BIOL1406 Biology2e Ch12 Guide - PDF
Document Details
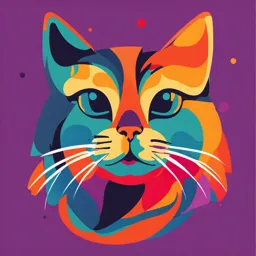
Uploaded by NeatNaïveArt
Tags
Related
Summary
This document provides an overview of Mendelian genetics, covering topics such as traits, alleles, genotypes, phenotypes, and inheritance patterns in diploid organisms. It's useful for understanding fundamental concepts in biology.
Full Transcript
CH. 12 – MENDEL’S EXPERIMENTS & HEREDITY GENES Units of information about specific traits Passed from parents to offspring on chromosomes (DNA) Each gene has a specific location (locus) on a chromosome...
CH. 12 – MENDEL’S EXPERIMENTS & HEREDITY GENES Units of information about specific traits Passed from parents to offspring on chromosomes (DNA) Each gene has a specific location (locus) on a chromosome ALLELES Different molecular forms of a gene (i.e. the exact sequence present) New alleles arise by mutation Different alleles of the same gene produce basically the same protein or RNA molecule during gene expression, but one or more amino acids may be changed, resulting in a different cellular function (either level of function or type of function). Example: A gene locus contains the information to produce an enzyme that degrades the amino acid phenylalanine. The most common allele at this gene locus produces a normal enzyme. If you have the PKU allele at this gene locus, that means your DNA has a mutation that causes it to produce a NON-functional enzyme. Individuals with only PKU alleles cannot degrade phenylalanine and suffer from the genetic disorder, PKU. This work is licensed under a Creative Commons Attribution-NonCommercial-ShareAlike 4.0 International License. SOME KEY TERMS FOR THIS CHAPTER Trait: a particular physical characteristic (like flower color, blood type, etc.) Types of Alleles o Wild-type allele: the most common allele in a population (so called “normal allele”) o Mutant allele: a rare allele in a population; reasoned to be the most recently formed allele by mutation (also called “non-normal allele”) o Dominant: allele that masks others, often designated with capital letters o Recessive: allele that is masked by others, often designated with lower case letters Zygote: first diploid cell produced by fertilization Cross: mating between individuals that produces offspring (or hypothetical mating to examine potential offspring) o Self-pollinate: In plants, a form of sexual reproduction where the male and female gametes originate from the same plant. o Cross-pollinate: In plants, when the male gametes of one plant are delivered to the female gametes of a different plant. Punnett Square: Chart used to determine POSSIBLE GENOTYPE AND PHENOTYPE Genotype: the specific combination of alleles carried by an individual that cause a particular phenotype o Genotypes are the allele symbols. In diploids: AA vs. Aa vs. aa o Deciding which letter to use – must be the same letter for all alleles at the same gene locus, and choice is often the most noticeable trait. Dominant alleles are written as capital letters Recessive alleles are written as lower case letters o Example: in pea plants, seed color is either yellow or green. Yellow is dominant to green, so alleles are written as Y (for yellow allele) and y (for green allele). Phenotype: the version of the trait displayed by an individual, determined by the genotype for that trait o Phenotypes are usually word descriptions (yellow vs. green), not symbols. o Because some alleles are dominant and others are recessive, two individuals can have different genotypes and still display the same phenotype. o NOTE: phenotypes are physical traits, but are NOT always directly ALLELE COMBINATIONS (GENOTYPES) HOMOZYGOUS when both alleles at the same gene locus are identical also described as “true-breeding” because they pass on the same alleles to all offspring Example: if a gene locus is designated by the letter A, both being the dominant “A” form, so genotype is AA, or both begin the recessive “a” form, so genotype is aa. HETEROZYGOUS having two different alleles at the same gene locus also described as a “hybrid” and are NOT true breeding When two different alleles are present in the same individual, one allele may be dominant over the other and mask (hide) it. The hidden allele is called recessive. o Example: if a gene locus is designated by the letter A, having one dominant “A” allele and one recessive “a” allele, so genotype is Aa. o In this condition, heterozygotes may be called carriers (because they carry, but don’t show a recessive allele) THE MYSTERY