DNA Replication Lectures 3-4 BIOL2010 PDF
Document Details
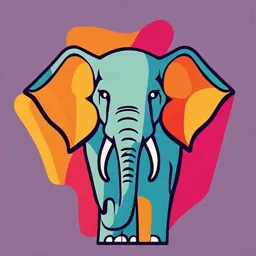
Uploaded by JoyousHawkSEye599
Southampton General Hospital
Dr M.L.Bellamy
Tags
Related
- Lecture 2.1 Molecular and Cellular Biology (DNA Replication) PDF
- Molecular Biology Lv2 DNA Replication Lecture 4 PDF
- "DNA Replication" - AP Biology Past Paper PDF
- Fundamentals in Biology 1: From Molecules to the Biochemistry of Cells PDF
- General Biology 2 BZE: DNA Replication Class Notes PDF
- LF130 Cellular and Molecular Biology Lecture 9, 2024 PDF
Summary
These lecture notes cover DNA replication, focusing on processes in E. coli. It examines the composition and structure of DNA, compares DNA synthesis and replication, explores problems unique to E. coli replication, and further investigates how eukaryotic and viral DNA replication differs. Includes visuals.
Full Transcript
BIOL2010 DNA replication Lectures 3-4 Dr M.L.Bellamy Overview 1) Composition/structure of DNA 2) DNA synthesis vs DNA replication 3) Problems and solutions in E.coli 4) The 3 steps in replication 5) Differences in eukaryotes/viruses DNA synthesis...
BIOL2010 DNA replication Lectures 3-4 Dr M.L.Bellamy Overview 1) Composition/structure of DNA 2) DNA synthesis vs DNA replication 3) Problems and solutions in E.coli 4) The 3 steps in replication 5) Differences in eukaryotes/viruses DNA synthesis PCR Only 1 enzyme required: Taq (DNA Polymerase) + dNTPs (ATP,CTP,GTP,TTP), Template DNA, primers Heat to 95°C (Denaturing) Cool to 55°C (Annealing) Heat to 72°C (Extending) DNA synthesis Escherichia coli Only 1 enzyme required: DNA polymerase III + dNTPs (ATP,CTP,GTP,TTP), Template DNA, primers But to replicate the entire chromosome: + Helicase + Topoisomerases + Primase + SSB proteins + DNA Polymerase I + DNA ligase +… DNA synthesis Escherichia coli Bacterial cells contain multiple DNA polymerases Named in order of discovery, not importance Pol I: DNA repair and replication Pol II: DNA repair Pol III: Principal DNA replication enzyme Pol IV: DNA repair Pol V: DNA repair All synthesize DNA 5’→3’ DNA synthesis Escherichia coli Bacterial cells contain multiple DNA polymerases Comparison of Pol I and Pol III: Pol I: one gene,109 kDa, ~400 copies per cell ~10 nt/s, 20-100 nt at a time → Too slow (>100 hours per genome) Pol III: 22 genes,106 kDa,~10 copies per cell ~1600 nt/s, >50,000 nt at a time → 40 minutes per genome (4.6 x 106 nt) DNA replication E.coli How can we determine which genes (proteins) are important? Simple knock-outs will be lethal Temperature-sensitive mutants allow proteins to be switched on or off by changing the temperature e.g. protein works at 20°C, but not 37°C Allow cells to begin replication, then deactivate one protein to see the effect 1) Quick stop mutants: Replication immediately stops 2) Slow stop mutants: Current round of replication finishes, but a new one can’t start DNA replication E.coli Gene Function dnaA Initiation at oriC dnaG primase (primer synthesis) dnaI Initiation at oriC dnaL DNA ligase dnaN Pol III β subunit (sliding clamp) dnaQ Pol III ε subunit (proofreading) dnaS UTPase dnaX Pol III holoenzyme γ subunit (binds ATP) dnaZ Pol III τ subunits (dimerization) holA Pol III δ subunit holB Pol III (δ’ subunit) holC Pol III (χ subunit) holD Pol III (ψ subunit) holE Pol III (θ subunit) gyrA DNA gyrase α subunit gyrB DNA gyrase β subunit ssb single strand DNA binding protein polA Pol I polB Pol II lig DNA ligase rep ATP-dependent helicase DNA replication Overview 1. DNA synthesis is simple 2. DNA replication is complex Overview 1) Composition/structure of DNA 2) DNA synthesis vs DNA replication 3) Problems and solutions in E.coli 4) The 3 steps in replication 5) Differences in eukaryotes/viruses DNA replication Problems 1. Strands being coiled (topology) 2. Circular DNA molecules (topology) 3. Antiparallel strands (polarity & topology) 4. Mutations/errors (fidelity) Need solutions that allow accurate synthesis, without slowing down the overall process too much. DNA replication Problem 1 Strands being coiled (topology) 5’ 3’ DNA strands are plectonemically coiled → have to be unwound to separate them DNA replication Problem 1 "Since the two chains in our model are intertwined, it is essential for them to untwist if they are to separate…. Although it is difficult at the moment to see how these processes occur without everything getting tangled, we do not feel that this objection will be insuperable." DNA replication Problem 1 Helicases can separate and unwind the duplex using ATP hydrolysis (3 bp/ATP) Hexamer ring surrounds a single DNA strand. Conformational changes in the helicase pull on the DNA strand, separating it from its partner. DNA replication Problem 1 Helicases move towards the 3’ end of the strand they are clamped to. One on each strand. 