is swept off by first ribosome AUG UAA AAAAAAAAAAA Translation of mRNA containing PTC (nonsense mRNA) AAAAAAAAAAA Splicing Proteins/factors recruited to exon junctions AUG UAA AAAAAAAAAAA NONSENSE mRNA AUG UAA UAA AAAAAAAAAAA First ribosome recruited What is “nonsense”? Stop codons more than 50-55 nucleotides upstream from most 3’ exon junctions are recognised as premature. Translation of mRNA containing PTC (nonsense mRNA) AAAAAAAAAAA Splicing Proteins/factors recruited to exon junctions AUG UAA UAA AAAAAAAAAAA NONSENSE mRNA First ribosome recruited AUG UAA UAA AAAAAAAAAAA Pioneer round of translation Ribosome does not reach EJC Downstream of PTC AUG UAA UAA AAAAAAAAAAA Fate of mRNAs containing a PTC AUG UAA UAA AAAAAAAAAAA Recruitment of SURF complex UAA UAA AAAAAAAAAAA SURF complex: SMG1 (kinase) UPF1 (RNA and ATP-dependent helicase) eRFs (translation termination factors) UPF1 interacts with UPF2, bridging SURF complex to EJC Fate of mRNAs containing a PTC AUG UAA UAA AAAAAAAAAAA Recruitment of SURF complex UAA UAA AAAAAAAAAAA SURF + EJC = DECID complex - causes aberrant termination Following SURF binding, SMG1 phosphorylates UPF1 and causes dissociation of eukaryotic Release Factors and ribosome. Fate of mRNAs containing a PTC AUG UAA UAA AAAAAAAAAAA Recruitment of SURF complex UAA UAA AAAAAAAAAAA SURF + EJC = DECID complex - causes aberrant termination Rapid degradation by multiple decay pathways Phosphorylated UPF1 recruits SMG7 and decay enzymes Fate of mRNAs containing a PTC AUG UAA UAA AAAAAAAAAAA Recruitment of SURF complex UAA UAA AAAAAAAAAAA SURF + EJC = DECID complex - causes aberrant termination Rapid degradation by multiple decay pathways AUG UAA UAA AAAAAAAAAAA Endonuclease Decapping and attack near the PTC Deadenylation and 5’-3’ decay 3’-5’ decay Prevalence of PTCs in human disease Diseases associated with presence of PTCs include: Duchenne muscular dystrophy (DMD) Familial adenomatous polyposis Hereditary breast and ovarian cancer Polycystic kidney disease Cystic Fibrosis PTCs may account for 90% of identified mutations in these diseases! Ribosomes can read through the PTC in the presence of Gentamicin Is therapeutic intervention possible with similar aminoglycosides? PTC124 (Translaren/Ataluren) is available for DMD treatment in the UK, but CF trials failed, even though they showed promise in mice with G542X mutation of the CFTR Non-Stop Decay A ribosome-associated quality control mechanism that targets mRNAs lacking a proper stop codon, preventing stalled ribosomes from blocking the translation machinery. Trigger: Occurs when mRNAs are improperly processed, resulting in transcripts that lack a termination codon. This defect causes ribosomes to translate into the 3′ untranslated region (UTR) and stall at the end of the transcript. Mechanism: Ribosomes stall at the 3′ end of the nonstop mRNA. A specialized surveillance complex recognizes the stalled ribosome. The Ski complex (Ski2, Ski3, Ski8) and the RNA exosome are recruited to degrade the defective mRNA. Dom34 (Pelota) and Hbs1 facilitate the dissociation of stalled ribosomes and release of the defective transcript. No Go Decay (NGD) mRNA surveillance mechanism that resolves ribosome stalling during translation. It targets defective mRNAs with translation-blocking features (e.g., strong secondary structures, rare codons, or damaged RNA) for degradation. Trigger: Occurs when a ribosome stalls irreversibly on an mRNA, preventing translation elongation or completion. Mechanism: Endonucleolytic Cleavage: Dom34 (Pelota) and Hbs1 are recruited to the stalled ribosome. Endonuclease activity cleaves the defective mRNA near the stall site. mRNA Degradation: The upstream mRNA fragment is degraded by the 5′ to 3′ exonuclease Xrn1. The downstream fragment is processed by the RNA exosome complex. Ribosome Recycling: Dom34 and Hbs1 also aid in releasing and recycling stalled ribosomes. Dom34 (Pelota) rescues stalled-ribosomes for recycling and known to regulate cell cycle in yeast = Pelota Doma & Parker, Nature 2006 Guydosh & Green, Cell 2014 Pelota deletion affects skin function Skin cross section Epidermal hyperplasia Liakath-Ali et al. Nature 2018 Liakath-Ali et al. Nature 2018 Amino Amino Rare codons Triplet TTT acid F Fraction Triplet 0.45 TCT acid S Fraction 0.18 TTC F 0.55 TCC S 0.22 TTA L 0.07 TCA S 0.15 In humans Leu is 6x more likely to be coded by a CUG TTG L 0.13 TCG S 0.06 TAT Y 0.43 TGT C 0.45 than a UUA TAC Y 0.57 TGC C 0.55 TAA * 0.28 TGA * 0.52 TAG * 0.2 TGG W 1 CTT L 0.13 CCT P 0.28 Useful to stop ribosomes accelerating too quickly at CTC L 0.2 CCC P 0.33 CTA L 0.07 CCA P 0.27 start of ORF CTG L 0.41 CCG P 0.11 CAT H 0.41 CGT R 0.08 CAC H 