OF HEREDITY Heredity = transmission of traits from one generation to the next Genetics = the scientific study of heredity Very early on, we knew that sperm and eggs transmitted information about traits, but how? Blending theory was initially a popular explanation However: o With blending, we would expect variation to disappear Figure 1: Homunculus proposal o in reality, variation in traits on inheritance from a 1640 Swedish text. The illustration shows a “little man” pre- persists and some traits even formed. appear unexpectedly after disappearing for one or more generations MENDEL AND PEAS Gregor Johann Mendel is considered the father of genetics Chose peas as his organism to study inheritance because: o had many simple dichotomous traits (only 2 versions present - no blending - so always one or the other): flower color, seed shape, seed color, etc.) o many of these traits were true breeding o male and female reproductive structure were in one flower and easy to manipulate for specific crosses (easy to choose mating pairs) o Each pea was a new individual – could evaluate thousands of MENDEL’S EXPERIMENTAL METHOD Usually 3 stages 1. Obtain true-breeding strains for each trait he was studying – called these the Parental Generation (P) 2. Cross-fertilize true-breeding strains having alternate forms of a trait, producing hybrid offspring – called these the First Filial Generation (F1) 3. Allow the hybrid offspring to self-fertilize and examine offspring produced in this Second Filial Generation (F2) and for several generations continuing to self- fertilize after this (F3, F4, etc.). At each generation, count the number of offspring showing each form of the trait and record data for hundreds of offspring ONE-TRAIT CROSSES Cross to study variations of a single trait (looks at only one gene locus at a time) Starts with true-breeding pea strains for 7 different traits (Parental Generation) o Each trait had 2 variant phenotypes o Mendel performed reciprocal crosses (first where male has one version of the trait and female has the other, then vice versa for reciprocal) First cross is true-breeding to true-breeding Second generation cross turns out to be a monohybrid cross (cross between 2 organisms that are hybrids for this one trait). F1 GENERATION What Mendel Learned Offspring produced by crossing 2 true-breeding strains For every trait Mendel studied, all F1 plants resembled only 1 parent, and it was always the same phenotype that showed up in the F1 generation, no matter which parent had that phenotype (father vs. mother made no difference) o Referred to this trait as dominant o Alternative trait was recessive No plants with characteristics intermediate between the 2 parents were produced for any of Mendel’s chosen traits (remember: he chose them because there were no intermediate phenotypes in nature, so this isn’t surprising!) F2 GENERATION What Mendel Learned Offspring resulting from the self-fertilization of F1 plants Although hidden in the F1 generation, the recessive trait always reappeared among some F2 individuals o When is showed back up, the recessive phenotype was unchanged (not blended or modified) Counted proportions of traits - always found about 3:1 ratio in the F2 generation (offspring of two hybrids crossed) o This ratio suggested the notion of parents having 2 copies of every trait-determining factor (what we now know as alleles) – hybrids having one of each type so the recessive one is hidden, but can show up in offspring if they get the recessive from BOTH parents LOOKING CLOSER AT THE F2 GENERATION The 3:1 PHENOTYPE ratio Mendel found is actually caused by a genotype ratio of 1:2:1 (the “3” includes 2 types) F2 plant Phenotypes: ¾ plants with the dominant form ¼ plants with the recessive form The dominant to recessive ratio was 3:1 Mendel discovered the ratio is actually: 1 true-breeding dominant plant 2 not-true-breeding dominant plants (the F3, F4 and F5 generations showed that these are hybrids) 1 true-breeding recessive plant DEFINING MONOHYBRID CROSSES Parental Cross: true breeding purple crossed with true P breeding white PP, purple homozygote x pp, white homozygote F1 Monohybrid Cross: hybrid siblings crossed or hybrids F1 self-fertilize Pp, purple heterozygote x Pp, purple heterozygote All purple No blending! F2 Offspring: Mixture of purple plants and white plants, PP approximately Pp 3:1 Pp ratio.pp F2 MENDEL’S 5-ELEMENT MODEL (HYPOTHESIS) (with modern terminology and knowledge added) 1. Parents transmit discrete factors (genes) that determine traits. 