5’ 3’ 3’ 5’ Pol III can synthesize ~1600 nt/s There are ~10 nt per turn → Helicases must rotate DNA at ~10,000rpm! DNA replication Problem 1 Helicases separate and unwind the two DNA strands, creating a replication bubble. REPLICATION FORKS Uncoiling at one part of the duplex, creates mechanical strain in the rest of the molecule DNA replication Problems 1) Strands being coiled (topology) 2) Circular chromosomes (topology) Unwind part of duplex Torsional strain elsewhere in duplex Supercoiling DNA replication Problem 2 Supercoiling Relaxed Supercoiled DNA replication Problem 2 Supercoiling DNA topology can be described by the equation: Lk = T + W Lk = Linking number (fixed value in circular DNA) T = Twist – number of duplex turns (↑ with more nt) W = Writhe – number of duplex self-crossings Twist = N/h (Number of bp/helical repeat) e.g. pBR322 plasmid → 4361/10.5 = 415 e.g. E.coli genome → 4.6 x 106/10.5 = 440,000 N can’t change, but h can (over- or under-winding) DNA replication Problem 2 Supercoiling DNA topology can be described by the equation: Lk = T + W Lk = Linking number (fixed value in circular DNA) T = Twist – number of duplex turns (↑ with more nt) W = Writhe – number of duplex self-crossings The relaxed form, where W=0, is called Lk0 If Lk > Lk0 then there is +ve supercoiling (+W) If Lk < Lk0 then there is –ve supercoiling (-W) DNA replication Problem 2 Supercoiling W=2 RELAXED W=-2 W=0 POSITIVE (LEFT) NEGATIVE (RIGHT) DNA is overwound DNA is underwound DNA replication Problem 2 Supercoiling 1 2 525 bp Negatively supercoiled 3 N h →(525/11.4) 4 (525/10.5) DNA replication Problem 2 Supercoiling Superhelical density has the equation: σ = (Lk-Lk0)/Lk0 = ΔLk/Lk0 This is a normalised way of expressing how supercoiled a piece of DNA is, removing the effect of chain length. In relaxed DNA, σ = 0 Sign indicates type of supercoiling, as before DNA replication Supercoiling (-ve) Biological significance Purified cellular DNA is negatively supercoiled σ = -0.06 (eukaryotes and prokaryotes) Conversion of –ve Writhe to less Twist aids unwinding for transcription and replication Unwound ↑h -ve W DNA replication Supercoiling (-ve) Eukaryotic DNA is –vely supercoiled around histones when forming a nucleosome DNA replication Problem 2 Supercoiling (+ve) Biological significance Helicase-based unwinding→overwinding elsewhere Overwinding will resist replication fork movement Lk can’t change to relieve the stress without breaking the phosphodiester bond → Positive supercoils form Also applies to very long linear DNA Local overwinding DNA replication Problem 2 Supercoiling (+ve) Biological significance ↑h Unwound Relaxed Overwound ↓h +vely supercoiled ↑W +ve supercoiling needs to be removed for replication to continue. DNA replication Problem 2 Supercoil removal Specific enzymes that act on supercoils were first discovered by Wang and Gellert (1970s). Topoisomerases cause a change in Lk. Type I Cleave backbone of one strand Type II Cleave backbone of both strands → DNA then twists or untwists to get back to h=10.5 → DNA backbone is resealed after manipulation DNA replication Problem 2 Supercoil removal LK ↑↓ by 1 Topoisomerase type I mechanism 1) Phosphodiester bond is transferred to Tyr residue on enzyme, breaking one DNA strand 2) The other strand passes through the gap 3) The phosphodiester bond is transferred back to DNA, reforming the backbone on the other side → DNA moves one step towards the relaxed form DNA replication Problem 2 Supercoil removal LK ↑↓ by 2 Topoisomerase type II mechanism (ATP-dependent) 1) Both strands cleaved 2) A section of duplex is passed through the gap 3) Gap resealed Duplex may be from the same DNA molecule, or another DNA replication Problem 2 Supercoil removal LK ↓ by 2 Topoisomerase type II mechanism (ATP-dependent) Bacteria have a unique type II topoisomerase