0.59 CGC R 0.19 Help to give enough time for the protein to fold CAA CAG Q Q 0.25 0.75 CGA CGG R R 0.11 0.21 ATT I 0.36 ACT T 0.24 ATC I 0.48 ACC T 0.36 May be helpful in regulating protein expression ATA ATG M I 0.16 1 ACA ACG T T 0.28 0.12 AAT N 0.46 AGT S 0.15 AAC N 0.54 AGC S 0.24 K 0.42 R 0.2 E.g. in ZEB2 translation of Leu(UUA)-Gly(GGU)- AAA AAG K 0.58 AGA AGG R 0.2 Val(GUA) causes ribosomal pausing and limits protein GTT V 0.18 GCT A 0.26 GTC V 0.24 GCC A 0.4 production but changing the codons to the common GTA V 0.11 GCA A 0.23 GTG V 0.47 GCG A 0.11 ones does not GAT D 0.46 GGT G 0.16 GAC D 0.54 GGC G 0.34 GAA E 0.42 GGA G 0.25 BUT pausing can cause no go decay GAG E 0.58 GGG G 0.25 Summary Comparing the three surveillance mechanisms, all can lead to destruction of an aberrant mRNA This is an important quality control mechanism E.g. with No-Go decay, if the collided ribosomes do not dissociate, the reading frame can shift to the +1 frame and then make an aberrant protein Powers et al (2020) Curr Opin Struct Biol 65:110-118 Recap: Overview of translational control steps in eukaryotes Protein synthesis in eukaryotes - Initiation 48S pre-initiation complex (PIC) formation and mRNA circularisation Binds poly(A) tail PABP Scaffold for AA AAA complex AAA Provides first tRNA Met assembly 4B 2 GTP 1 1A eIF4G 4E 4B mRNA 4A 3 40S AUG m7G cap-binding Mnk Similarities to Unwinds RNA Bridges scaffold to prokaryotes: structures eIF2 bound to GTP and ribosome brings in first tRNA Key differences: eIF3 and eIF1 ensure mRNA is circularised accuracy of initiation and Complexity adds several opportunities for prevent association of 60S control large subunit Control of eIF4E availability Rapamycin mTORC1 Primed PP P P P P 4E-BP1 4E-BP1 4E-BP1 4E 4E 4E 4E-BPs contain a similar sequence motif 4E to eIF4E binding site of eIF4G Hyperphosphorylation of 4E-BP disrupts interaction with eIF4E eIF4G Key control point: Without the eIF4E:eIF4G interaction, how can the ribosome attach to the 56 Control of eIF2 availability 48S pre-initiation complex (PIC) formation and mRNA circularisation PABP AA AAA AAA Provides first tRNA Met 4B 2 GTP Coupled to GTP 1 1A eIF4G 4E 4B mRNA 4A 3 40S AUG Mnk Key control point: Without the first tRNA, how do you start synthesising the polypeptide? Function and control of eIF2 Met g eIF2 has three Ternary complex subunits Met- b gamma binds tRNAi Met GTP 2 GTP eIF2 GT P GDP finds and guanine binds 40S recognise nucleotide eIF2B subunit s exchange Pi AUG start GTP codon eIF2 GD Translation P Initiation Release of factors GTP hydrolysis Function and control of eIF2 eIF2 has three Met g subunits Ternary complex P gamma binds Met- b tRNAi Met GTP phosphorylated 2 GTP eIF2 GT on Ser51 of P alpha subunit GDP eIF2B Pi GTP eIF2 GD Translation P Initiation PERK, PKR, Release of GCN2, HRI factors P Activation of PKR in response to viral RNA Virus-infected cells produce Interferon (IFN) This induces PKR (protein kinase R) expression in neighbouring cells (‘anti- IFN viral state’) dsRNA Inhibitory domain Based on Hinnebusch (2005) Nature Structural & Molecular Biology 12, 835 - 838 This causes a reduction in protein synthesis of both host and viral mRNA, which should prevent further infection Control of eIF4E availability Rapamycin mTORC1 Primed PP P P P P 4E-BP1 4E-BP1 4E-BP1 4E 4E 4E 4E-BPs contain a similar sequence motif 4E to eIF4E binding site of eIF4G Hyperphosphorylation of 4E-BP disrupts interaction with eIF4E eIF4G Key control point: Without the eIF4E:eIF4G interaction, how can the ribosome attach to the 61 Translational Control – mRNA Specific Mechanisms Secondary and tertiary structures formed by base-pairing of single stranded RNA – depends on length and G-C content Internal Ribosome Entry Sites (IRESs) May need non-canonical factors (ITAFs) Met Internal Ribosome Entry Site (IRES) 2 GTP 40S 4A 3 AUG Mnk1 eIF4G Ct 62 16S and 18S rRNA as a phylogenetic markers rRNAs have conserved as well as hypervariable regions Hypervariable regions are prone to mutations Genetic analyses of 16S or 18S rRNA are used for the identification and classification of new species Woese et al., 1990 PNAS Hypervariable regions differ among organisms. Sequencing of these regions provides taxonomic information about the organism and allows to build phylogenetic trees Summar y Accurate translation of genetic information is crucial in all living organisms mRNA surveillance acts as a final quality control step to ensure only a minimal amount of protein is translated from aberrant mRNAs All mechanisms result in mRNA decay Nonsense mediated Non-stop No go Further Resource Protein synthesis https://www.ibiology.org/biochemistry/protein-synthesis/