2. For each characteristic, an organism inherits two copies of every gene, one from each parent. 3. Not all copies of a gene are identical. Alleles are alternative versions of genes that account for variations in inherited characters. The alleles an organism inherits can be the same or different. 4. Alleles remain discrete. The contents aren’t blended because we now know that each gene copy separately produces a product (protein or RNA) that functions in the organism. 5. The presence of an allele does not guarantee it will produce a “phenotype” in the organism. If the alleles of an inherited pair differ, then one determines the organism’s appearance and is called the dominant allele. The other has no noticeable effect on the organism’s appearance and is called the recessive allele. MENDEL’S FIRST LAW: LAW OF SEGREGATION Remember learning during meiosis that a gamete (sperm or egg) carries only one allele for each inherited characteristic because allele pairs separate (segregate) from each other during the production of gametes. This is what causes Mendel’s law of segregation. This means that the physical basis for allele segregation is the behavior of chromosomes during meiosis. Two alleles from 2 different gametes are joined at random, one from each parent, during fertilization. Note: Mendel had no knowledge of chromosomes or meiosis – the details of genes had not yet been discovered! Mendel used observations, experiments, and critical thinking to come up with this hypothesis that was later supported by discoveries about genes and meiosis that led to his model becoming theory. SETTING UP A PUNNETT SQUARE (ONE- TRAIT CROSSES) ent 1 genotype = AA …. all gametes get A ent 2 genotype = aa …. all gametes get a Possible gametes of parent 1: A A Possible gametes of a Aa Aa parent 2: Possible combinations a Aa Aa of traits in offspring (zygotes) Genotype ratio: 100% Aa (can also be written as 0:4:0, where the first zero means no AA offspring and the last zero means no aa offspring) Phenotype ratio: 100% Tall (can also be written as 4:0 where the 4 means 4/4 will be tall and the 0 means 0/4 will be short) PROBABILITY BASICS Chance events are described by probability Probability is given as a fraction or decimal equivalent over a range of zero to one (where 0 = 0% and 1 = 100%) A probability of 0 means an event will not happen and therefore is not due to chance at all. A probability of 1 means an event will happen – it is certain and therefore not due to chance at all Anything that is chance ranges between those values For example, the chance of a flipped coin landing with heads up is ½ ( or 0.5, or 50%) PROBABILITY VS. RANDOM OFFSPRING PROBABILITY: The chance that each outcome of a given event will occur is proportional to the number of ways that event can be reached. This is what you expect to see. ACTUALITY/REALITY: What you actually measure showing what actual offspring were produced each time a random egg and random sperm fused – Mendel counted hundreds of offspring for every cross to get a large enough sample size to be able to draw conclusions. What you actually see is usually similar to what you expect to see, but it depends on how many offspring you examine. Think of a family of 4 with all boys – you expect 50% boys, but don’t always see that! SOLVING PROBLEMS WITH PUNNETT SQUARES 1. Decide on symbols for each allele and create a KEY/LEGEND. 2. Write out the phenotypes and genotypes (if known) of parents 3. Determine the allele(s) present in each possible gamete for each parent 4. Create a Punnett square by placing the gamete genotypes across the top for one parent and down the left for the other parent 5. Simulate random matings between each type of gamete by filling the squares with offspring genotypes that would be formed if those gametes fused during fertilization. 6. List all different offspring genotypes you created & probability. 