DNA gyrase Can introduce a –ve supercoil, usually by converting a +ve supercoil → Vital for replication fork progression in replication → A good antibiotic target DNA replication Problem 2 Supercoil removal LK ↓ by 2 Topoisomerase type II mechanism DNA gyrase Horizontal section cut. W 5’-P linked to Tyr on A subunit Reform backbone Vertical section W passed through DNA topology Supercoiling Supercoiled DNA runs faster than linear DNA in an agarose gel Relaxed/nicked DNA replication Problem 2 Bidirectional synthesis Theta replication Side view (Greek letter θ) DNA replication Problem 2 Catenanes When circular DNA molecules are replicated, the two daughter rings are interlocked and form a catenane Topoisomerase IV (Type II) Cleave, pass through and re-seal (decatenation) DNA replication Problem 3 Antiparallel strands Best solution: Two polymerases, one for each direction 5 3 ’ ’ 3 5 5 ’ ’ ’ 5 3 3 ’ ’ ’ 3 5 ’ genes needed → Extra ’ → Mutation rate ↑100x DNA replication Problem 3 All DNA polymerases synthesize DNA by adding to the 3’ end of an existing ds region (primer) 5 3’ ’ 3 3 5 ’ ’ ’ 5 3 3 ’ ’ ’ 3 5 ’ ’ Works, but requires complete strand separation before synthesis can start on upper strand → wasted time (separation time + synthesis time) DNA replication Problem 3 All DNA polymerases synthesize DNA by adding to the 3’ end of an existing ds region (primer) → Loop back the 5’→3’ (lagging) strand to correct direction 5’ 3’ Unwind start of duplex 5’ 5’ 3’ 5’ 5’ 5’ 3’ 3’ Synthesis of both strands can occur simultaneously, but semi-discontinuously → fragmented DNA DNA replication Consequences End result of semi-discontinuous replication is two, non-identical dsDNA molecules 5’ 3’ LEADING (1 primer) LAGGING (multiple primers) 5’ 3’ Okazaki fragment Chromosome is replicated, but lagging strand is unfinished: ‘Nicks’ in the new backbone, because Pol III can’t join fragments. Nick = broken phosphodiester bond. Not a gap. RNA is present in the DNA DNA replication Okazaki fragments Not completely DNA Primer is RNA RNA DNA ~10 bp ~2000 bp We know this because tiny fragments are left over after using DNAse on Okazaki fragments DNA replication Okazaki fragments Removal of RNA Okazaki fragments have RNA at their 5’ end and a nick at their 3’ end LAGGING STRAND(multiple primers) 5’ 3’ Pol I binds to nicks; Pol III does not Pol I can remove nt ahead of it; Pol III cannot DNA replication Okazaki fragments Removal of RNA Pol I binds to nicks 5’ 3’ RNA primers removed by Pol I 5’-3’ exonuclease Pol I detaches after ~100 bp →new nick left behind 3’ 5’ Pol I causes nick translation DNA replication Okazaki fragments Removal of RNA Alternatively: 3’ 5’ RNAse H degrades RNA Pol I fills in gap 3’ 5’ DNA replication Okazaki fragments Removal of nicks LAGGING 5’ 3’ DNA ligase seals DNA nicks (can’t do RNA-DNA) LAGGING DNA replication Okazaki fragments Okazaki investigated the synthesis of DNA over time using radioactivity E.coli culture infected with T4 bacteriophage Add 3H-TTP (tritiated thymidine) New phage DNA strands will be radioactive Alkaline lysis (pH13) Map out sizes of radioactive ssDNA over time DNA replication Okazaki fragments Okazaki investigated the synthesis of DNA over time using radioactivity 3H-TTP Incr eas incr ing eas time ing , size DNA replication Okazaki fragments Sizes mapped with density centrifugation ~2000bp Full length 2s 5s 10s 60s → Size of radioactive ssDNA doesn’t simply increase smoothly over time. Short pieces are always present. DNA replication Okazaki fragments Explanation: Short fragments are repeatedly being made on the lagging strand as new sections of DNA are unwound After a few seconds they are joined to each other and to non-radioactive pieces that had already been synthesized→size jumps DNA replication Okazaki fragments Evidence: 1) Pulse/chase experiments After 2s the 3H-TTP is removed and “chased” with normal ~2000bp TTP → Radioactivity all moves down Full length the tube 2s 60s DNA replication Okazaki fragments Evidence: P OH 2) DNA ligase mutants DNA ligase seals “nicks” in the backbone and can join Okazaki fragments to each other. ATP consumed. DNA replication Okazaki fragments Evidence: 2) DNA ligase mutants Short fragments still made, but they remain ~2000bp as fragments. → Lagging strand can’t be “finished” Full length 2s 60s