7. Use these genotypes to determine phenotypes & combine TEST CROSSES Reminder: An individual with a dominant phenotype could have either the homozygous OR heterozygous phenotype. A test cross allows you to determine an unknown genotype by observing offspring phenotypes and calculating backwards. Selecting the correct mating partner in a test cross is crucial. To do a test cross: An individual with dominant phenotype but unknown genotype is always crossed to an individual with recessive phenotype. Why? o To show a recessive phenotype means the individual must have the homozygous recessive genotype. o Therefore, phenotypic ratios among offspring will be different ONLY depending on the genotype of the unknown parent. This lets you see what alleles the unknown parent contributed. If BB is crossed with bb 100% of offspring would be dominant. If Bb is crossed with bb offspring would be a mix of dominant & HOW THE TEST CROSS WORKS Rationale: Determine whether an organism expressing a dominant trait is a homozygote or a heterozygote. Mendel verified these results for test crosses with numerous different traits in monohybrids. Each parent carries 2 alleles for each trait. Alleles segregate equally and randomly into gametes. Gametes randomly recombine alleles in zygotes during fertilization. HUMAN INHERITANCE DOMINANT AND RECESSIVE PATTERNS Some Dominant Traits Some Recessive Traits Achondroplasia Albinism Brachydactyly Cystic fibrosis Huntington’s disease Duchenne muscular dystrophy Marfan syndrome Galactosemia Neurofibromatosis Phenylketonuria Widow’s peak Sickle-cell anemia Familial hypercholesterolemia Tay-Sachs disease Most human traits listed “dominant” are not really simple dominant. One copy of the “dominant” allele produces the disease or trait shown in the table. The heterozygote shows a phenotype - this is why we call them dominant. But, two copies of the “dominant” allele produces a more severe HUMAN INHERITANCE ANALYSIS Most human traits are controlled by interactions between multiple gene loci, but some human traits are controlled by a single gene. o of those controlled by a single gene, some exhibit dominant / recessive inheritance (see previous slide), others exhibit more complicated inheritance patterns. In humans, we can’t control the # of offspring or mating choices, so determining inheritance of a trait often takes decades of information. A pedigree: o shows inheritance of a trait in a family through multiple generations, o demonstrates dominant or recessive inheritance o can also be used to deduce genotypes of family members o can be used in genetic counseling of potential parents who worry they are carriers of a disease-causing, recessive allele SYMBOLS FOR PEDIGREES Probability rules can be used in conjunction with pedigrees to make genetic inferences. RECESSIVE TRAITS IN PEDIGREES Individuals with the disorder are in blue and have the genotype aa. Unaffected individuals are in yellow and have the genotype AA or Aa. o It is often possible to determine a person’s genotype from the genotype of their offspring. For example, if neither parent has the disorder but their child does, they must be heterozygous. o Two individuals on the pedigree have an unaffected phenotype but unknown genotype. Because they do not have the disorder, they must have at least one normal allele, so their genotype gets the “A?” Recessive traits disappear and reappear. designation. Affected individuals may have unaffected parents. Because the trait is inherited through recessive allele, carriers (heterozygotes who are hiding their recessive allele) are DOMINANT TRAITS IN PEDIGREES Dominant traits can be more difficult to discern because there is no obvious rule as there is for recessive traits. In this same pedigree, notice the pattern of the individuals in yellow (the normal phenotype is dominant, so these individuals may have the genotype AA or Aa. Dominant traits appear in every generation or if they are missing in the parents, they are lost/absent in all generations that follow from those parents. Carriers cannot exist: if you have it, you show it. TWO-TRAIT CROSSES Crosses looking at inheritance of TWO TRAITS at the same time. The cross is still between two parents each with their own genotype, but now looking at two different genes under investigation. Example: If the TRAITS under investigation are flower color AND stem height: o So, we are comparing purple flowers (caused by the A allele) vs. white flowers (caused by the a allele) AND we are also comparing tall (caused by the B allele) vs. short (caused by the B allele) o A parental cross would be mating the opposite double homozygous parents: one double homozygous dominant (AABB) and one double homozygous recessive (aabb) o A dihybrid cross would be a mating between heterozygotes (hybrids) when looking at two traits at the same time (di). MENDEL’S SECOND LAW: LAW OF INDEPENDENT ASSORTMENT In a 2-trait cross (and in crosses looking at more than 2 traits at a time), the alleles of each gene locus assort independently The segregation of different allele pairs is independent Why? Independent alignment of different homologous chromosome pairs during metaphase I leads to the independent segregation of the different allele pairs WHAT YOU NEED TO KNOW ABOUT 2- TRAIT CROSSES Good news: I will not expect you to be able to work any 2-trait crosses or Punnetts on a test. Less-good news: I will expect you to be able to identify the gametes that an organism would produce when looking at 2 or even 3 traits (such as an organism with genotype AaBBcc) and to be able to explain why alleles at different gene loci assort independently and what meiosis, chromosomes, gene loci, etc. have to do with it. DETERMINING POSSIBLE GAMETES IN TWO- TRAITS WITH HETEROZYGOTES Heterozygous parent: AaBb Possible gametes:AB Ab aB ab A must segregate from a (homologous pairs, Mendel’s Law of Segregation) B must segregate from b (homologous pairs, Mendel’s Law of Segregation) Determining whether A vs. a goes into a gamete is independent of whether B vs. b goes into that same gamete. This is Mendel’s Law of Independent Assortment. MENDEL’S PARENTAL & DIHYBRID CROSSES Parental and F1 crosses starting with parents that were true breeding for opposite phenotypes of two different traits. The traits were seed shape, round (R) or wrinkled (r) and seed color, yellow (Y) or green (y). True- breeding parents were RRYY (round and yellow) and rryy (wrinkled and green). P generation (homozygotes) F1 dihybrids (double heterozygotes) F2 is a mixture of genotype and phenotype categories MENDEL’S SECOND LAW IN ACTION If you count for each trait individually you will see each still gives a 3:1 ratio as in monohybrid crosses. This led Mendel to conclude that traits were inherited independently from one another. Segregation and recombination of alleles for one trait has no effect on segregation and recombination of alleles for other traits. TREMENDOUS VARIATION Living organisms are the results of a LOT more than just two traits… The number of different genotypes an individual could inherit from two parents is enormous. Considering independent assortment, the number of possible genotypes an offspring could inherit from two heterozygote parents is 3n where n is the number of gene loci at which the parents differ EXTENSIONS TO MENDEL Mendel’s model of inheritance assumes that Each trait is controlled by a single gene Each gene has only 2 alleles There is a clear dominant-recessive relationship between the alleles (law of dominance applies) Most genes do NOT meet these criteria. POLYGENIC INHERITANCE Occurs when multiple genes are involved in controlling the phenotype of a trait The phenotype is an accumulation of contributions by multiple genes These traits show continuous variation and are referred to as quantitative traits o histogram shows normal distribution (not bimodal or A bar chart to represent variation in height discrete and separate traits) Image Credit: bbc.co.uk – © copyright 2019 BBC Direct link to image (text address: https://www.bbc.co.uk/bitesize/guides/z9gk87h/revision/3) o PLEIOTROPY Inheritance pattern caused by an allele which has more than one effect on the individual’s phenotype Pleiotropic effects are difficult to predict, because a gene that affects one trait often performs other, unknown functions This can be seen in human diseases such as cystic fibrosis or sickle cell anemia o multiple symptoms can be traced back to one defective allele MULTIPLE ALLELES Gene locus for which there are more than 2 alleles commonly found in a population, each causing a different phenotype Just based on the important function of the gene product, how many different variants can exist will be limited, but many gene loci have more than just 2 alleles possibilities. Regardless of the number of possible alleles, each individual can still only inherit 2 alleles (diploid) Example: ABO blood types in humans has 3 common alleles (see later slides) DOMINANCE RELATIONS Complete dominance o In Mendel’s peas, this was common, but in many organisms, complete dominance is less common or seen only when one allele is defective (causing a disorder in the homozygous recessive). Incomplete dominance o Heterozygote phenotype is somewhere between that of two homozygotes o Common when one copy of a functioning allele is not enough to produce the same phenotype as two copies Codominance o Non-identical alleles specify two phenotypes that are both expressed in heterozygotes INCOMPLETE DOMINANCE Flower Color in Snapdragons: Cross a true breeding red flower parent with a true breeding white flowered parent o Result: all pink F1 heterozygotes (no red flowers and no white flowers). o If you cross these pink F1 sibs you get an F2 generation with the following ratio of Thephenotypes: red pigment1 red: 2 pink: allele 1 white is NOT completely dominant over the no-pigment (white) allele, it is incompletely dominant. o We cannot use capital and lower case letter choices because neither phenotype dominates over the other – the heterozygote phenotype is in between. o So, in example, the red flower allele is designated CR – and the white flower allele is designated CW HOMEOTIC MUTANTS AFFECT DEVELOPMENT Homeotic mutants affect development – these are alleles at gene loci that regulate expression of other genes Mutant alleles can potentially affect many characters. Remember Mendel’s traits were As seen in comparing the caused by single genes that wild-type Drosophila (left) and the Antennapedia mutant affected only one trait. (right), the Antennapedia mutant has legs on its head in place of antennae. GENETICS OF ABO BLOOD TYPES: CODOMINANCE AND MULTIPLE ALLELES In codominance, two alleles cause a phenotype that is not blended, but rather shows both phenotypes at the same time Key Example: In humans, the gene that controls ABO blood type encodes an enzyme that controls the structure of a glycolipid produced & placed on the surface of red blood cells o Two known alleles at this gene locus (IA and IB) are codominant to one another If an individual has genotype IA IB (both codominant alleles), this person produces both glycolipids & is described as having the dual phenotype - blood type AB IA and IB are both written with a capital “I” to indicate they are dominant alleles. Superscript indicates the particular allele. o A third allele at this same gene locus (i) is recessive to A B ABO BLOOD TYPE: ALLELE COMBINATIONS Note: blood type is the phenotype here! Type A - IAIA or IAi Type B - IBIB or IBi Type AB - IAIB Type O - ii ABO AND TRANSFUSIONS When a person receives a blood transfusion, they are receiving blood cells from someone else. The recipient’s immune system will attack any blood cells with an unfamiliar antigen on their surface o A person who normally has type A blood, but receives blood from a type B person will mount a massive immune response against the blood cells carrying the B antigen. o Antibodies cause red blood cells to clump together, blocking blood flow. This kills the patient. Type O individuals are considered “universal donors” because their red blood cells carry neither type A nor type B antigens, so do not cause an immune response in recipients. o Type O blood can be given to individuals with type O, type A, type B, or type AB blood. EPISTASIS If the product of one gene directly affects expression or action of another gene product, this effect is called epistasis. o Common when a trait is determined by action of a metabolic pathway (controlled by series of enzymes). o Behavior of gene products changes expected ratios despite independent assortment of the actual alleles on the 2 gene loci Example: o Mottled agouti coat color (A) is dominant to solid coloration, such as black or gray. o A gene at a separate locus (C) is responsible for pigment production. o The recessive c allele does not produce pigment. o A mouse with homozygous recessive cc genotype is albino regardless of which alleles are present at the A locus. GENE LINKAGE Gene linkage = when two gene loci are so close together on a chromosome that they do not assort independently. o Affects inheritance because traits become linked in offspring. Crossing over reduces linkage, but if the genes are too close together, it is less likely crossing over will shuffle these alleles. Sex linkage = when a gene locus is on a sex chromosome (X or Y). o Affects inheritance because human males receive an X chromosome only from their mother (none from their father). Human females receive 2 X chromosomes, one from each parent. o Males only get one allele at these gene loci, so they ALWAYS show the phenotype of that allele = recessive is visible more often than in females because can’t be hidden behind the other copy (if dominant) o Males cannot inherit X-linked traits from their fathers because to be male, they have to get the Y chromosome from their father. o Females never inherit Y-linked traits at all because they do not receive a Y. Note: Your textbook goes into detail about how these traits are inherited – INHERITANCE OF SEX LINKED TRAITS The son of a woman who is a carrier of a recessive X-linked disorder will have a 50 percent chance of being affected. A daughter will not be affected, but she will have a 50 percent chance of being a carrier like her mother.